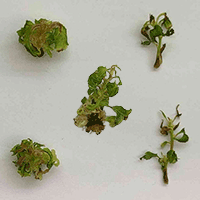
Variability of tolerance of Wild cherry clones to PEG-induced osmotic stress in vitro
iForest - Biogeosciences and Forestry, Volume 15, Issue 4, Pages 265-272 (2022)
doi: https://doi.org/10.3832/ifor4033-015
Published: Jul 25, 2022 - Copyright © 2022 SISEF
Research Articles
Abstract
The effects of drought simulated via osmotic stress induced by polyethylene glycol (PEG) in the growing medium were examined on two Wild cherry (Prunus avium L.) clones (6A and 8A) based on thirteen morphometric, physiological, and biochemical traits. The shoot tips were exposed to two PEG concentrations (20 and 50 g L-1) in growing medium designed for micropropagation with axillary buds. The results showed that all morphological and physiological traits were significantly reduced, indicating a strong detrimental effect of increased PEG concentrations. The significant decline of radical scavenging activity against ABTS•+ and total content of flavonoids (TFC) and phenols (TPC) were recorded in both clones as a response to high PEG concentrations, whereas opposite trends were noticed for ferric reducing antioxidant power (FRAP). Clone 8A achieved better performance, having more intensive growth, higher multiplication percentage, higher values of carotenoids, more intense decrement of ABTS and increment of FRAP values compared to the clone 6A. The results of the principal component analysis indicate that parameter TFC/TPC in both stem and leaves, as well as TFC in the stems, achieved the strongest relation with morphometric parameters. Our results confirm the feasibility of in vitro evaluation of drought tolerance of Wild cherry, supporting further research on the variability of examined traits in this noble broadleaved tree species.
Keywords
Drought, Selection, Prunus avium, Tissue Culture, Oxidative Stress
Introduction
Wild cherry (Prunus avium L.) is a noble tree species spread throughout Europe, Northern Africa, and Western Asia, mostly in mixed stands. Deep moist soils are suitable for its growth, but due to its high adaptability, it grows in a wide range of climatic and edaphic conditions ([47]). In addition to its importance in fruit production, there is considerable interest in its wood by the furniture industry, but it also has a significant role in agroforestry ([34]). Along with its economic role, it has ecological significance because the conservation of genetic variability of this species is considered to be an important part of the preservation of biodiversity, in general ([38]). Therefore, many European countries have developed long-term breeding projects to improve the species’ wood production and increase its tolerance to predominant biotic and abiotic stressors ([9], [28]).
Drought is an important abiotic stress, caused by insufficient water availability and water imbalance in the ecosystem. It leads to osmotic stress that can produce severe consequences in plants and causes considerable losses in the production of woody species. Due to climate change, plant species have faced new environmental conditions that intensively influence biological variability ([35]). Although covering an extensive geographical range and showing a high genetic variability, Wild cherry is considered to be negatively affected by upcoming/ongoing climate change due to its drought vulnerability ([5]). Considering the predictions for Southeastern Europe that include a rise in temperature and decrease of precipitation ([37]), the interest in drought tolerance studies in Wild cherry grows. In that sense, the selection of drought-tolerant varieties is seen as an important way of mitigating the problems caused by the expected climatic conditions ([9], [18]). Vuksanović et al. ([45]) also suggest that the basic approach to the mitigation of climate changes should be the breeding and cultivation of tolerant cultivars, particularly of forest tree species tolerant to drought. Rubio et al. ([33]) claim that drought-typical effects could be found as a result of the influence of other abiotic factors, such as stresses caused by salt, high and low temperatures. On the other hand, it is known that a combination of drought and other abiotic stress factors leads to a very specific plant response that is not an extrapolation of responses to separately applied stressors ([24]). Furthermore, the overlap of the responses at the transcriptomic and metabolic level among drought and other abiotic stress factors such as heat and salt stress appeared to be smaller than previously thought ([24]).
In order to optimize the evaluation process for drought tolerance, it is necessary to investigate the mechanisms of plants’ drought tolerance, especially in the case of forest tree species. Many studies on controlled water stress are based on osmotic stress induced by water-soluble, nonionic polymer polyethylene glycol (PEG - [19]). According to Osmolovskaya et al. ([27]), PEG-induced drought stress models are currently “state of the art”. Agar-based models are preferable since they allow avoiding or reducing the development of the hypoxic state. The response of plants to drought stress includes numerous changes in course of mitigation of the negative effect of water deficiency and can be monitored at multiple levels ([2]). Among them, special attention is given to the detrimental effect of drought-induced oxidative stress which presents the imbalance between reactive oxygen species (ROS) production and antioxidant defense systems ([36], [44]). Therefore, drought tolerance is intrinsically linked to antioxidant defense mechanisms ([30]), and investigation on antioxidant response could indicate more drought tolerant Wild cherry clones. To ameliorate oxidative stress, plants evolved complex enzymatic and non-enzymatic antioxidant systems that can neutralize and scavenge these ROS species and prevent the occurrence of oxidative stress in plants ([46]). Total phenolics and total flavonoids content, together with ROS-scavenging related tests are commonly used as markers of drought-related oxidative stress ([27], [43]).
Due to all limitations caused by multiannual growth and development of tree species, a large area that is required for experiments in the field conditions, as well as the influence of the external multifactorial stressors present in the natural environment, in vitro culture is one of the suitable models for fast examination of the forest trees’ tolerance to abiotic agents in relatively controlled conditions. In vitro culture has broad application in forestry and horticulture, presenting a low-cost and short-time mean for efficient mass production of healthy planting material with clonal purity, as well as evaluation of tolerance to abiotic agents ([43]). Mozafari et al. ([25]) reported that in vitro techniques are of great importance as a propagation tool for superior genotypes, especially in horticultural and fruit crops. Turhan & Baser ([41]) found a positive correlation between drought tolerance of genotypes in the laboratory and experiments under field conditions in soybean and sunflower, respectively. In that sense, there is a need for the implementation and improvement of in vitro tests as easily controlled models for the evaluation of drought tolerance in genotypes of forest tree species ([44]).
The aim of the study was twofold: (i) to examine the responses of two Wild cherry clones to drought-like conditions in vitro, induced by different concentrations of polyethylene glycol (PEG) in a growing medium; (ii) to evaluate the complex relationship between morphological, physiological, and biochemical traits using multivariate statistics. The aim was set in the context of drought tolerance variability, the necessity of preservation and improvement of biodiversity of Wild cherry, and its use in landscape architecture, horticulture, and forests regeneration.
The novelty of this research lies in the evaluation of antioxidant capacity according to appropriate tests and total phenolic and flavonoids content, and the study of relationship of these parameters to morphological and physiological traits. We assumed that the use of biochemical parameters in combination with morphological and physiological traits will improve the evaluation and selection of drought tolerant Wild cherry clones in vitro and give a clearer answer on differences between the two examined clones.
Material and methods
Plant material and experimental design
The study involved two clones of Wild cherry: Prunus avium L. clone 8A and P. avium L. clone 6A, which were selected in natural stand and multiplied by micropropagation in vitro by shoot tip culture to preserve clonal purity and avoid somaclonal variability. Both clones were obtained by vegetative propagation of accessions, chosen by positive selection for straight tree, vigorous growth, and tolerance to prevalent biotic agents from a natural population in Northern Serbia (45° 10′ N; 19° 18′ E; elevation 207 m a.s.l.) and transferred to the gene pool of the Institute of Lowland Forestry and Environment, University of Novi Sad, Serbia. These accessions were introduced in tissue culture in early spring of 2016, and regularly subcultured for four years until the experiment was set. The micropropagation was performed on solid modified MS basal growing medium ([26]) with added 6.5 g L-1 agar, 30 g L-1sucrose, 1 mg L-1 BA, 0.5 mg L-1 kinetin, 50 mg L-1 L-lysine, 50 mg L-1 adenine sulfate, and 100 mg L-1 myo-inositol. The pH of the media was adjusted to pH 5.8 by 1N HCl and 1N NaOH before sterilization. The cultures were grown for 35 days in controlled conditions at a temperature of t = 26 ± 2 °C, under a white light of 3500 lx m-2 emitted by LED lamps in 16h/8h day/night regime, and then subcultured in the same conditions for further propagation.
Drought tolerance of examined clones was tested on the same solid growing medium with three treatments that differed in the concentration of polyethylene glycol (PEG 6000): 0 (Control); 20 g L-1 (PEG20); and 50 g L-1 (PEG50). The concentrations of PEG were chosen to induce considerable osmotic stress in explants ([18]), and to achieve solidification of agar which can be a problem in such studies ([27]). For each Clone × PEG combination, three jars with five explants per jar (1.0 cm high shoot tips) were set. The experiment was set in a completely random design. Measured or average values at the level of the jar were treated as repetition and as such entered further statistical analysis.
Morphological, physiological, and biochemical analyses
After 35 days of cultivation, the height of the highest shoot (SH, mm) and the number of new shoots (NS) were recorded. Also, for every jar the percentage of explant survival (%) and percentage of explants with new shoots (i.e., percentage of multiplication - MP, %) were calculated. After the measurement of morphological parameters, the same plant material was used for the assessment of drought stress intensity on explants by physiological and biochemical parameters. Two samples of leaves and stems per jar were collected. Physiological measurements included parameters related to the content of photosynthetic pigments (mg g-1) in the plant tissues, determined by Lichtenthaler & Welburn ([22]) for the following pigments: chlorophyll a (Chl a), chlorophyll b (Chl b), and carotenoids (Car), and following derived parameters: chlorophyll a+b (Chl a+b) and chlorophyll a/b ratio (Chl a/b). In every sample, the following biochemical parameters related to oxidative stress were measured: total phenolic content (TPC, mg GAE g-1, where GAE stands for gallic acid equivalents - [20]), total flavonoids content (TFC, mg QE g-1, where QE stands for quercetin equivalents - [8]), ferric reducing antioxidant power (FRAP, mg AS g-1, where AS stands for ascorbic acid equivalents - [6] ), radical scavenging activity against ABTS·+ (2.2′-Azino-bis[3-ethylbenzthiazoline-6-sulfonic acid], ABTS, expressed in mM TE g-1, where TE stands for Trolox equivalents - [23]), and flavonoids/phenolics ratio (TFC/TPC). Ethanol extracts were obtained by extraction from 100 mg of grounded fresh plant material in 2 ml of 96% EtOH. Those extracts were then used to determine the content of photosynthetic pigments and measure biochemical parameters. All measured biochemical and photosynthetic parameters were determined by the spectrophotometric method on a MultiSkan™ GO spectrophotometer (Thermo Fisher Scientific, Waltham, MS, USA) in triplicates, i.e., three measurements per sample. Physiological and biochemical traits were measured or calculated both for leaves and stems. Physiological and biochemical traits were measured or calculated both for leaves and stems.
Statistical analyses
Two and three-way factorial analysis of variance (ANOVA) and Tukey’s honestly significant difference (HSD) test for a significance level of α = 0.05 were used for statistical analysis of data. The main effects (factors) examined were as follows: Clone and PEG concentration in morphological traits and Clone, PEG concentration, and Organ in physiological and biochemical traits. The NS and MP were transformed by square root transformation (√x+1) and arcsine transformation (arcsin √x), respectively, to meet the normal distribution of frequencies, which is required for used statistical tests. The relationships between examined traits were analyzed by Principal Component Analysis (PCA) according to their loadings with the first two principal components. The entering data was the matrix of Pearson’s correlation coefficients based on average values that are calculated at the level of interaction Clone × PEG concentration. For physiological and biochemical parameters, values calculated for leaves and stem entered PCA as separate parameters and in that sense were further discussed. Statistical analysis was performed by statistical program package STATISTICA® v. 14.0 ([40]).
Results
Variability of morphological traits
The percentage of explant’s survival in the experiment was 100% in all treatments. According to the results of the F-test of two-way ANOVA, variation of shoot height, the number of new shoots, and multiplication percentage was significantly influenced by both factors (Clone and PEG concentration). The effect of interaction Clone × PEG concentration was significant only for shoot height and number of new shoots (Tab. 1). The results of statistical analysis suggested a greater vigor of clone 8A than clone 6A by all examined morphological traits. Also, differences were significant between the total means of all examined PEG concentrations. There was a clear inhibitory effect of drought, which increased with the increase of concentration of PEG in the medium (data not shown).
Tab. 1 - Results of F-test on examined morphological characters in Wild cherry clones. (ns): non-significant; (*): p<0.05; (**): p<0.01.
Trait | Shoot height | Number of new shoots |
Multiplication percentage |
---|---|---|---|
Clone (A) | 27.292** | 55.158** | 11.1031** |
PEG concentration (B) | 21.405** | 100.939** | 31.6724** |
Interaction A×B | 4.478* | 11.044** | 0.0723ns |
The strongest inhibitory effect of drought was recorded on the NS, as the NS in plants cultivated on treatment PEG50 was more than ten times lower than that observed in the control treatment (Fig. 1b). The effect of drought on shoot height was relatively weak, being significant only in clone 8A. The SH measured on PEG50 treatment was 14.73 ± 2.25 mm in clone 6A and 17.07 ± 3.06 mm in clone 8A (Fig. 1a). Clone 8A achieved a higher multiplication rate on PEG50 treatment (39.36% with confidence interval CI0.05 = 20.3-60.3%) compared to clone 6A (9.25% with CI0.05 = 0.17-29.7% - Fig. 1c). Generally, the number of new shoots, as well as multiplication percentage were significantly higher on control than on media with PEG in both clones, but the inhibitory effect of water stress was stronger in clone 6A, suggesting its lower drought tolerance.
Fig. 1 - Changes in morphological traits of Wild cherry clones on media with different PEG concentrations. (a): Shoot height (SH); (b): number of new shoots (NS); (c): multiplication percentage (MP). Differences between values of the same traits that are labeled with the same letter are not statistically significant (p>0.05). Error bars represent confidence intervals (α = 0.05). (Control): 0; (PEG20): 20 g L-1 PEG; (PEG50): 50 g L-1 PEG.
Variability of physiological and biochemical traits
Statistically significant effect of factor Clone in examined physiological and biochemical traits was found only for Chl b, Car, FRAP, and ABTS assays. In contrast, variation of the majority of examined physiological and biochemical traits were significantly influenced by the main effects of Organ and PEG concentration (Tab. 2).
Tab. 2 - Results of the F-test for physiological and biochemical traits of Wild cherry clones. (Chl a): Chlorophyll a content; (Chl b): Chlorophyll b content; (Chl a+b): Chlorophyll a+b content; (Car): Carotenoid content; (Chl a/b): Chlorophyll a/b ratio; (TPC): Total phenolic content; (TFC): total flavonoids content; (FRAP): ferric reducing antioxidant power; (ABTS): radical scavenging activity against ABTS·+; (TFC/TPC): flavonoids/phenolics ratio; (ns): non-significant; (*): p<0.05; (**): p<0.01.
Group | Traits | Clone (A) | Organ (B) | c(PEG) (C) | Interaction A×B |
Interaction A×C |
Interaction B×C |
Interaction A×B×C |
---|---|---|---|---|---|---|---|---|
Physiological traits | Chl a | 1.08 ns | 231.12 ** | 17.05 ** | 9.11 ** | 1.24 ns | 8.85 ** | 4.76 * |
Chl b | 21.13 ** | 179.40 ** | 15.26 ** | 16.24 ** | 0.52 ns | 15.88 ** | 0.22 ns | |
Car | 18.75 ** | 38.24 ** | 4.35 * | 0.03 ns | 1.12 ns | 1.95 ns | 6.56 ** | |
Chl a+b | 1.78 ns | 267.69 ** | 20.47 ** | 14.88 ** | 1.11 ns | 13.35 ** | 1.64 ns | |
Chl a/b | 0.35 ns | 5.81 * | 7.84 ** | 4.07 * | 1.81 ns | 1.02 ns | 7.18 ** | |
Biochemical traits | TPC | 0.11 ns | 142.73 ** | 786.52 ** | 19.72 ** | 8.14 ** | 3.30 * | 170.24 ** |
TFC | 0.86 ns | 19.72 ** | 12.51 ** | 13.75 ** | 7.05 ** | 2.02 ns | 3.54 * | |
FRAP | 130.29 ** | 81.35 ** | 31.14 ** | 12.16 ** | 7.33 ** | 1.97 ns | 0.64 ns | |
ABTS | 47.22 ** | 66.20 ** | 54.38 ** | 17.85 ** | 18.97 ** | 94.08 ** | 12.87 ** | |
TFC/TPC | 4.87 * | 132.08 ** | 0.63 ns | 4.58 * | 4.33 ** | 21.84 ** | 15.70 ** |
Variability of physiological traits
According to statistical analysis, the Chl a content and Chl b and Car were significantly higher in leaves than in stem, while the opposite was for Chl a/b ratio. Also, there was a significant inhibitory effect of PEG50 treatment compared to the control treatment in all physiological traits except for the Chl a/b ratio. Differences between clones in total were significant in Chl b, which was higher in clone 6A (0.14 ± 0.05 mg g-1 FW) compared to clone 8A (0.08 ± 0.05 mg g-1 FW), and in Car which was higher in clone 8A (0.059 ± 0.026 mg g-1 FW) than in clone 6A (0.032 ± 0.026 mg g-1 FW - data not shown).
Measured values for Chl a content in PEG50 treatment ranged from 0.10 ± 0.05 mg g-1 FW in stems of clone 6A to 0.36 ± 0.10 mg g-1 FW in leaves of clone 8A (Fig. 2a). Also, at the same treatment (PEG50) in the stems, the lowest values for the content of Chl b, Car, and Chl a+b were measured in clone 6A (Fig. 2b, Fig. 2c, Fig. 2d). Interestingly, the content of Car in leaves of clone 8A was not significantly different in PEG50 treatment (0.089 ± 0.028 mg g-1 FW) compared to the control (0.078 ± 0.004 mg g-1 FW - Fig. 2c). For Chl b the inhibitory effect of treatment with 50 g L-1 PEG was significant in leaves of both clones, but in leaves of clone 8A, Chl b was four times higher in control treatment than in treatment with 50 g L-1 PEG, while in leaves of clone 6A it was two times higher. The Chl a+b content in the stems was lower by 84% (clone 6A) and 67% (clone 8A) compared to leaves in the control treatment. Also, the Chl a+b content in the PEG50 was 73% (clone 6A) and 56% (clone 8A) lower in stems than in leaves. The inhibitory effect of water stress was mostly evident in leaves, except for Chl a/b ratio (Fig. 2e), while in stem the only significant difference at this level was in Chl a/b ratio between control and treatment PEG50.
Fig. 2 - Changes in examined physiological traits in Wild cherry clones on media with different PEG concentrations. (a): Chlorophyll a content (Chl a); (b): Chlorophyll b content (Chl b); (c): Carotenoid content (Car); (d): Chlorophyll a+b content (Chl a+b); (e): Chlorophyll a/b ratio (Chl a/b). Differences between values of the same traits that are labeled with the same letter are not statistically significant (p>0.05). Error bars represent confidence intervals (α=0.05). (Control): 0; (PEG20): 20 g L-1 PEG; (PEG50): 50 g L-1 PEG.
Variability of biochemical traits
According to statistical analysis at the level of main effects, significant differences between examined clones were found only for FRAP, ABTS, and TFC/TPC ratio (data not shown). The reaction of clones in these three characters differed: 8A had a higher FRAP and TFC/TPC, but lower ABTS than clone 6A. The difference between organs was significant in all parameters of oxidative stress. The values for TPC and FRAP were higher in stems, while for TFC, ABTS and TFC/TPC they were higher in leaves. The inhibitory effect of drought was clear in the cases of TPC, TFC, and ABTS, while FRAP was significantly higher in the PEG50 than in the control treatment in clone 8A.
The highest differentiation at the level of second-order interaction Clone × Organ × PEG concentration was achieved in total phenolic content and ABTS (Fig. 3a, Fig. 3d). There was a clear trend of significantly decreased total phenolic content and total flavonoid content with increased PEG concentration (Fig. 3a, Fig. 3b). Considering clone 8A, TPC on PEG50 treatment in leaves was 80.8% lower relative to the control, while TFC in the leaves was 57.9% lower than in the control plants. However, while ABTS in the leaves of both clones was significantly higher in PEG20 than in PEG50 treatment, the opposite trend was recorded in the stem. Likewise, while FRAP increased with elevated concentration of PEG in both leaves and stems of clone 8A, there were no significant differences in FRAP values relative to PEG concentration in the leaves and the stems of clone 6A (Fig. 3c). TFC/TPC in the leaves of clone 8A increased and in stems of the same clone decreased in the presence of increased PEG concentration, while in clone 6A the influence of PEG50 treatment was not significant both in leaves and in stems (Fig. 3e).
Fig. 3 - Changes in biochemical traits in Wild cherry clones on media with different PEG concentrations. (a): total phenolic content (TPC); (b): total flavonoids content (TFC); (c): ferric reducing antioxidant power (FRAP); (d): radical scavenging activity against ABTS·+ (ABTS); (e): flavonoids/phenolics ratio (TFC/TPC). (Control): 0; (PEG20): 20 g L-1 PEG; (PEG50): 50 g L-1 PEG. Differences between values of the same traits that are labeled with the same letter are not statistically significant (p>0.05). Error bars represent confidence intervals (α=0.05).
Relationship between examined traits
According to the first two principal components that accounted for 71.7% of the total variance, there was a close relationship between morphological parameters that achieved their highest loadings onto the first principal component that explained 44.47 % of the total variation (Fig. 4). Physiological parameters, measured within the same organ, were in a relatively close relationship. In contrast, a weak correlation between physiological parameters measured in the leaves and the stems was observed. Parameters FRAP and ABTS were in negative correlation and for both of them, measurements between leaves and stem were in positive correlation. There was a strong positive correlation between TPC measured in the leaves and the stems, but weak in the case of TFC. However, a strong negative correlation was recorded between TFC/TPC measured in the leaves and the stems. The parameter TFC/TPC in both stem and leaves, as well as TFC in the stems, achieved the strongest, while FRAP and ABTS achieved the weakest correlation with examined morphometric parameters.
Fig. 4 - Loadings of the first two principal components for physiological and biochemical parameters. (SH): shoot height; (NS): number of new shoots; (MP): percentage of multiplication; (Chl a): Chlorophyll a content; (Chl b): Chlorophyll b content; (Chl a+b): Chlorophyll a+b content; (Car): Carotenoid content; (Chl a/b): Chlorophyll a/b ratio; (TPC): Total phenolic content; (TFC): total flavonoids content; (FRAP): ferric reducing antioxidant power; (ABTS): radical scavenging activity against ABTS•+; (TFC/TPC): flavonoids/phenolics ratio; (L): leaves; (S): stems.
Discussion
In the present study, we examined the effects of two levels of water stress in vitro on two clones of Wild cherry. The levels of water stress were induced by two different concentrations of polyethylene glycol. The study of differences in the reaction of two clones on PEG concentrations was mainly based on a comparison of results gained on media with PEG to those recorded on control treatment. In this context, we hypothesized that studied accessions will demonstrate different performances in response to drought-induced stress, therefore facilitating the identification of those clones that might be potentially used for reforestation of drought-prone environments. As references, we selected well-established morphological, physiological, and biochemical parameters. Morphological response to water stress in this study resulted in the reduction of all measured morphological parameters: shoot height, number of new shoots, and multiplication percentage (Fig. 5). A similar inhibitory effect of 50 g L-1 PEG on the same morphological traits was obtained by Vuksanović et al. ([44]) in White poplar clones grown in vitro. Our results are also in concordance with other studies which reported the negative effect of PEG on shoot height in plants grown in vitro ([41], [14]). Growth reduction of Wild cherry explants with increasing concentration of PEG in micropropagation medium could be probably explained as a result of osmotic stress, reduced turgor pressure, and limitation of nutrient uptake. Anjum et al. ([3]) reported that under drought conditions, cell elongation can be inhibited by interruption of water flow from the xylem to the surrounding elongating cells. Based on presented data for morphological traits, clone 8A was characterized by a higher tolerance to drought-like conditions in vitro compared to clone 6A. Considering presented results for morphological parameters, particularly the clear differentiation of two examined clones by the difference between PEG50 and control treatment in SH and MP, medium with 50 g L-1 PEG could be proposed for further Wild cherry drought tolerance studies in vitro based on these morphological traits.
Fig. 5 - Explants of clone 8A after 35-days cultivation on examined PEG concentrations. (Control): 0; (PEG20): 20 g L-1 PEG; (PEG50): 50 g L-1 PEG.
The content of photosynthetic pigments, including chlorophyll and carotenoids, is an important indicator of plant physiological status ([11]). Drought conditions were frequently described to reduce the content of photosynthetic pigments, further decreasing plants’ photosynthesis and growth ([17]). Osmolovskaya et al. ([27]) stressed the importance of drought-related oxidative stress in the decrease of chlorophyll content. Indeed, Guo et al. ([12]) reported the inhibitory effect of drought on chlorophyll concentration and intensity of photosynthesis in three hybrid poplar (Populus deltoides × Populus nigra) clones, whereas Jaleel et al. ([16]) found reduced chlorophyll content in Catharanthus roseus L. plants subjected to water stress. Kovalikova et al. ([21]) also found a decrease in the content of chlorophyll a and b in leaves of cherry cultured on a growing medium with 50 g L-1 PEG in vitro, as well as a difference in the response of examined cultivars by these parameters. Thus, the reduction of chlorophyll a content and chlorophyll b content caused by water stress in our study, particularly in leaves, is in accordance with the general opinion.
However, while the reduction of carotenoids was significant in leaves of clone 6A, there was no significant reduction of carotenoid content in leaves of clone 8A. This difference in response of examined clones is interesting, considering the work of Jaleel et al. ([17]) who reported the important role of carotenoids of different crop species in coping with drought stress due to their antioxidant activity. In this way, they contribute to the total plant antioxidant defense system, and therefore probably have a similar role in the alleviation of drought stress in Wild cherry as well.
According to Ashraf & Harris ([4]), Chl a/b ratio increase drought-induced stress, but they also notified cases of opposite reactions. In our study, the Chl a/b ratio at the treatment with 50 g L-1 PEG significantly increased in stems of clone 6A cultivated under control treatment. However, Viljevac et al. ([42]) recorded a reduction of the Chl a/b ratio, with a difference in response between two Sour cherry cultivars exposed to drought in greenhouse conditions. These findings suggest a need for further study of the possible role of Chl a/b in the evaluation of Wild cherry tolerance to water stress.
Total flavonoids content and total phenolic content were found to change significantly in tissue culture of many tree species regarding the severity of water stress they had been exposed to. Also, the reactions to water stress by these parameters usually differed between genotypes ([45], [18]). In our study, the close relationship of TFC in stem and TPC in leaves and stem with morphological traits was found. It is in concordance with the fact that these metabolites complement the enzymatic antioxidant system and have a considerable potential to prevent cell damage and reduce ROS. Their biosynthesis and accumulation are generally induced in response to biotic and abiotic stimuli such as drought stress in plant tissues ([39]). However, the results of our study also suggested poor accumulation of total phenolics in severe drought conditions. It was in concordance with the results of Puente-Garza et al. ([31]) who found a decrement of flavonoid and phenolic content under drought stress in Agave salmiana Otto ex Salm-Dyc, which has been classified as stress-tolerant by Escamilla-Treviño ([10]). Ahmad et al. ([1]) found increment of TPC and TFC in leaves of shoot gained by direct organogenesis of Stevia rebaudiana on MS solid media with PEG concentration from 0 to 2%, but TPC on medium with 4% was significantly lower than on medium with 2% PEG. A reduction of total phenolic content in Solanum tuberosum L. exposed to drought stress in vitro was noted by Hamooh et al. ([13]). Results similar to ours were reported by Popović et al. ([29]) and Popović et al. ([30]), who found a significant reduction in total phenol content in leaves of Populus × canadensis genotypes at the highest level of osmotic stress (200 mOsm PEG). Finally, our findings supported the results of Roussos ([32]), who evidenced the reduction of both total phenolic and flavonols’ content as a consequence of the drought effect caused by the presence of mannitol. Bettaieb et al. ([7]) suggested that opposite to the increment of phenolic biosynthesis in mild drought conditions, under severe drought stress, the enzymes involved in their biosynthesis such as phenylalanine ammonia-lyase (PAL) could be partially inhibited, leading to reduced phenolic content. Decreased PAL activity due to drought was observed in Euramerican poplar ([30]). Also, Roussos ([32]) found that the reduction of both phenolic and flavanols’ content was accompanied by accumulation of glucose, mannitol, and pinitol, which mitigated osmotic stress. Jiroutova et al. ([18]) also reported intense accumulation of osmoprotectants, such as proline and sorbitol, as well as the increase of activity of some important antioxidant enzymes in explants of apple and cherry cultivars on a medium with 50 g L-1 PEG, emphasizing the significance of their role in the mitigation of effects of severe drought stress. Considering the results of both our and cited studies, we assume that lower content of total phenolics and total flavonoids in plants grown on PEG50 than in control indicates severe drought conditions provided by this treatment. Also, it seems that the reduced accumulation of TPC and TFC on PEG50 found in our study could be related to the increased accumulation of osmoprotectants with considerable antioxidant activity found in similar conditions by Jiroutova et al. ([18]). This is in accordance with the result notified in our study, by which accession 8A, considered to be more drought-tolerant according to the response by morphometric traits, achieved stronger decrement of TPC and TFC in leaves on PEG50 compared to control treatment than accession 6A.
The TFC/TPC ratio was considerably higher in leaves and lower in stems in treatment with 50 g L-1 PEG comparing to the control treatment. Although this parameter had been rarely used in similar studies ([45]), it appeared to be important because of its strong correlation with morphometric traits, which was negative for TFC/TPC in leaves, and positive for TFC/TPC in stems. These results additionally stressed the importance of flavonoids in the evaluation of drought tolerance, which are a well-known group of phenolic compounds that contributes greatly to antioxidant properties of the plants since it exhibits a wide range of antioxidant properties against various ROS by scavenging effect ([35]). In the study of evaluation of low pH stress tolerance in White poplar clones, Vuksanović et al. ([45]) particularly favorized the TFC/TPC ratio as a parameter that considerably contributed to the differentiation of clones. Regarding the difference in TFC/TPC between treatment with 50 g L-1 PEG and control treatment both in leaves and stems, the response (positive in leaves and negative in stems) was more intense in clone 8A, while in 6A it was not statistically significant. Such response is related to a considerable decrease of TPC in leaves and reduction of TFC in stems due to severe drought conditions on PEG50, processes that were considerably intense in the drought-tolerant clone 8A.
In the case of FRAP and ABTS, as widely used parameters of oxidative stress, both organs had a similar response: higher FRAP values, that stands for a reducing capacity of extracts and lower ability of the extract to neutralize ABTS•+ radical, were obtained in PEG50 treatment compared to control treatment. In their study, Jiroutova et al. ([18]) found a significant decrement of scavenging activity of DPPH•+ (2.2-diphenyl-1-picrylhydrazyl) radical in cherry (Prunus avium) cultivars, cultivated in vitro on media with 25 and 50 g L-1 PEG in comparison to the control. Cultivars differed in the degree of that DPPH•+ decrement. Clone 8A presented significantly higher FRAP in both organs and lower ABTS in leaves at treatment with 50 g L-1 PEG compared to control treatment, but clone 6A did not, suggesting that increase of FRAP and decrease of ABTS in drought stress could be characteristic of a drought-tolerant clone. However, the correlations of FRAP and ABTS with morphological traits were weak except moderate correlation of ABTS in leaves. This suggests the necessity for further study of the importance of these assays in the evaluation of drought tolerance in Wild cherry. According to PCA results, the particularly close relationship between phenolic and flavonoid content, on one side, and FRAP and ABTS, on the other, was recorded in the leaves. The correlation with FRAP was negative, and positive with ABTS (Fig. 4). ABTS measured in leaves achieved a strong correlation with TPC and TFC/TPC ratio, which additionally suggests higher reliability of this assay in the evaluation of drought tolerance in Wild cherry. The difference in reaction by FRAP and ABTS could be in concordance with high pH-dependence of both biochemical assays on different levels of phenolic protonation since ABTS assay is performed at neutral pH, while FRAP assay is done under alkaline pH conditions ([15], [35]). So, it indicated changes in the accumulation of various phenolics related to oxidative stress mitigation that differ in protonation under neutral and alkaline pH conditions and consequently influence the results of the assays. In addition, it is well known that besides oxidative stress, increased PEG concentration intensifies osmotic stress, stimulating biosynthesis of some osmolytes that also contribute to the total antioxidant power, such as proline, polyamines, or glycine betaine ([30], [18]), which can also contribute to the difference between two tests.
The results presented in this study support the use of in vitro evaluation of drought tolerance in Wild cherry accessions. Vuksanović et al. ([44]) also stressed that in vitro technique could be useful to narrow the group of candidates for introduction to plantation production, but the final decision should also be based on trials in field conditions. We assume that due to numerous benefits that in vitro testing of drought tolerance offers, especially in perennial woody species, the study should be continued in a course of investigation of variability in the response of different accessions, use of additional parameters and optimization of the procedures.
Conclusions
The examined morphological and biochemical parameters appeared to be more convenient for the evaluation of drought tolerance in vitro than physiological parameters. The most promising traits for the evaluation of drought tolerance examined in our study are morphological traits and biochemical parameters. In particular, interesting parameters are those related to the content of flavonoids and phenolics, where most of them were in strong correlation with morphometric traits. We assume that the Wild cherry accession 8A was more tolerant to water stress than 6A because it was characterized by more intensive growth, higher MP, higher values of carotenoids, more intense decrement of ABTS and increment of FRAP values on treatment with 50 g L-1 PEG, compared to the clone 6A. The results obtained in this study suggest that further research on the drought tolerance of Wild cherry clones in vitro should be performed on a medium with 50 g L-1 PEG. Also, this study should be continued especially regarding variability in the response of different clones on drought stress by the examined and additional parameters in order to optimize the evaluation procedure.
List of abbreviations
PEG: polyethylene glycol; BA: 6-Benzylaminopurine; Chl a: chlorophyll a content; Chl b: chlorophyll b content; Chl a+b: chlorophyll a+b content; Car: carotenoid content; Chl a/b: chlorophyll a/b ratio; TPC: total phenolics content; TFC: total flavonoids content; FRAP: ferric reducing antioxidant power; ABTS: radical scavenging activity against ABTS·+ (2.2′-Azino-Bis-3-Ethylbenzothiazoline-6-Sulfonic Acid); TFC/TPC:, flavonoids/phenolics ratio; (L): leaves; (S): stems; GAE: gallic acid equivalents; QE: quercetin equivalents; AS: ascorbic equivalents; TE: Trolox equivalents; FW: fresh weight; PEG20: polyethylene glycol concentration 20 g L-1; PEG50: polyethylene glycol concentration 50 g L-1; SH: height shoot; NS: number of new shoots; MP: percentage of multiplication.
Acknowledgments
We acknowledge the Ministry of Education, Science and Technological Development of the Republic of Serbia.
Funding
This work was supported by the Ministry of Education, Science and Technological Development of the Republic of Serbia under Grant no. 451-03-9/2021-14/200117 and 451-03-9/2021-14/200197.
Conflicts of Interest
The authors declare no conflict of interest.
Author contributions
OS, KB, GV, VV, and SS contributed the ideas and designed the study. Material preparation and measurements of morphological, physiological, and biochemical analyses were performed by VV, KB, and KL. The statistical analysis was performed by VV and KB. VV and KB wrote the first version of the manuscript and all of the authors contributed critically to the drafts and gave final approval for publication.
References
Gscholar
Gscholar
Gscholar
Gscholar
CrossRef | Gscholar
CrossRef | Gscholar
Gscholar
Gscholar
Gscholar
Gscholar
Gscholar
Gscholar
Authors’ Info
Authors’ Affiliation
Saša Orlović 0000-0002-2724-1862
University of Novi Sad, Faculty of Agriculture, Trg Dositeja Obradovića 8, 21000 Novi Sad (Serbia)
Srdan Stojnić
Marko Kebert 0000-0003-0171-6150
Lazar Kesić 0000-0003-2643-9727
Vladislava Galović
University of Novi Sad, Institute of Lowland Forestry and Environment, Antona Cehova 13d, 21000 Novi Sad (Serbia)
Corresponding author
Paper Info
Citation
Vuksanović V, Kovačević B, Stojnić S, Kebert M, Kesić L, Galović V, Orlović S (2022). Variability of tolerance of Wild cherry clones to PEG-induced osmotic stress in vitro. iForest 15: 265-272. - doi: 10.3832/ifor4033-015
Academic Editor
Federica Brunoni
Paper history
Received: Dec 03, 2021
Accepted: May 11, 2022
First online: Jul 25, 2022
Publication Date: Aug 31, 2022
Publication Time: 2.50 months
Copyright Information
© SISEF - The Italian Society of Silviculture and Forest Ecology 2022
Open Access
This article is distributed under the terms of the Creative Commons Attribution-Non Commercial 4.0 International (https://creativecommons.org/licenses/by-nc/4.0/), which permits unrestricted use, distribution, and reproduction in any medium, provided you give appropriate credit to the original author(s) and the source, provide a link to the Creative Commons license, and indicate if changes were made.
Web Metrics
Breakdown by View Type
Article Usage
Total Article Views: 27304
(from publication date up to now)
Breakdown by View Type
HTML Page Views: 23497
Abstract Page Views: 2337
PDF Downloads: 1092
Citation/Reference Downloads: 1
XML Downloads: 377
Web Metrics
Days since publication: 1071
Overall contacts: 27304
Avg. contacts per week: 178.46
Article Citations
Article citations are based on data periodically collected from the Clarivate Web of Science web site
(last update: Mar 2025)
Total number of cites (since 2022): 8
Average cites per year: 2.00
Publication Metrics
by Dimensions ©
Articles citing this article
List of the papers citing this article based on CrossRef Cited-by.
Related Contents
iForest Similar Articles
Research Articles
Effect of drought stress on some growth, morphological, physiological, and biochemical parameters of two different populations of Quercus brantii
vol. 11, pp. 212-220 (online: 01 March 2018)
Research Articles
Genetic monitoring in forests - early warning and controlling system for ecosystemic changes
vol. 4, pp. 77-81 (online: 05 April 2011)
Research Articles
Oak sprouts grow better than seedlings under drought stress
vol. 9, pp. 529-535 (online: 17 March 2016)
Research Articles
Links between phenology and ecophysiology in a European beech forest
vol. 8, pp. 438-447 (online: 15 December 2014)
Research Articles
Magnolia grandiflora L. shows better responses to drought than Magnolia × soulangeana in urban environment
vol. 13, pp. 575-583 (online: 07 December 2020)
Research Articles
A physiological approach for pre-selection of Eucalyptus clones resistant to drought
vol. 13, pp. 16-23 (online: 15 January 2020)
Short Communications
Preliminary indications for diverging heat and drought sensitivities in Norway spruce and Scots pine in Central Europe
vol. 13, pp. 89-91 (online: 01 March 2020)
Research Articles
Tracing the acclimation of European beech (Fagus sylvatica L.) populations to climatic stress by analyzing the antioxidant system
vol. 14, pp. 95-103 (online: 01 March 2021)
Research Articles
Effect of salt and drought on growth, physiological and biochemical responses of two Tamarix species
vol. 8, pp. 772-779 (online: 25 March 2015)
Research Articles
Use of δ13C as water stress indicator and potential silvicultural decision support tool in Pinus radiata stand management in South Africa
vol. 12, pp. 51-60 (online: 24 January 2019)
iForest Database Search
Search By Author
Search By Keyword
Google Scholar Search
Citing Articles
Search By Author
Search By Keywords
PubMed Search
Search By Author
Search By Keyword