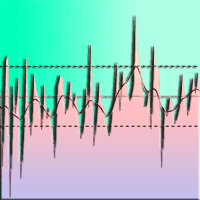
Response to climate and influence of ocean-atmosphere phenomena on annual radial increments of Pinus oocarpa Schiede ex Schltdl. & Cham in the Lagunas de Montebello National Park, Chiapas, Mexico
iForest - Biogeosciences and Forestry, Volume 16, Issue 3, Pages 174-181 (2023)
doi: https://doi.org/10.3832/ifor4112-016
Published: Jun 30, 2023 - Copyright © 2023 SISEF
Research Articles
Abstract
The Lagunas de Montebello National Park, Chiapas, Mexico, is one of the most important protected areas in terms of ecology and the provision of ecosystem services in the state of Chiapas; however, it lacks long-term climate information to support comprehensive plans for the conservation of endangered species and ecosystem restoration actions. The objectives of this work were to analyze: (i) the interannual variability of the annual rings of Pinus oocarpa Schiede in the Lagunas de Montebello National Park, as influenced by climate; and (ii) the ocean-atmosphere phenomena influence on both the interannual variability of the total ring-width series. A dendrochronological series of P. oocarpa was built for the period 1857-2018 (162 years), which indicates the presence of extreme hydroclimatic events in the region. Of these, the 1998 drought was a milestone for the management of this natural area due to the high-intensity fires that affected the area and caused unprecedented ecological, social, and economic damage in the site history. The climatic variables with the greatest influence on the annual radial increase of the species are precipitation and maximum temperature in winter-spring, when growth is positively associated with precipitation and negatively associated with temperature, attributed to increases in evapotranspiration. El Niño Southern Oscillation was the phenomenon with the highest correlation with climatic variability and the radial growth of the species, in frequencies of less than five years, although the positive influence of the Atlantic Multidecadal Oscillation was also assessed annually. Global warming, characterized by increasing temperature, threatens the persistence of plant communities in the study area; therefore, knowing its impact on the growth of species of economic importance is essential to support conservation actions.
Keywords
Dendrochronology, Hydroclimatic Variability, Drought, Pinus oocarpa
Introduction
Biocultural diversity in the Lagunas de Montebello National Park (PNLM, in Spanish) is essential to support its protection and conservation. This area is home to several plant species listed as priorities for conservation, including several orchids (Epidendrum corirfolium Lind., Maxillaria elatior Rchb. f.), and bromeliads (Tillandsia carlsoniae L.B.Sm., T. eizii Lyman B. Smith - [5]). Some of the tree species are included in the Red List of the International Union for Conservation of Nature (IUCN), such as Quercus crassifolia Humb. & Bonpl., Quercus sapotifolia Liebm., Quercus elliptica Née, Pinus oocarpa Schiede, and Pinus maximinoi H.E. Moore. Additionally, the PNLM has been designated as a RAMSAR site and integrated into the World Network of Biosphere Reserves due to the importance of its wetlands and lakes of karst origin, which are sites of unparalleled scenic beauty and valuable provision for ecosystem services to the region ([9], [31]).
The presence of P. oocarpa in the conifer forest with shallow soil and annual precipitation below 1200 mm characterizes this vegetation type in the PNLM ([9]). Although rainfall may not be a limiting factor for the development of this species, the karst condition of the site characterized by the dominance of shallow low-fertility soils contributes to both limited water and nutrient availability, thus affecting the annual radial growth and enhancing the interannual variability in the annual rings of P. oocarpa ([39]).
Despite long-lived trees have been removed for forest logging or eliminated by strong fires and pests that have occurred in the region ([32]), long-lived pine individuals are still found in hard-to-access forest patches of Chiapas, Mexico, and Guatemala. These specimens can be used to construct dendrochronological series covering a period up to 200 years or more, beyond the extent of the available climatic records. This is a viable alternative to produce a better understanding of hydroclimatic variability in the study area, and to determine the presence and frequency of extreme hydroclimatic events and their trends over time; this is key to supporting biodiversity management and conservation plans in this area.
Knowledge of the variability of seasonal climatic factors such as precipitation and temperature is essential both for developing management strategies for conservation and implementing mitigation actions in case of extreme hydroclimatic events ([46]). To this end, one of the resources used for assessing the impact of the future climate is the historical and current behavior of global circulation patterns. These simulate physical processes in the atmosphere and provide support to predictive climate models ([8]).
The information derived from tree rings is used to capture the variability in climate series and their historical frequency and trends, which are useful for determining the fluctuations of extreme hydroclimatic events and changes in climate behavior due to global warming ([46]). This approach can provide information to respond to the challenges of climate change and set technically based actions for the conservation and restoration of biodiversity in this and other regions of Mexico and the world ([43]). This place is potentially vulnerable to climate change and wildfire risk, whose effect may be greater in areas of higher biological diversity included in conservation projects ([28]).
The objectives of the present study are to analyze: (i) the interannual variability of the annual rings of P. oocarpa in the PNLM as influenced by climate; and (ii) the ocean-atmosphere phenomena influence on both the interannual variability of the total ring-width series.
Materials and methods
Description of the study area
Sites representative of the coniferous forest were located within and outside the PNLM (Fig. 1). The first site is “Antelá”, located at 16° 06′ 48.8″ N, 91° 42′ 47.9″ W at an elevation of 1556 m a.s.l., in the municipality of Trinitaria, Chiapas, Mexico, adjacent to the PNLM; the site is dominated by mixed forest vegetation composed of pine-oak-sweetgum. The second site is “Tziscao” (16° 04′ 31.1″ N, 91° 41′ 11.7″ W; 1547 m a.s.l.), located within the PNLM and covered by a sweetgum-pine-oak forest. The third site, “Park” (16° 07′ 45.9″ N, 91° 43′ 53.8″ W; 1467 m a.s.l.), comprises part of the municipalities of La Independencia and La Trinitaria, Chiapas; it is located within the core area of the PNLM and has dominant vegetation of pine-oak-sweetgum. The coniferous species present in the area include Pinus maximinoi, Pinus tecunumanii F. Schwerdtf. ex Eguiluz & Perry, Pinus teocote Schiede ex Schltdl. & Cham., Pinus devoniana Lindl., and Pinus oocarpa, the latter being the dominant conifer species in the area and, in general, in the region ([9]). The dominant oak species are Quercus benthamii A.DC, Quercus laurina Humb. & Bonpl., and Quercus candicans Née ([34], [33]). The prevailing soils are classified as Lithosols, Rendzinas, Vertisols, Acrisols, Fluvisols, and Gleysols ([21]). Lithosols and Rendzinas prevail across most of the park and are dominant in steep slopes and hills, with very shallow soils prone to erosion and low moisture retention. Rendzinas also occupy gentler sloping sites. These soil types are deeper, with good fertility, and allow an adequate rooting of trees ([45]).
Fig. 1 - Distribution of Pinus oocarpa sampling sites in the Lagunas de Montebello National Park, Chiapas, Mexico.
Climate data
The PNLM comprises an elevation range of 1500 to 1800 m.a.s.l. According to García ([17]), the climate is of type C(fm), humid temperate with rains throughout the year, with a mean monthly temperature of 22.6 °C and mean annual precipitation in the range of 1200 to 1400 mm. The period with the highest precipitation spans from June to September (summer season). There are no climatic records from meteorological stations located directly in the PNLM, so the climate variability was extrapolated from the “La Trinitaria” meteorological station (16° 07′ 04″ N, 92° 03′ 06″ W; 1540 m a.s.l. - Fig. 2), located 32 km away from the study site in straight line that, due to its proximity, altitude, and similarity in ecological conditions, is considered representative of the climatic conditions characterizing the PNLM.
Fig. 2 - Climogram of the “La Trinitaria” meteorological station, Chiapas. (PPT): precipitation (mm); (Max Temp): maximum temperature (°C); (Min Temp): minimum temperature (°C).
Climate data (1901-2013) from the ClimaNA database ([48]), and a database for the period 1950-2015 of climatic stations of the National Meteorological System available in a 0.1° x 0.1° grid. Missing data were estimated by interpolation based on the existing data and Thiessen polygons ([42]), seasonal precipitation data (1892-2016) from the North American Seasonal Precipitation Atlas (NASPA - [38]), and drought indices (1870-2012) obtained from the Mexican Drought Atlas (MXDA - [37]).
Sampling procedure
At each sampling site, several individuals of P. oocarpa were chosen by including the largest number of mature and long-lived specimens and approximately 10% of young individuals, distributed over a large part of the study basin and, at the same time, representative of the dominant climatic conditions of the PNLM. Tree-ring series for P. oocarpa have been previously developed in Mexico and Guatemala, where the formation of annual rings and their response to limiting climate conditions have been demonstrated ([23], [47]).
Although P. oocarpa forms annual rings, the period where the formation of annual rings is completed is not well understood. Results from a dendrochronological study done with this species in Eastern Guatemala indicates the activation of cambial activity with the first rains of April and May, earlywood formation may end with the mid-summer drought (July-August), be reactivated in September when the latewood formation starts forming and ending by November ([39]).
From each sampled tree, 2 to 3 increment cores were drilled at breast height (1.30 to 1.40 m) with a Pressler bore of variable dimensions (20.0 to 45.0 cm) and 5.1 mm inner diameter. Each individual was georeferenced and the presence of fire scars, wounds, incidence of pests, and some other ecological variables of the microsite were noted; these data are useful to explain growth anomalies, which could be evidenced during the dating of annual increments.
Overall, 102 trees were sampled, 43 in Antelá, 33 in the Park, and 26 in Tziscao, from which two increment cores per tree were obtained totaling 204 increments. Additionally, 187 cores were collected from 83 trees, which were used for an age structure analysis carried out for the same study sites. Also, 10 cross-sections obtained from dead individuals or from stumps found scattered across the study area were obtained, for a total of 401 samples from 195 trees for dendrochronological dating.
Tree-ring series development
Samples were dried at room temperature, then polished with sandpaper of different grains (coarse to fine) to delimit annual growth rings. After pre-dating, the ring widths were measured in a Velmex system to the nearest 0.001 mm. Dating quality was determined with the COFECHA program ([20]). To eliminate the influence of an increasing diameter, the measured series were standardized with the ARSTAN program by applying a double standardization procedure to the data: first, a negative exponential or regression line; second, a flexible curve (spline) comprising 128 years that preserves 50% of the variance ([12]). This process yielded three versions of the chronology, namely standard, residual, and arstan, each generated through different statistical processes ([12]). The residual chronology, in which the autocorrelation between ring-width series was eliminated by a robust mean, was used as a proxy of the interannual climate variability of the PNLM in the extension of the chronology, where the number of increment cores indicated statistical reliability for the purposes of climate reconstruction and determined by an Expressed Population Signal (EPS) value higher than 0.85 ([49]). Likewise, various dendrochronological parameters were selected to determine the potential of the chronology for dendroclimatic reconstructions, such as the intercorrelation between series, mean sensitivity, and first-order autocorrelation.
Climate response function and influence of large-scale circulation modes
To analyze the response of the total ring-width chronology to climate, we used a Pearson correlation approach by contrasting the tree-ring series with several climate databases, including weather records from the Trinitaria station. To assess the influence of large-scale ocean-atmosphere modes on the behavior of the total dendrochronological series, this was compared with El Niño Southern Oscillation (ENSO) indices, that is, the Multivariate ENSO Index (MEI), an indicator that combines oceanic and atmospheric variables to estimate an index of ENSO variability ([50]), and El Niño Ocean Index (ONI), a measure of the condition of El Niño-Southern Oscillation and its warm (El Niño) and cold (La Niña) phases in the Central Equatorial Pacific ([18]). Similarly, Pearson’s correlation values were obtained when comparing the ring-width chronology with indices of the Atlantic Multidecadal Oscillation (AMO - [14]), a quasi-periodic climate variation from 50 to 70 years centered on the Atlantic region, and the Pacific Decadal Oscillation (PDO - [26]), a recurrent pattern of ocean-atmosphere climate variability above 20° in the Pacific Ocean basin.
The total dendrochronological series, which constitutes an indirect source of climate variability, was plotted over the total period of time studied, with a mean close to unity (≈ 1.0), where values above (> 1.0) and below (< 1.0) the mean represent wet and dry conditions, respectively ([16]). In the ring-width chronology, extreme hydroclimatic events were those with a standard deviation below (extremely dry) or above the mean (extremely wet - [25]).
To assess the influence of general circulation modes, the presence of significant peaks in this series was determined by a power spectral analysis ([19]); the periods in which the dendrochronological series indicates the significant influence of these phenomena were determined by a wavelet spectral analysis performed in R ([41]).
Results
Dendrochronological series
Of a total of 401 increment cores, 157 (39% of the total samples) were suitable for dating, although the number of cores per year to estimate the ring-with indices varied along the extent of the chronology, with a sample size of 5 cores after 1890 (Fig. 3).
Fig. 3 - Residual version of the ring-width chronology with sample depth showing extreme hydroclimatic events (wet and dry) based on one standard deviation above and below the mean, respectively.
The dendrochronological series span over the period 1857 to 2018 (162 years), with a series intercorrelation of 0.399, which is higher than the minimum value required to be considered adequately dated (r = 0.328, p < 0.01). The mean sensitivity was 0.491, and the first-order autocorrelation was 0.4369, indicating that the growth of the current year is influenced by the growth of the previous year, which is common for the vast majority of conifers and other broadleaves in Mexico. The EPS value was higher than 0.85 from the early to the most recent part of the chronology. Therefore, the total series is considered statistically robust for climate reconstruction purposes and to analyze its influence on the radial growth of the species (Fig. 3).
Response of the chronology to climate variables
The relationship between total ring-width chronology and data from the regional precipitation grid, comprising the PNLM over the period 1953-2013 showed positive associations in April (r = 0.35, p < 0.01), May (r = 0.19, p < 0.05), and June (r = 0.17, p < 0.05) and the May-June cumulative period (r = 0.24, p < 0.05). A similar behavior was observed with shorter precipitation records (1992-2015; 24 years) from the La Trinitaria meteorological station, with a positive response in February (r = 0.43, p < 0.05), March (r = 0.17, p > 0.05), April (r = 0.18, p > 0.05), May (r = 0.15, p > 0.05), and seasonally for February-May (r = 0.32, p > 0.05).
Instrumental precipitation records for the 1980-2014 December-April season (35 years), obtained from NASPA ([38]) indicate a significant association with the total ring-width residual chronology (r = 0.43, p < 0.01). Precipitation data from ClimaNA showed no significant association in any of the 18 months in the comparison (6 months prior to the growth year and 12 months during the growing season). Based on these results, the period when the species shows a positive response to precipitation corresponds to the winter-spring season.
Regarding maximum temperature, the ClimaNA database (1901-2013) showed a negative association with the total ring-width chronology in November (r = -0.15, p > 0.05), December (r = -0.18, p < 0.05), January (r = -0.13, p > 0.05), February (r = -0.16, p > 0.05), March (r = -0.20, p < 0.05), April (r = -0.28, p < 0.01), May (r = -0.23, p < 0.05), and June (r = -0.21, p < 0.05), and seasonally for the mean maximum temperature in November-June (r = -0.29, p < 0.01).
In relation to La Trinitaria meteorological station, the maximum monthly temperature for 1992-2015 was negatively associated with the ring-width indices. Significant correlations occurred in June (r = -0.40, p < 0.05), July (r = -0.52, p < 0.01), and August (r = -0.56, p < 0.01) of the current growth year and the mean maximum temperature in June, July, and August (r = -0.53, p < 0.01). The associations between the total ring-width chronology and the precipitation and maximum temperature records for La Trinitaria meteorological station are shown in Fig. 4.
Fig. 4 - Correlation values between the dendrochronological series of total ring-width (residual version), monthly precipitation (Ppt in mm) and monthly mean maximum temperature (Tmax in ºC) records for La Trinitaria meteorological station.
Extreme hydroclimatic events in the dendrochronological series
The response of the chronology to climatic variables (precipitation, maximum temperature), supported that dendrochronological indices can be used as a proxy or indirect method of climatic conditions that have influenced the PNLM over the Past 162 years (1857-2018) and that extreme events evidenced as one standard deviation above or below the mean (1.0) correspond to wet and dry years, respectively. Based on the above, suitable climatic conditions occurred in years 1860, 1861, 1868, 1893, 1910, 1911, 1917, 1918, 1919, 1946, 1950, and 1996; whereas limiting climatic conditions occurred in 1859, 1862, 1866, 1870, 1889, 1890, 1894, 1899, 1900, 1917, 1920, 1955, and 1998; the latest (1998) was a milestone in the management of the PNLM because of devastating fires in the region (Fig. 5).
Fig. 5 - Prevailing rainfall conditions during 1998, which triggered extensive and severe fires across Mexico, especially in the state of Chiapas. Source: [38] (⇒ http://drought.memphis.edu/NASPA/).
Relationship between tree ring-width to large-scale ocean-atmosphere circulation modes
The association between the total ring-width chronology and various ENSO indices indicated a significant relationship (p < 0.05) for the MEI (1951-2017) in February (r = -0.25), March (r = -0.25), April (r = -0.24), and May (r = -0.27) of the current growth year (Fig. 6). A similar behavior was observed for the ONI index (1951-2018), with correlations in January (r = -0.20, p > 0.05), February (r = -0.23, p < 0.05), March (r = -0.23, p < 0.05), and April (r = -0.21, p > 0.05) of the current growth year.
Fig. 6 - Response of the ring-with chronology of P. oocarpa in the Lagunas de Montebello National Park and ENSO indices (MEI, ONI). Months with a lowercase letter (p) belong to the previous years of growth.
The smoothed AMO indices for the period 1920-2010 showed a significant association with the total ring-width indices (r = 0.21, p < 0.05), but only when considering the annual mean, suggesting that this phenomenon also influences tree growth in this region, although to a lesser extent relative to ENSO.
With regard to the association between PDO indices and total ring-width chronology indices in the period 1902-2012, we did not find any significant relationship (p< 0.05), which may indicate the lack of influence of this phenomenon on the radial growth of P. oocarpa in the PNLM.
The analysis of the spectral peaks in the regional dendrochronological series of the PNLM indicates significant frequencies in periods of 4.5, 3.69, and 2.18 years, respectively (Fig. 7).
Fig. 7 - Wavelet power analysis of total ring-width series residual version of P. oocarpa in the PNLM. The peaks framed by the red line are significant at 95%.
Discussion
The response of the ring-width chronology to climatic variables (precipitation, maximum temperature), supported that dendrochronological indices can be used as a proxy or method of predicting dominant climatic conditions that have characterized the PNLM over the past 162 years (1857-2018), and that extreme events evidenced as one standard deviation above or below the mean (1.0) correspond to wet and dry years, respectively.
The significant peaks detected in the ring-width chronology are located within the high frequency range of ENSO (5.0, 3.8, 2.5 years), a situation that confirms the significant relationship between total ring-width indices and MEI and ONI indices of this circulatory phenomenon. This behavior is verified through a wavelet analysis, which shows that periods when this phenomenon exerted a significant influence occurred throughout the chronology (Fig. 7).
The development of ring-width chronologies of P. oocarpa requires a large sample-size in comparison to other more climate-sensitive conifer species such as Pinus cembroides and Pseudotsuga menziesii characterized by interseries correlations > 0.7 ([10]). P. oocarpa showed frequent growth-ring releases and compression episodes, as well as false rings, which may be intrinsic to the species or associated with the seasonality of precipitation, forest density, and other human-induced disturbances ([40]).
Precipitation in winter-spring has a positive effect on the annual radial increment; however, rises in mean monthly maximum temperature in spring and summer along with higher water availability in tropical ecosystems exert an adverse effect on net primary productivity, attributed to an increase in evapotranspiration. This may result in greater vapor-pressure shortages, less growth, and ultimately in dieback and mortality due to the greater susceptibility of trees to pests and diseases ([1], [29]). On the other hand, higher temperatures imply a higher evapotranspiration rate and, therefore, lower biomass production ([35]).
The positive effect of cumulative seasonal precipitation in winter-spring and the negative effect of rises in temperature during the growing season has been reported for various species of conifers in temperate forests of north-central and southern Mexico ([2], [7], [27], [46]).
The relationship between the total ring-width series of P. oocarpa in the PNLM and precipitation and temperature data indicates that the chronology generated is a proxy of the prevailing climatic conditions in the area. Historical information on drought events at the national level indicates extreme dry periods in Chiapas from 1866 to 1870, 1887, and 1899 ([11]). Droughts recorded at national level, such as those occurred in the decades 1870, 1890, and 1950, also impacted the state of Chiapas, with adverse effects on food production ([15], [13]).
The most devastating drought in the state of Chiapas, particularly for the PNLM, occurred in 1998 due to fires that affected a large portion of the region ([9]). On the other hand, the extraordinary rainfall occurred at the PNLM in 1860, 1861, 1868, 1893, 1910, 1911, 1917, 1918, 1919, and 1946 are difficult to confirm with weather records other than dendroclimatic reconstructions ([38]).
A study of the variability of precipitation from the records of the Tonalá meteorological station, located on the coast of Chiapas, from 1961 to 2013, indicates wet years in 1963, 1968, 1973, 1974, 1980, 1981, 1988, 1989, 1995, 1998, 2005, 2006, 2007, and 2013 ([3]); these records generally coincide with above-average precipitation for the PNLM, but were not the most extreme events according to the dendrochronological series.
The general circulation modes that influence interannual climate variability in the PNLM indicate that El Niño is the climate phenomenon with the greatest impact in the region, as shown by the significant frequencies of 4.5, 3.69, and 2.18 found in the total ring-width chronology, corresponding to ENSO at high frequencies reported by Bruun et al. ([6]). In the southern part of Mexico, the effect of El Niño shows a contrasting behavior in terms of precipitation, compared to the northern part of the country. El Niño (warm phase) favors higher precipitation levels in winter-spring in northern Mexico, but dry conditions in the south of the country. This behavior is reversed under La Niña (cold phase -[30]), but is similar during intense La Niña events, producing droughts at a national level ([24]).
The effect of this phenomenon from the climatological perspective has been scarcely documented in this region. A study of the climatic records in the Zanateco river basin, Chiapas, indicates that the volume of rain recorded was related to an El Niño event, as greater or lesser precipitation is related to intense events of this phenomenon, causing variability in evaporation and temperature, although droughts and floods have occurred more frequently from 1997 onwards ([3]).
The influence of other atmospheric circulation models such as AMO and PDO on climate variability in southern Mexico has been little investigated ([36]). However, studies determining the influence of one of these phenomena, such as AMO, indicates a significant impact on the amount and distribution of precipitation, minimum and maximum temperatures, and their influence on food production in the coastal regions of Mexico, including Chiapas ([4]). A higher frequency of floods in Chiapas and Tabasco has been recorded during the positive phase of this phenomenon ([44]). In the present study, the total ring-width dendrochronological series of P. oocarpa showed a significant association with annual AMO indices, although the relationship was weak compared with the influence of ENSO. However, it has been determined that this phenomenon is a key driver of precipitation volumes in the Yucatan Peninsula ([22]). Therefore, the influence of this and other circulatory phenomena on the PNLM and other protected natural areas of Chiapas and the south of the country deserves more detailed analyses.
Conclusion
Pinus oocarpa is a dominant species in the conifer forest of the PNLM, and displays a suitable potential for dendroclimatic reconstructions, as indicated by the series intercorrelation value and average mean sensitivity found in the present work. This potential was confirmed by the significant association of the total ring-width series, residual version, and the local, regional, and reconstructed climate databases. The dendrochronological series spanned over 150 years and the total period covered was statistically reliable for climatic reconstruction purposes. It showed a significant response to the cumulative winter-spring precipitation and was negatively correlated with the mean maximum monthly temperature in summer; this suggests that temperature increases over this period may affect the growth of the species and its ecological stability in this conservation area of great ecological and social importance.
The dendrochronology series of P. oocarpa showed significant peaks in less than 7 years, corresponding to the frequencies of occurrence of the ENSO phenomenon. In fact, the warm phase of ENSO produced severe droughts, particularly during high-intensity events. The association with the AMO was weaker though significant, and no evidence of the influence of PDO was found.
The understanding and identification of the processes that affect the increasing damage caused by climate change will support the participatory work in the development of management strategies to protect the biodiversity of the PNLM.
Acknowledgments
The leading author acknowledges CONACYT and ECOSUR for funding this research as part of her Ecological and Sustainable Development doctorate studies. Part of the fieldwork and dendrochronological analysis were supported with funds of the project “Dendrochronological Mexican network: hydroclimatic and ecological applications”, FOINS” SEP-CONACYT CB 2016-1, No. 283134
Author Contributions
LPPC: conceived the study and carried out field measurements, samples curation, data processing, bibliographic investigation, methodology and wrote the original draft; JVD: conceived the study, carried out field measurements, made data curation, data processing validation, statistical analysis (dendrochronology), conceived methodology, provided resources, software, supervision, training, and writing review and editing, funding acquisition and wrote the original draft; DART: conceived the study and methodology, supervision and made writing review and editing; BAB: participated in bibliographic investigation, supervision and review, writing-review and editing; GCAG: conceived the study and provided supervision, and writing review and editing.
References
CrossRef | Gscholar
CrossRef | Gscholar
Gscholar
Gscholar
Online | Gscholar
Gscholar
Gscholar
Gscholar
Gscholar
Gscholar
Gscholar
Gscholar
Gscholar
CrossRef | Gscholar
CrossRef | Gscholar
CrossRef | Gscholar
Gscholar
Gscholar
CrossRef | Gscholar
CrossRef | Gscholar
Gscholar
Gscholar
Gscholar
Gscholar
CrossRef | Gscholar
Gscholar
Gscholar
Authors’ Info
Authors’ Affiliation
Guadalupe del Carmen Álvarez-Gordillo 0000-0002-9543-7920
El Colegio de la Frontera Sur, Departamento de Sociedad y Cultura, San Cristóbal de Las Casas, Chiapas (México)
Laboratorio de Dendrocronología, INIFAP CENID-RASPA, Gómez Palacio, Durango (México)
División de Ciencias Forestales, Universidad Autónoma Chapingo. Estado de México (México)
Departamento de Estudios Ambientales, Universidad Simón Bolívar, Caracas (Venezuela)
Corresponding author
Paper Info
Citation
Ponce-Calderón LP, Villanueva-Díaz J, Rodríguez-Trejo DA, Bilbao BA, Álvarez-Gordillo GC (2023). Response to climate and influence of ocean-atmosphere phenomena on annual radial increments of Pinus oocarpa Schiede ex Schltdl. & Cham in the Lagunas de Montebello National Park, Chiapas, Mexico. iForest 16: 174-181. - doi: 10.3832/ifor4112-016
Academic Editor
Angelo Rita
Paper history
Received: Apr 10, 2022
Accepted: Mar 21, 2023
First online: Jun 30, 2023
Publication Date: Jun 30, 2023
Publication Time: 3.37 months
Copyright Information
© SISEF - The Italian Society of Silviculture and Forest Ecology 2023
Open Access
This article is distributed under the terms of the Creative Commons Attribution-Non Commercial 4.0 International (https://creativecommons.org/licenses/by-nc/4.0/), which permits unrestricted use, distribution, and reproduction in any medium, provided you give appropriate credit to the original author(s) and the source, provide a link to the Creative Commons license, and indicate if changes were made.
Web Metrics
Breakdown by View Type
Article Usage
Total Article Views: 18268
(from publication date up to now)
Breakdown by View Type
HTML Page Views: 15206
Abstract Page Views: 1593
PDF Downloads: 1134
Citation/Reference Downloads: 3
XML Downloads: 332
Web Metrics
Days since publication: 747
Overall contacts: 18268
Avg. contacts per week: 171.19
Article Citations
Article citations are based on data periodically collected from the Clarivate Web of Science web site
(last update: Mar 2025)
(No citations were found up to date. Please come back later)
Publication Metrics
by Dimensions ©
Articles citing this article
List of the papers citing this article based on CrossRef Cited-by.
Related Contents
iForest Similar Articles
Short Communications
Climate effects on growth differ according to height and diameter along the stem in Pinus pinaster Ait.
vol. 11, pp. 237-242 (online: 12 March 2018)
Research Articles
The effect of provenance of historical timber on tree-ring based temperature reconstructions in the Western Central Alps
vol. 13, pp. 351-359 (online: 25 August 2020)
Research Articles
Influence of tree density on climate-growth relationships in a Pinus pinaster Ait. forest in the northern mountains of Sardinia (Italy)
vol. 8, pp. 456-463 (online: 19 October 2014)
Research Articles
Runoff reconstruction and climatic influence with tree rings, in the Mayo river basin, Sonora, Mexico
vol. 13, pp. 98-106 (online: 11 March 2020)
Research Articles
Growth-climate relations and the enhancement of drought signals in pedunculate oak (Quercus robur L.) tree-ring chronology in Eastern Hungary
vol. 11, pp. 267-274 (online: 29 March 2018)
Research Articles
Role of photosynthesis and stomatal conductance on the long-term rising of intrinsic water use efficiency in dominant trees in three old-growth forests in Bosnia-Herzegovina and Montenegro
vol. 14, pp. 53-60 (online: 28 January 2021)
Research Articles
Seedling emergence capacity and morphological traits are under strong genetic control in the resin tree Pinus oocarpa
vol. 17, pp. 245-251 (online: 16 August 2024)
Research Articles
Contrasting resin-tapped and untapped Pinus pinaster Ait. trees of central Spain in a dendroclimatic research
vol. 17, pp. 148-155 (online: 30 May 2024)
Research Articles
Historical fire ecology and its effect on vegetation dynamics of the Lagunas de Montebello National Park, Chiapas, México
vol. 14, pp. 548-559 (online: 01 December 2021)
Research Articles
The effects of fire on Pinus sylvestris L. as determined by dendroecological analysis (Sierra de Gredos, Spain)
vol. 15, pp. 171-178 (online: 09 May 2022)
iForest Database Search
Search By Author
Search By Keyword
Google Scholar Search
Citing Articles
Search By Author
Search By Keywords
PubMed Search
Search By Author
Search By Keyword