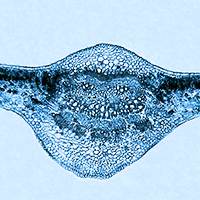
Assessment of cadmium tolerance and phytoextraction ability in young Populus deltoides L. and Populus × euramericana plants through morpho-anatomical and physiological responses to growth in cadmium enriched soil
iForest - Biogeosciences and Forestry, Volume 10, Issue 3, Pages 635-644 (2017)
doi: https://doi.org/10.3832/ifor2165-010
Published: Jun 01, 2017 - Copyright © 2017 SISEF
Research Articles
Abstract
Fast growing woody plants represent effective tools for cadmium (Cd) extraction during remediation of low to medium Cd contaminated soils. Poplars are good candidates for this task because of their rapid growth rate, high biomass yield, and adaptability, as well as the availability of well-characterized clones/ genotypes with various anatomical and physiological traits. The present study evaluates the potential of Populus deltoides (clone B-81) and Populus × euramericana (clone Pannonia) for phytoremediation of Cd contamination in soil. Poplar clones were analyzed for (1) plant growth response to Cd contamination, (2) Cd accumulation, translocation, and partitioning between plant organs, and (3) morphological, anatomical and physiological responses to Cd stress as a function of biomass production. Plants were cultivated in soil moderately contaminated with Cd (8.14 mg kg-1 soil) under semi-controlled conditions for six weeks. Our results suggest that P. × euramericana and P. deltoides clones respond differently to Cd contamination. Biomass production and morphological characteristics were more negatively affected in P. × euramericana than in P. deltoides plants. However, most examined leaf structural parameters were not significantly affected by Cd. In most cases, photosynthetic characteristics and gas exchange parameters were affected by Cd treatment, but the levels and patterns of changes depended on the clone. High tolerance to applied Cd levels, as estimated by the tolerance index, was observed in both clones, but was higher in P. deltoides than P. × euramericana (82.2 vs. 66.5, respectively). We suspect that the higher tolerance to Cd toxicity observed in P. deltoides could be related to unchanged proline content and undisturbed nitrogen metabolism. Following treatment, 58.0 and 46.7% of the total Cd content was accumulated in the roots of P. × euramericana and P. deltoides, respectively, with the remainder in the stems (18.2 and 39.9%) and leaves (23.8 and 13.4%). In summary, P. deltoides displayed better phytoextraction performance under Cd exposure than P. × euramericana, suggesting its potential not only for Cd phytostabilization, but also phytoextraction projects.
Keywords
Introduction
Cadmium (Cd) is a non-essential heavy metal for plants, and is commonly considered to be an extremely significant pollutant due to its high toxicity for all living organisms ([34]). Widespread sources of Cd release, such as mining, cultivation of plants for energy production and agricultural activities, have caused vast areas of land to become unproductive and even hazardous for wildlife and humans, and have diminished the availability of arable land. Furthermore, crop plants can accumulate and translocate contaminants such as Cd in edible parts ([27], [21]). Because of this, European Union regulatory legislation has set the recommended upper limit for Cd contamination in agricultural soil at 3 µg g-1 ([14]). Thus, development of methods for efficient removal of toxic elements, such as Cd from contaminated soil is necessary for prevention of Cd transfer into the human food chain. As an alternative to conventional methods, various phytoremediation techniques have been proposed employing woody plant species to either remove pollutants from contaminated soils or render them harmless ([39]). One of these techniques, referred to as phytoextraction, involves the use of plants for extraction of heavy metals from contaminated soils, and appears to be a promising method for remediation of low to medium levels of soil contamination. Importantly, phytoextraction of low to medium concentrations of heavy metals does not significantly disturb plant morpho-physiological processes, allowing normal growth and development, i.e., production of biomass coupled with soil decontamination.
Along with other fast growing tree species, poplars are considered to be multifunctional trees, displaying potential for environmental restoration and land reclamation, together with high biomass production convenient for bioenergy or paper production ([39]).
Reliable evaluation of heavy metal tolerance and phytoremediation potential in poplar species should be conducted by screening the physiological and morphological characteristics of plants exposed to the contaminant metal of interest in the soil. Plant growth, gas exchange parameters (photosynthetic rate, stomatal conductance, substomatal CO2 concentration), chlorophyll content and chlorophyll a fluorescence parameters are often used as indicators of possible disturbances triggered by heavy metal contamination ([6], [7]). In addition, the rate of photosynthesis has often been correlated with biomass production, and thus might be used as an indicator of the growth potential of tree species and genotypes under pollutant exposure. Cd causes structural and functional alterations in photosynthetic apparatus, resulting in reduced or inhibited photosynthesis ([31]). Furthermore, Cd toxicity may provoke an increase in peroxidation of chloroplast membrane lipids by enhancement of lipoxygenase activity, resulting in disorganization of thylakoid membranes and decreased concentration of both chlorophylls and carotenoids ([12]). High Cd concentrations in plant tissues can also affect other physiological processes, such as water regime, mineral nutrient uptake and ion metabolism ([31]). Optimal supply of nitrogen and other mineral nutrients could alleviate Cd-induced toxic effects in plants by enhancing biochemical reactions and physiological processes, along with reduced Cd accumulation ([27]).
Inhibition of nitrate reductase (NR) activity in plants experiencing Cd toxicity leads to reduced nitrate assimilation ([4]) and nitrogen deficiency. In order to obtain the nitrogen necessary for the synthesis of other organic compounds under conditions of N deficiency, degradation of proline is favored by stimulation of proline dehydrogenase activity, resulting in production of glutamate utilized as a nitrogenous source for the synthesis of other amino acids ([11]). Furthermore, proline alleviates heavy metal stress in plants through direct metal binding, as well as enhancement of antioxidant signaling and defenses ([35]).
There is little information in the literature on the role of leaf anatomic structure in photosynthetic performance under heavy metal stress ([36]). In spite of structural and ultrastructural changes to leaf lamina, photosynthetic balance and biomass production in P. deltoides might be sustained by the fact that photosynthetic pigment concentrations remain unchanged under heavy metal stress ([37]). Thicknesses of the spongy and palisade parenchyma are associated with biomass production and could be used as indicators of growth potential in poplars ([3]). Therefore, the interrelation between micromorphological parameters together with physiological and biochemical characteristics is necessary for identification of plants suitable for phytoremediation ([23]).
The present study was designed to evaluate the phytoremediation potential of six week old Populus × euramericana and Populus deltoides plants cultivated under semi controlled conditions, in pots containing soil artificially contaminated with Cd. Poplar clones were analyzed for: (1) plant growth response to Cd contamination; (2) Cd accumulation, translocation, and partitioning between plant organs; and (3) morphological, anatomical and physiological responses to Cd stress as a function of biomass production. Obtained results may be used as reliable guidelines in selection of genotypes with high Cd tolerance and phytoremediation potential for efficient use in projects aimed at reclamation of Cd polluted sites.
Material and methods
Plant material and cultivation conditions
Soil pot culture experiments were conducted in a greenhouse (semi-controlled conditions) at the Department of Biology and Ecology, Faculty of Sciences, University of Novi Sad, Republic of Serbia. The temperature range was 18/30 °C (night/ day) and illumination was natural and dependent on outside light conditions. Relative air humidity ranged from 70 to 80%. Two poplar clones were selected from an assortment established at the Institute of Lowland Forestry and Environment (Novi Sad, Republic of Serbia): Populus deltoides (clone B-81) and a hybrid poplar Populus × euramericana (clone Pannonia). The seedling nursery is located in the Panonian plane in northern Serbia (45° 18′ 15″ N, 19° 56′ 31″ E). Dormant hardwood cuttings of both poplar clones were collected from one-year-old shoots in the beginning of February, and kept in a cool chamber until use. Soil (fine sandy loam) was collected from the seedling nursery (0-20 cm depth) and its physico-chemical properties are presented in Tab. 1. One month prior to planting, dry soil was sieved and enriched with cadmium by spraying aqueous solution of CdCl2·H2O, and thoroughly mixed (the experiment was set up in May). For both clones, two treatments - (1) control, 0 mg kg-1 of Cd; (2) Cd treatment, 8.14 mg kg-1 of Cd - were set up with four replicates (pots). Hardwood cuttings were soaked in water for 24 h before use. Six cuttings (20 cm long of similar diameter) were planted in a single pot, containing 5 l of either Cd-free (control) or Cd-enriched soil. During the cultivation period, plants were allowed to develop only one sprout per cutting, by cutting off extra sprouts. Soil moisture was maintained between 60 and 80% of soil water capacity throughout the experimental period using tap water. Three weeks after planting, 0.2 l of full-strength Hoagland nutrient solution was added to the pots. After 6 weeks of cultivation, the morphological and physiological traits of both clones were measured, prior to harvesting for chemical analysis. The fifth fully-expanded leaf (counted from the apex of the shoot) was used for either in vivo or in vitro measurements of physiological parameters, as well as structural characteristics of leaf lamina. Ten leaves were sampled from all plants in the same treatment group within a clone for analysis of structural characteristics. Other leaves of the same age were collected and pooled to obtain a representative sample used for determination of photosynthetic pigments, proline concentration, nitrate reductase activity and chemical analyses.
Tab. 1 - Physico-chemical characteristics of soil in the seedling nursery.
Characteristic | Value | |
---|---|---|
Coarse sand(%) | 0.6 | |
Find sand(%) | 76.4 | |
Powder(%) | 13.2 | |
Clay(%) | 9.88 | |
pH | in KCl | 7.57 |
in H2O | 8.45 | |
CaCO3 (%) | 17.25 | |
Humus(%) | 1.48 | |
Total N(%) | 0.12 |
Growth parameters
Shoots were immediately harvested after determination of physiological parameters, and leaves were separated from stems. Roots were carefully separated from the soil and gently washed using tap and deionized water. The total leaf area was measured using a portable leaf area meter (Li-Cor 3000®, Lambda Instruments Corporation, Lincoln, NE, USA). Fresh weight of leaves, roots and stems, as well as stem height and basal diameter (1 cm above the insertion to the rooted cutting) were measured, and samples were completely dried (80 °C, 48h) and milled (A11 basic, IKA®, Werke, Germany). Biomass production and growth parameters of six individual plants was determined for each treatment and each clone (n=6).
Physiological parameters
Rate of photosynthesis (Pn) and transpiration (Tr), stomatal conductance (gs), and intercellular concentrations of CO2 (Ci) were determined using a LCpro+ portable photosynthetic system (ADC BioScientific Ltd, Hoddesdon, UK). Light conditions were set using the Lcpro+ light unit to emit photosynthetically active radiation (PAR) at 1000 µmol m-2 s-1. A flow of ambient air to the leaf chamber was provided by the air supply unit at a constant rate of 100 µmol s-1. Temperature, CO2 concentration, and humidity were at ambient levels. The gas exchange parameters of six individual plants were determined for each treatment and each clone (n=6). After measurement of gas exchange parameters, the same leaves were collected and used for estimation of photosynthetic pigments and proline concentration, as well as for measurement of nitrate reductase activity. Concentration of chlorophylls and carotenoids was determined according to the method of Von Wettstein ([41]). Fresh plant material was homogenized with absolute acetone using a pestle and mortar, and the homogenate was filtered. The filtrate was used to measure absorbance spectrophotometrically (Beckman DU-65®, Beckman Coulter, Brea, CA, USA) at 662, 644 and 440 nm. Concentrations of photosynthetic pigments were calculated and expressed as mg g-1 of dry weight. The concentration of free proline was estimated spectrophotometrically using the method of Bates ([5]). Plant material was homegenized with 10 ml of 3% sulfosalicylic acid and the homogenate was filtered. The mixture containing 2 ml of filtrate, 2 ml of glacial acetic acid and 2 ml of acid ninhydrin reagent was incubated in a boiling water bath for 15 minutes. After cooling, four ml toluene was added to the mixture. Absorbance of the proline-nynhidrin complex extracted in toluene was measured spectrophotometrically (Beckman DU-65) at 520 nm. Proline concentration was expressed as μg g-1 fresh weight. Nitrate reductase (NR) activity was determined using an in vivo method as follows: samples of leaf lamina without the main vein (0.2 g) were incubated in a buffered medium containing NO3-, and the concentration of nitrites produced over time as the product of nitrate reductase activity were measured spectrophotometrically at 520 nm ([16]). NR activity was calculated using calibration curves, and expressed as µM NO2- g-1 h-1. Analysis of photosynthetic pigments and proline concentration, as well as NR activity, was performed in five replicates for each treatment and each clone (n=5).
Structural characteristics of leaf lamina
Leaves (the fifth leaf from the apex of the shoot) were sampled at the end of the experimental period for each treatment and clone, and fixed in 50% ethanol. Anatomical parameters were analyzed on samples excised from the section between the third and fourth lateral vein of the lamina and the main vein section. 25 µm thick cross sections were made using a Leica CM 1850® cryostat (Leica, Wetzlar, Germany) at a temperature of -20 °C. Measurements were performed by Image Analysing System Motic Images Plus. Relative proportions of adaxial and abaxial epidermis, mesophyll, spongy and palisade tissues were calculated as a ratio versus the full lamina thickness (taken as 100%). The main vein index was calculated as follows: leaf blade thickness at the main vein/leaf blade thickness at 1/4 of the leaf blade width.
Chemical analysis of plant samples
After biomass measurements, leaves, stems and roots were oven-dried at 80 °C until a constant weight was obtained. Plant samples were digested with HNO3 using a closed vessel microwave digestion system (Milestone D series Microwave Digestion System, Milestone Inc, Shelton, CT, USA). Concentrations of Cd were determined by employing flame atomic absorption spectrophotometry (AAS240FS®, Varian Medical Systems Inc, Palo Alto, CA, USA). Analyses of plant samples were performed in triplicate (n=3) for each treatment and each clone. Cd concentrations in leaves, stems and roots (μg g-1) were calculated on the basis of dry weight as follows: reading (µg Cd ml-1) × dilution factor (ml) / dry weight (g).
Calculations and statistical analysis
In order to estimate poplar clone performance and phytoremediation potential, tolerance index (Ti - [42]), biological concentration (BCF) and translocation (Tf) factors ([44]) for individual plant organs were calculated. Tolerance index was calculated as follows: fresh weight of plants treated with Cd × 100 / fresh weight of control plants. Biological concentration factor for leaves, stems and roots were evaluated as the ratio of Cd concentration in plant organs to Cd concentration in soil. Translocation of Cd from roots to leaves and stems (Tf) was calculated as follows: concentration of Cd in leaves or stems/concentration of Cd in the root. Obtained data were calculated and statistically processed using Microsoft Office Excel® and STATISTICA® for Windows ver. 12.0 (StatSoft, Tulsa, OK, USA). Independent t-test or Duncan’s test were used to compare the mean values of studied parameters obtained for control and treated plants, within or between individual clones. A two-way analysis of variance (ANOVA) was performed to detect significance levels (P values) for effects of clone, treatment and their interactions. Relationships between morphological, anatomical and physiological parameters, Cd accumulation, and biomass production in poplar plants treated with Cd were evaluated by Pearson’s correlation coefficient.
Results and discussion
In the present study we investigated morpho-physiological responses and leaf structural characteristics of two poplar clones cultivated in soil moderately contaminated with Cd, in order to estimate their performance and phytoextraction potential.
Biomass production and plant tolerance
Previous investigations had commonly reported stunted growth of roots and stems as an effect of Cd toxicity in plants, because of the tight relationships between biomass production and changes in photosynthetic activity, root characteristics, mineral elements and plant water supplies. Growth parameters of the poplar genotypes included in the present study are presented in Tab. 2. Two-way ANOVA results suggest that most of the growth parameters were affected by clone and Cd treatment. However, the genotypes differently responded to Cd treatment with respect to stem height and diameter. Total biomass, as well as the mass of leaves and roots, was significantly reduced in plants exposed to Cd with respect to control, resulting in a decrease of Ti in both genotypes. In both clones, the obtained results suggest that root growth was more negatively affected by Cd than shoot growth. However, reduced root biomass was more evident in Populus × euramericana than in P. deltoides plants, probably due to higher accumulation of Cd in Populus × euramericana vs. P. deltoides. Decreased root growth, along with other Cd-induced alterations, limit the potential of roots to absorb water and mineral elements, leading to inhibition of photosynthesis and transpiration ([10], [25]).
Tab. 2 - Plant growth and tolerance indices (Ti) in Populus × euramericana and P. deltoides plants cultivated in soil culture without (control) or with (Cd treat) Cd. Values are mean ± standard error. Means within a row followed by different letters are significantly different after Duncan’s test (P≤0.05). Two-way ANOVA (n=6) was applied to evaluate the coefficient of variation (CV, %), the clone effect, the Cd treatment effect (Cd treat) and their interaction (Clone × Cd). (*): p<0.05; (**): p<0.01; (***): p<0.001; (ns): p>0.05.
Parameter | Populus × euramericana | P. deltoides | CV (%) |
ANOVA P value | ||||
---|---|---|---|---|---|---|---|---|
Control | Cd treat |
Control | Cd treat |
Clone | Cd treat |
Clone × Cd |
||
Total biomass (g) | 7.86 ± 0.10 ab | 5.23 ± 0.06 c | 9.21 ± 0.50 a | 7.53 ± 0.69 b | 9.97 | ** | ** | ns |
Root mass (g) | 0.70 ± 0.07 a | 0.30 ± 0.02 b | 0.85 ± 0.07 a | 0.48 ± 0.04 b | 15.94 | * | *** | ns |
Shoot mass(g) | 1.81 ± 0.08 a | 1.45 ± 0.14 a | 1.72 ± 0.24 a | 1.33 ± 0.27 a | 21.81 | ns | ns | ns |
Leaf mass (g) | 5.36 ± 0.20 b | 3.47 ± 0.15 c | 6.65 ± 0.25 a | 5.72 ± 0.44 b | 9.21 | *** | ** | ns |
Shoot height (cm) | 27.00 ± 0.29 a | 27.90 ± 1.30 a | 23.00 ± 1.15 b | 15.53 ± 1.43 c | 8.42 | *** | * | ** |
Shoot diameter (mm) | 3.45 ± 0.07 b | 3.33 ± 0.07 b | 3.78 ± 0.07 a | 3.57 ± 0.06 b | 3.34 | ** | * | ns |
Number of leaves | 19.33 ± 0.33 a | 17.67 ± 0.33 a | 12.67 ± 0.33 b | 14.33 ± 0.88 b | 5.7 | *** | ns | * |
Leaf area (cm2) | 333.58 ± 27.62ab | 280.05 ± 15.22b | 344.18 ± 22.72ab | 368.25 ± 13.50 a | 10.75 | * | ns | ns |
Ti (%) | 100.00 ± 0.00a | 66.50 ± 0.38 c | 100.00 ± 0.00 a | 82.19 ± 7.95 b | 7.91 | ns | *** | ns |
To evaluate the ability of the studied poplar clones to tolerate Cd soil contamination, Ti was calculated (Tab. 2). Although Ti was negatively affected by Cd treatment in both clones, P. deltoides plants showed higher tolerance to applied Cd levels than Populus × euramericana. Estimated values of Ti (66.5 and 82.2 in Populus × euramericana and P. deltoides, respectively) were greater than 60 for both clones, indicating a high tolerance to the applied Cd levels ([26]).
In an attempt to identify a reliable indicator of good productivity in Cd stressed poplars, we correlated biomass production with some morphological, structural and physiological characteristics in Populus × euramericana and P. deltoides plants (Tab. 3). Significant positive correlation was recorded between biomass and the rate of photosynthesis, mass of leaves (per plant) and palisade tissue thickness in both poplar clones. In addition to the above characteristics, in the less tolerant Populus × euramericana clone, biomass production was significantly (positively or negatively) correlated with numerous other characteristics under Cd exposure. Recently, Aasamaa et al. ([1]) found that biomass production of Salix plants grown in a wastewater purification system correlated more strongly with photosynthetic characteristics than with plant water relations. According to the results of our experiments, the rate of photosynthesis might be considered to be a reliable, easy-to-measure indicator of high biomass production in poplars under heavy metal stress.
Tab. 3 - Pearson’s correlation coefficients between biomass production and morphological, leaf anatomical and physiological characteristics, and Cd accumulation in Populus × euramericana and P. deltoides plants under Cd exposure. (Ti): tolerance index; (Cdroots, Cdstem, Cdleaves): concentration of Cd in roots, stems and leaves, respectively; (BCFroots, BCFstem, BCFleaves): biological concentration factors for roots, stems and leaves, respectively; (Tfstem, Tfleaves): translocation factors for stem and leaves, respectively; (*): p<0.05; (**): p<0.01; (***): p<0.001
Parameters | Biomass Production | |
---|---|---|
P. × euramericana | P. deltoides | |
Root mass | 0.9148* | 0.6978 |
Shoot mass | 0.7271 | 0.9426** |
Number of leaves per plant | 0.8692* | -0.1947 |
Leaf area per plant | 0.6711 | -0.4792 |
Leaf mass per plant | 0.9798** | 0.9769** |
Ti | 0.9958*** | 0.8477* |
Lamina thickness | -0.3543 | 0.2070 |
Palisade/spongy tissue ratio | 0.9783** | -0.7756 |
Mesophyll thickness | -0.5589 | 0.2523 |
Adaxial epidermis thickness | 0.4731 | 0.0644 |
Abaxial epidermis thickness | -0.2922 | 0.7487 |
Palisade tissue thickness | 0.8414* | 0.8275* |
Spongy tissue thickness | -0.6422 | 0.4079 |
Main vein cross section area | -0.9636** | 0.4103 |
Main vein height/lamina thickness | -0.8966* | -0.2503 |
Main vein vascular bundle cross section area | -0.9252** | 0.5129 |
Main vein vascular bundle vessel lumen area | -0.8218* | 0.6048 |
Adaxial epidermis cell cross section area | -0.9566** | 0.6386 |
Palisade tissue cell cross section area | 0.9753** | -0.8145* |
Spongy tissue cell cross section area | -0.1765 | -0.3400 |
Abaxial epidermis cell cross section area | 0.8252* | -0.0726 |
Rate of photosynthesis (Pn) | 0.9952*** | 0.8486* |
Rate of transpiration (Tr) | 0.9830*** | 0.7723 |
Stomatal conductance (gs) | 0.9862*** | 0.5887 |
Intercellular CO2 concentration (Ci) | -0.8919* | -0.3199 |
Chlorophyll a | 0.7859 | 0.7369 |
Chlorophyll b | 0.9867*** | 0.7128 |
Chlorophyll a+b | 0.8954* | 0.7043 |
Carotenoids | 0.6920 | 0.7188 |
Chlorophyll a/b | -0.9477** | -0.6709 |
Concentration of proline | 0.9598** | 0.1875 |
Activity of nitrate reductase | 0.9697** | 0.3073 |
Cdleaves | -0.9933*** | -0.8045 |
Cdroots | -0.9969*** | -0.6228 |
Cdstem | -0.9775** | -0.7247 |
BCFleaves | 0.9901*** | 0.6438 |
BCFroots | 0.9935*** | 0.7052 |
BCFstem | 0.9757** | 0.6934 |
Tfleaves | 0.9224** | -0.7221 |
Tfstem | 0.9565** | -0.7647 |
Leaf anatomical characteristics
In plants exposed to heavy metals, alterations to leaf structural parameters might affect photosynthesis, and thus biomass production and plant performance under stress conditions. The structural characteristics of leaves in P. deltoides and P. × euramericana plants cultivated with or without moderate levels of Cd in the soil are presented in Tab. 4. Although leaf lamina thickness did not differ significantly among poplar clones, values measured for relative mesophyll thickness, main vein characteristics (cross section area, vascular bundle and vessel lumen area, index), and the cross section area of spongy tissue and abaxial epidermis cells were considerably lower in Populus × euramericana than P. deltoides plants grown under control conditions (Fig. 1). Cd did not considerably affect most of the studied leaf structural parameters, with the exception of the cross section area of adaxial epidermis cells and vessel lumen area in Populus × euramericana, and the cross section area of palisade tissue cells in both poplar clones. The thickness of spongy tissue was greater than the thickness of palisade tissue in both clones, regardless of Cd exposure. These findings are in agreement with results obtained for other poplar genotypes from different taxa ([3]).
Tab. 4 - Leaf anatomical parameters in Populus × euramericana and P. deltoides plants cultivated in soil culture without (control) or with (Cd treat) Cd. Values are mean ± standard error. Means within a row followed by different letters are significantly different after Duncan’s test (P≤0.05. Two-way ANOVA was applied to evaluate coefficient of variation (CV, %), the clone effect, the Cd treatment effect (Cd treat) and their interaction (Clone × Cd). (*): p<0.05; (**): p<0.01; (***): p<0.001; (ns): p>0.05.
Group | Parameter | Populus × euramericana | P. deltoides | CV (%) |
ANOVA P value | ||||
---|---|---|---|---|---|---|---|---|---|
Control | Cd treat |
Control | Cd treat |
Clone | Cd treat |
Clone × Cd |
|||
- | Lamina thickness (µm) | 141.70 ± 6.57 a | 143.40 ± 4.15 a | 158.90 ± 5.19 a | 148.80 ± 6.49 a | 8.58 | ns | ns | ns |
Relative proportion (%) of |
Mesophyll thickness | 80.60 ± 1.29 b | 83.40 ± 0.99 ab | 86.10 ± 0.48 a | 85.70 ± 0.56 a | 2.38 | *** | ns | ns |
Adaxial epidermis thickness | 9.70 ± 0.55 a | 8.70 ± 0.43 ab | 7.30 ± 0.27 c | 7.60 ± 0.39 bc | 11.36 | *** | ns | ns | |
Abaxial epidermis thickness | 7.50 ± 0.20 a | 7.50 ± 0.21 a | 6.60 ± 0.26 b | 6.30 ± 0.21 b | 7.19 | *** | ns | ns | |
Palisade tissue thickness | 37.60 ± 0.64 ab | 35.00 ± 1.00 b | 38.40 ± 0.83 ab | 38.70 ± 1.52 a | 6.27 | * | ns | ns | |
Spongy tissue thickness | 43.60 ± 2.10 a | 45.20 ± 1.26 a | 46.40 ± 1.07 a | 46.50 ± 1.92 a | 8.10 | ns | ns | ns | |
- | Palisade/spongy tissue ratio | 0.90 ± 0.05 a | 0.80 ± 0.04 a | 0.80 ± 0.03 a | 0.80 ± 0.06 a | 13.57 | ns | ns | ns |
Main vein | Cross section area (mm2) | 0.24 ± 0.03 b | 0.38 ± 0.03 b | 0.78 ± 0.10 a | 0.71 ± 0.09 a | 29.78 | *** | ns | ns |
Index | 4.40 ± 0.32 b | 5.00 ± 0.25 b | 6.30 ± 0.43 a | 6.80 ± 0.48 a | 15.44 | *** | ns | ns | |
Vascular bundle cross section area (mm2) | 0.10 ± 0.01 b | 0.14 ± 0.01 b | 0.28 ± 0.03 a | 0.26 ± 0.03 a | 24.73 | *** | ns | ns | |
Vascular bundle vessel area (µm2) |
417.70 ± 9.68 b | 352.00 ± 32.79 c | 568.70 ± 8.77 a | 526.80 ± 10.78 a | 8.85 | *** | ns | * | |
Cell cross-section area |
Adaxial epidermis (µm2) | 258.30 ± 11.26 b | 318.50 ± 12.18 a | 229.90 ± 3.13 c | 227.50 ± 3.65 c | 7.47 | *** | ** | ** |
Palisade tissue (µm2) | 285.80 ± 3.91 b | 261.40 ± 0.81 c | 291.00 ± 4.13 b | 313.40 ± 3.43 a | 2.59 | *** | ns | *** | |
Spongy tissue (µm2) | 205.80 ± 3.70 b | 206.20 ± 5.29 b | 269.80 ± 1.16 a | 274.60 ± 2.98 a | 0.55 | *** | ns | ns | |
Abaxial epidermis (µm2) | 213.40 ± 4.18 b | 204.10 ± 2.09 b | 258.40 ± 1.14 a | 258.60 ± 3.65 a | 2.89 | *** | ns | ns |
Fig. 1 - Effect of Cd on the main vein cross section of Populus × euramericana (a, c) and P. deltoides (b, d) plants grown in soil culture for six weeks. (a, b): control; (c, d): Cd treatment.
Well documented relationships between structural and functional characteristics of leaf mesophyll indicate that changes in internal leaf morphology affect the photosynthetic process ([18]). In the present study, palisade cell size was considerably but differentially changed in both clones under Cd exposure: palisade cell size was decreased in Populus × euramericana but increased in P. deltoides. In a hydroponic experiment with Populus × euramericana (clone I-214) and Salix fragilis L., Luković et al. ([23]) reported no significant effect of Cd treatment on the characteristics of photosynthetic tissue in the poplar clone. However, the percentage of palisade tissue, palisade thickness and cell area increased in Salix fragilis exposed to Cd. In our study, the decrease of palisade cell area observed in the Populus × euramericana clone is in agreement with the findings of Djebali et al. ([12]), who reported a reduction of mesophyll cell size, reduced intercellular spaces, and severe alterations of chloroplast fine structure in tomato upon Cd exposure. Furthermore, Cd treatment affected adaxial epidermis cell size only in the Populus × euramericana clone. These results are in agreement with the effect of Cd on dermal tissue in poplar ([23]).
Physiological responses: gas exchange parameters, concentration of photosynthetic pigments, proline accumulation, and NR activity
Undisturbed photosynthesis is necessary for continuous biomass production in plants exposed to heavy metals. Thus, photosynthetic activity is considered an important indicator of plant tolerance to heavy metals, which results from efficient mechanisms involved in the prevention of metal induced toxic effects ([39]). Means of light-saturated net assimilation at ambient CO2 levels (Pn) and the rate of transpiration (Tr) of poplar clones investigated in the present study can be found in Pajević et al. ([30]), who compared the photosynthetic activity of several poplar and willow clones exposed to various pollutants applied separately or in a cocktail. In the present study, relative changes of Pn, Tr, stomatal conductance (gs) and intercellular CO2 concentration (Ci) were calculated (with respect to control) for P. deltoides and P. × euramericana plants exposed to Cd in the soil, in order to compare their photosynthetic performance with respect to phytoextraction potential. Parameters related to gas exchange were further correlated with biomass production (Fig. 2, Tab. 3).
Fig. 2 - Changes of the net photosynthesis (Pn), transpiration (Tr), stomatal conductance (gs) and intercellular CO2 concentration (Ci) in Populus × euramericana and P. deltoides plants exposed to Cd, with respect to the control (%). Significance of differences between control and Cd-treated plants according to Student’s t-test are indicated as (*): p<0.05; (**): p<0.01; (***): p<0.001.
The rate of photosynthesis was considerably decreased in Cd-exposed plants in both clones (25.5 and 6.4% in Populus × euramericana and P. deltoides respectively). Similarly, the rate of transpiration was also decreased in both genotypes, but to a greater extent in P. deltoides (25.8%) than P. × euramericana (18.1%). However, a considerable decrease of stomatal conductance (gs) was observed only in Cd-exposed Populus × euramericana clone. The decrease in gs observed in the present study might be related to stomatal closure or decrease of the stomatal aperture size, with concomitant limitations on the diffusion of water vapor and gasses (CO2 and O2). A significant increase of intercellular CO2 concentrations, accompanied with a reduction of photosynthetic activity, was observed in both genotypes, while gs reduction occurred only in Populus × euramericana. Considering the significant increase in intercellular CO2 concentration in both poplar clones, but differential gs response following Cd exposure, our results suggest different mechanisms (stomatal and/or mesophyll impairments) are involved in Cd-induced reduction of photosynthetic activity in the studied poplars. Reduction of net photosynthetic rate due to mesophyll limitations has been observed in other woody (Salicaceae - [40]) and herbaceous ([38]) species exposed to Cd. However, the increase of intercellular CO2 levels observed in both clones (regardless of gs) suggests inhibition of Calvin cycle enzymes rather than PSII activity in the tested clones ([30]). Previous investigations reported negative effects of Cd on various enzymes of the Calvin cycle, for example ribulose-1.5-bisphosphate carboxylase/oxygenase ([10]), glyceraldehyde-3-phosphate dehydrogenase (GAPDH) and 3-phosphoglyceric acid kinase ([9]). These conclusions may also apply for the poplars used in the present study, due to the discrepancy between anatomical characteristic and expected photosynthetic activity. Namely, the decrease in palisade cell size observed here in the Populus × euramericana clone was expected to positively correlate with photosynthetic rate, since a decrease of mesophyll cell size results in an increase in area available for carbon dioxide diffusion and might contribute to higher photosynthetic efficiency ([18]).
In the present study, Cd significantly decreased the rate of transpiration in P. deltoides plants, without affecting stomatal conductance (Fig. 2), suggesting that the occurrence of structural changes in roots was triggered by Cd metal accumulation. Development of physical barriers in roots intended to restrict the entry of Cd into the xylem, and a concomitant decrease of Cd accumulation in shoot tissues, has been observed in various plant species ([25]). However, these changes might affect water uptake and transport as well as transpiration, due to the relationship between root permeability to water and the rate of transpiration. In Populus × euramericana clone, Cd treatment decreased both transpiration and stomatal conductance. Stomatal closure and alteration of stomatal conductance might be the result of the interaction of Cd at the guard cell level, or a consequence of toxic effects in other plant tissues, leading to impairment of water balance and subsequent stomatal closure ([32]). Cd-induced alterations of stomatal conductance and transpiration have been observed in other plant species ([33], [22]). Since stomatal conductance was not found to be reduced by Cd exposure in the P. deltoides clone (in contrast to the Populus × euramericana clone which displayed a considerably lower gs - Fig. 2), unlimited diffusion of CO2 to the mesophyll cells is to be expected.
Translocation of Cd is related to energy-dependent uptake from the medium to the xylem, and transfer from the xylem to the leaves, which is driven mainly by transpiration ([21]). Although transpiration was higher in P. deltoides than Populus × euramericana, it was reduced to a greater extent in P. deltoides following Cd exposure, and probably contributed to lower accumulation of Cd in the leaves of P. deltoides vs. P. × euramericana (Tab. 5). However, Akhter & Macfie ([2]) attributed greater importance to species-specific factors regulating internal plant distribution of Cd over transpiration rate, in Cd translocation to aboveground tissues.
Tab. 5 - Cadmium accumulation and translocation in Populus × euramericana and P. deltoides plants cultivated in soil culture without (control) or with (Cd treat) Cd. Values are mean ± standard error. Means within a row followed by different letters are significantly different after Duncan’s test (P≤0.05). Two-way ANOVA (n=3) was applied to evaluate coefficient of variation (CV, %), the clone effect, the Cd treatment effect (CD treat) and their interaction (Clone × Cd). (Cdroots, Cdstem, Cdleaves): concentration of Cd in roots, stems and leaves, respectively; (BCFroots, BCFstem, BCFleaves): biological concentration factors for roots, stems and leaves, respectively; (Tfstem, Tfleaves): translocation factors for stem and leaves, respectively; (*): p<0.05; (**): p<0.01; (***): p<0.001; (ns): p>0.05.
Parameter | Populus × euramericana | P. deltoides | CV (%) |
ANOVA P values | ||||
---|---|---|---|---|---|---|---|---|
Control | Cd treat |
Control | Cd treat |
Clone | Cd treat |
Clone × Cd |
||
Cdroots (mg kg-1) | 1.66 ± 0.02 d | 10.13 ± 0.06 a | 3.54 ± 0.08 c | 5.19 ± 0.10 b | 2.52 | *** | *** | *** |
Cdstem (mg kg-1) | 1.31 ± 0.14 c | 3.17 ± 0.04 b | 1.35 ± 0.3 c | 4.43 ± 0.06 a | 5.4 | *** | *** | *** |
Cdleaves (mg kg-1) | 1.03 ± 0.06 c | 4.16 ± 0.02 a | 0.77 ± 0.10 c | 1.49 ± 0.10 b | 7.6 | *** | *** | *** |
BCFroots | 4.76 ± 0.06 b | 1.24 ± 0.01 c | 10.10 ± 0.23 a | 0.64 ± 0.01 d | 4.99 | *** | *** | *** |
BCFstem | 3.73 ± 0.40 a | 0.39 ± 0.00 b | 3.86 ± 0.08 a | 0.54 ± 0.01 b | 16.73 | ns | *** | ns |
BCFleaves | 2.95 ± 0.18 a | 0.51 ± 0.00 c | 2.21 ± 0.30 b | 0.18 ± 0.01 c | 20.82 | * | *** | ns |
Tfstem | 0.78 ± 0.08 a | 0.31 ± 0.01 b | 0.38 ± 0.02 b | 0.85 ± 0.03 a | 12.8 | ns | ns | *** |
Tfleaves | 0.62 ± 0.05 a | 0.41 ± 0.00 b | 0.22 ± 0.03 c | 0.29 ± 0.02 c | 14.37 | *** | ns | ** |
The most readily observable symptom of perturbation of chlorophyll metabolism in plants experiencing the toxic effect of heavy metals is leaf chlorosis caused by decreased chlorophyll concentration due to enhanced degradation or reduced biosynthesis. In our experiment, chlorosis was not observed in young leaves of plants exposed to Cd. Generally, higher concentrations of chlorophylls were recorded in P. deltoides than P. × euramericana clone, independent of Cd exposure (Tab. 6). Although Cd negatively affected the concentration of chlorophylls in both poplar clones, values were reduced to a greater extent in Populus × euramericana than P. deltoides: specifically, concentrations of chlorophyll a, b and total chlorophylls decreased by 17.6%, 34.9% and 22.9% in Populus × euramericana, and by 16.2%, 31.3% and 20.8% P. deltoides, respectively as compared to control. These observations are in agreement with results obtained for other poplar clones cultivated in the presence of Cd, including clones of Populus × euramericana, Populus × generosa, Populus × canadensis, Populus deltoides × P. nigra, P. deltoides, P. nigra, P. alba and P. trichocarpa ([33], [43]).
Tab. 6 - Photosynthetic pigments concentration, activity of nitrate reductase (NR), and concentration of proline in leaves of Populus × euramericana and P. deltoides clones cultivated in soil culture without (control) or with (Cd treat) Cd. Values are mean ± standard error. Means within a row followed by different letters are significantly different after Duncan’s test (P≤0.05). Two-way ANOVA (n=5) was applied to evaluate the coefficient of variation (CV, %), the clone effect, the Cd treatment effect and their interaction (Clone × Cd). (*): p<0.05; (**): p<0.01; (***): p<0.001; (ns): p>0.05.
Parameters | Populus × euramericana | P. deltoides | CV (%) |
ANOVA P values | ||||
---|---|---|---|---|---|---|---|---|
Control | Cd treat |
Control | Cd treat |
Clone | Cd treat |
Clone × Cd |
||
Chlorophyll a (mg g-1) | 11.11 ± 0.27 b | 9.16 ± 0.63 c | 13.48 ± 0.10 a | 11.30 ± 0.02 b | 5.32 | *** | *** | ns |
Chlorophyll b (mg g-1) | 4.07 ± 0.03 b | 2.65 ± 0.09 d | 4.95 ± 0.02 a | 3.40 ± 0.12 c | 3.55 | *** | *** | ns |
Chlorophyll a+b (mg g-1) | 15.26 ± 0.23 b | 11.77 ± 0.73 c | 18.46 ± 0.13 a | 14.61 ± 0.14 b | 4.55 | *** | *** | ns |
Chlorophyll a/b | 2.73 ± 0.09 b | 3.45 ± 0.12 a | 2.72 ± 0.01 b | 3.33 ± 0.11 a | 5.16 | ns | *** | ns |
Carotenoids (mg g-1) | 3.26 ± 0.12 a | 2.79 ± 0.19 b | 3.62 ± 0.04 a | 3.33 ± 0.07 a | 6.39 | ** | * | ns |
NR (µM NO2- g-1 h-1) | 0.21 ± 0.01 a | 0.13 ± 0.01 b | 0.14 ± 0.01 b | 0.15 ± 0.00 b | 9.44 | * | *** | *** |
Proline (µg g-1) | 21.84 ± 1.29 a | 12.49 ± 0.80 b | 14.4 ± 0.93 b | 14.41 ± 0.38 b | 9.98 | * | *** | *** |
In our study, chlorophyll concentration was determined in young leaves emerged from plants exposed to Cd, indicating inhibition of biosynthesis rather than stimulated degradation in Populus × euramericana and P. deltoides. It has been suggested that Cd alters chlorophyll biosynthesis through disturbance of glutathione availability, inhibition of δ-aminolevulinic acid dehydratase activity, disturbance of protochlorophyllide reductase function, interference with the uptake of other divalent cations (including calcium, magnesium and iron), or substitution of Cd for Mg in the chlorophyll molecule ([31]). In our previous experiments on hydroponically cultivated poplars (P. deltoides cl. B-81, (P. nigra × P. maximowitzii) × P. nigra var. Italica cl. 9111/93, and P. deltoides × P. nigra cl. M-1) exposed to various Cd concentrations, a considerable decrease in Fe concentration in aboveground plant organs (i.e., young leaves and shoots) was detected ([28]). Therefore, we assume that the decreased chlorophyll content observed in Populus × euramericana and P. deltoides plants exposed to Cd might be, at least partially, ascribed to Fe deficiency. Although Cd treatment considerably reduced the concentration of chlorophyll a and b in both Populus × euramericana and P. deltoides plants, the increase of chlorophyll a/b ratio observed suggests a greater reduction of chlorophyll b concentration than chlorophyll a. A favored decrease of chlorophyll b concentration could arise from either decreased conversion of chlorophyll a to chlorophyll b, or increased reversion of chlorophyll b to chlorophyll a ([13]). Considering that the light-harvesting chlorophyll-protein complex of photosystem II contains the majority of chlorophyll b ([20]),the obtained results suggest that Cd causes a decrease in photosystem II (PSII) light harvesting complexes related to reaction centers ([22]).
The presence of Cd in the growth substrate considerably decreased the concentration of carotenoids in Populus × euramericana clones (by 14.4% vs. control - Tab. 6), in contrast with the results of Lunáčková et al. ([24]), who found no influence of Cd treatment on total chlorophyll and carotenoid content in another Populus × euramericana clone. The decreased carotenoid content observed in plant species during Cd toxicity has been ascribed to a protective mechanism that preserves chlorophyll pools at the expense of carotenoids, due to overproduction of reactive oxygen species (ROS - [38]). Therefore, the increase in carotenoid concentrations observed under heavy metal stress contributes to cell protection against oxidative damage ([8]). Nevertheless, the concentration of carotenoids was not considerably changed in P. deltoides plants.
NR activity is considered to be the rate-limiting step in nitrogen (N) assimilation in plants, due to the necessary reduction in the pathway nitrate - nitrite - ammonium prior to N incorporation into organic compounds. Poplar clones differ in NR activity due to their genetically determined ability to synthesize NR in response to nitrate ions. In our study, NR activity in leaves was considerably affected in Populus × euramericana, while in P. deltoides no significant changes were induced by the presence of Cd in the soil (Tab. 6). Significant changes of NR activity in both roots and leaves of Cd exposed plants with respect to the control were also found in the experiment with three poplar cultivars ([28]). This discrepancy in NR enzyme activity in the leaves of Populus × euramericana clones could be ascribed to the higher Cd concentrations measured in P. × euramericana leaves in our study (Tab. 5). Since the availability of nitrate ions, along with other mechanisms, enhances NR activity, the decreased NR activity observed in Populus × euramericana leaves may be, at least partly, attributed to the depletion of a continuous supply of nitrates through the xylem via the transpiration stream ([19]). Furthermore, NR can also catalyze the reduction of nitrites to nitric oxide, a cell signaling molecule which plays an important role in plant responses to cadmium stress ([15]). Lowered NR activity in P. × euramericana plants suggests Cd-induced diminution of nitrogen (N) delivery for assimilation into plant organic compounds. A sufficient N supply positively affects the tolerance of plants against Cd toxicity through increased biomass production (by improved photosynthetic capacity), soluble protein content, and activity of antioxidant enzymes, concomitantly with reduced Cd accumulation in plant organs ([34], [27]). Recent experiments indicate that nitrogen efficiently alleviates Cd toxicity in poplars, by unblocking the chlorophyll synthesis pathway and preventing the occurrence of toxicity symptoms ([43]). Higher NR activity accompanied by high N content and proline accumulation has been reported in Cd-tolerant plants ([4]). Therefore, N metabolism is pivotal to the response and acclimation of plants to heavy metal stress, because plant tolerance depends on the synthesis of diverse nitrogen containing metabolites, such as specific amino acids (proline, histidine), peptides (glutathione, phytochelatins), amines and mugineic acids ([35]). In our study, proline concentrations were higher in Populus × euramericana than P. deltoides plants in the control treatment (Tab. 6). However, a significant decrease in proline content (from 21.84 to 12.49 µg g-1 in control and Cd treatment, respectively) was observed only in Populus × euramericana clones under Cd exposure, consistent with previous investigations on poplars ([29]). This observed decrease in proline content in Populus × euramericana leaves might be explained by Cd-induced N deficiency (lower NR activity), with consequent enhancement of proline degradation over synthesis. Increased proline synthesis under Cd exposure has been related to increased tolerance of various plant species, including poplars ([35], [17]). Thus, we suppose that the unaffected proline content observed in P. deltoides plants under Cd stress indicates undisturbed N metabolism and contributes to the higher Cd tolerance (according to Ti - Tab. 2) of the P. deltoides clone versus P. × euramericana, along with other mechanisms.
Phytoremediation potential: Cd accumulation, translocation and BCF
The concentration and distribution of Cd in plant organs was noticeably different in P. deltoides and P. × euramericana plants cultivated in Cd-enriched soil (Tab. 5). The Populus × euramericana clone accumulated considerably more Cd in roots and leaves (10.13 and 4.16 mg kg-1, respectively) than the P. deltoides clone (5.19 and 1.49 mg kg-1, respectively). However, the Populus × euramericana clone accumulated significantly less Cd in stems than P. deltoides (3.17 and 4.43 mg kg-1, respectively). Previous studies have indicated that the transpiration stream is a driving force in translocation of Cd and its accumulation in leaves in various plant species ([40], [21]), and our results are consistent with these reports. Although P. deltoides displayed a higher rate of transpiration than Populus × euramericana (regardless of Cd treatment), these values were significantly lower in treated plants in both studied clones by 25.8 and 18.1%. Consequently, this lower transpiration reduced Cd accumulation in leaves to a greater extent in P. deltoides, resulting in higher Cd accumulation in stems, probably due to Cd retention ([21]). Based on a study addressing species-specific relationships between cadmium translocation and transpiration in various plant species, Akhter & Macfie ([2]) concluded that Cd accumulation in plants depends on multiple factors, including bulk flow through transpiration, Cd concentration in the growth substrate and a plant-specific factor. The results obtained in the present study suggest that both clones employ an exclusion strategy, by retaining the highest amounts of Cd in the roots and low translocation into aerial organs. However, P. deltoides plants accumulated more Cd in stems than P. × euramericana, suggesting its potential not only for phytostabilization, but also for phytoextraction projects.
Bioconcentration factors (BCF) calculated for aboveground (leaves, stem) plant organs and roots (Tab. 5) showed a narrow range of variation (0.18-1.24). BCF values decreased in both poplar clones with an increase in Cd concentration in the growth substrate. The tested poplar clones differed significantly only by root BCF values. The obtained results are in agreement with previous studies indicating higher BCF values in roots than aboveground organs in various poplar species, due to their differential ability to accumulate Cd ([17]).
Translocation of Cd from roots to shoots and leaves was estimated by Tfstem and TFleaves (Tab. 5). The clones showed specific patterns of Cd translocation in the treated plants. Under Cd exposure, Tf for both leaves and stems was considerably reduced in Populus × euramericana plants with respect to control. However, TFleaves was unaffected, and Tfstem significantly increased in P. deltoides plants exposed to Cd with respect to control. Furthermore, Tfleaves was lower, while Tfstem was higher in clone P. deltoides than P. × euramericana, which also exhibited higher tolerance (based on Ti) than the latter clone. These results are in agreement with the findings of Vassilev et al. ([40]), who conducted an experiment with two willow species grown hydroponically under increasing Cd concentrations and revealed greater allocation of Cd to leaves in less-tolerant species. The obtained results suggest that different strategies for metal distribution are employed by the tested poplars under Cd stress. Following treatment, 58.0 and 46.7% of the total Cd content was accumulated in the roots of Populus × euramericana and P. deltoides, respectively, with the remainder in the stems (18.2 and 39.9%) and leaves (23.8 and 13.4%).
Conclusion
Efficient decontamination of Cd polluted soils through phytoremediation requires fast growing tree species with high Cd tolerance and the ability to preferentially accumulate Cd in aboveground plant organs. The higher accumulation of Cd observed in stems rather than leaves of poplar plants is advantageous for prevention of secondary pollution of the environment due to autumnal shedding and spreading of Cd enriched leaves. In the present study, morphological, anatomical and physiological data suggest that Populus × euramericana and P. deltoides clones respond differently to Cd exposure. According to our results, P. deltoides plants exposed to Cd display higher tolerance, undisturbed N metabolism and increased Cd translocation to stems versus P. × euramericana. Thus, this P. deltoides clone may have potential for both phytostabilization and phytoextraction projects. The screening protocol outlined in the present study is based on physiological and morphological plant characteristics, and may represent an efficient method for evaluating crop performance and tolerance to heavy metal stress.
Acknowledgements
This study was supported by the Ministry of Education, Science and Technological Development of the Republic of Serbia. All authors have been involved in designing the experiment, cultivation of plants, measurement of studied parameters, processing and discussion of obtained results, and writing the manuscript. The authors have no conflicts of interest. The authors wish to express their appreciation to Dr. Edward Petri, for editing this manuscript for English language and style. We are also grateful to both anonymous reviewers for valuable and constructive suggestions and comments.
References
Gscholar
CrossRef | Gscholar
CrossRef | Gscholar
Gscholar
Gscholar
Gscholar
Gscholar
Authors’ Info
Authors’ Affiliation
Lana Zorić
Ivana Cvetković
Slobodanka Pajević
Milan Borišev
Department of Biology and Ecology, Faculty of Sciences, Trg D. Obradovića 2, Novi Sad (Republic of Serbia)
Andrej Pilipović
Institute of Lowland Forestry and Environment, Antona Cehova 13, 21000 Novi Sad (Republic of Serbia)
Corresponding author
Paper Info
Citation
Nikolić N, Zorić L, Cvetković I, Pajević S, Borišev M, Orlović S, Pilipović A (2017). Assessment of cadmium tolerance and phytoextraction ability in young Populus deltoides L. and Populus × euramericana plants through morpho-anatomical and physiological responses to growth in cadmium enriched soil. iForest 10: 635-644. - doi: 10.3832/ifor2165-010
Academic Editor
Claudia Cocozza
Paper history
Received: Jul 06, 2016
Accepted: Mar 30, 2017
First online: Jun 01, 2017
Publication Date: Jun 30, 2017
Publication Time: 2.10 months
Copyright Information
© SISEF - The Italian Society of Silviculture and Forest Ecology 2017
Open Access
This article is distributed under the terms of the Creative Commons Attribution-Non Commercial 4.0 International (https://creativecommons.org/licenses/by-nc/4.0/), which permits unrestricted use, distribution, and reproduction in any medium, provided you give appropriate credit to the original author(s) and the source, provide a link to the Creative Commons license, and indicate if changes were made.
Web Metrics
Breakdown by View Type
Article Usage
Total Article Views: 42687
(from publication date up to now)
Breakdown by View Type
HTML Page Views: 35339
Abstract Page Views: 2680
PDF Downloads: 3593
Citation/Reference Downloads: 38
XML Downloads: 1037
Web Metrics
Days since publication: 2613
Overall contacts: 42687
Avg. contacts per week: 114.35
Article Citations
Article citations are based on data periodically collected from the Clarivate Web of Science web site
(last update: Feb 2023)
Total number of cites (since 2017): 23
Average cites per year: 3.29
Publication Metrics
by Dimensions ©
Articles citing this article
List of the papers citing this article based on CrossRef Cited-by.
Related Contents
iForest Similar Articles
Short Communications
A quick screening to assess the phytoextraction potential of cadmium and copper in Quercus pubescens plantlets
vol. 10, pp. 93-98 (online: 13 October 2016)
Research Articles
Analysis of biometric, physiological, and biochemical traits to evaluate the cadmium phytoremediation ability of eucalypt plants under hydroponics
vol. 10, pp. 416-421 (online: 24 March 2017)
Research Articles
Links between phenology and ecophysiology in a European beech forest
vol. 8, pp. 438-447 (online: 15 December 2014)
Research Articles
Effect of cadmium (Cd) and lead (Pb) soil contamination on the development of Hymenoscyphus fraxineus on Fraxinus excelsior and F. angustifolia seedlings
vol. 16, pp. 307-313 (online: 09 November 2023)
Research Articles
Can species Cedrela fissilis Vell. be used in sites contaminated with toxic aluminum and cadmium metals?
vol. 14, pp. 508-516 (online: 11 November 2021)
Research Articles
Drought tolerance in cork oak is associated with low leaf stomatal and hydraulic conductances
vol. 11, pp. 728-733 (online: 06 November 2018)
Research Articles
Mapping Cadmium distribution in roots of Salicaceae through scanning electron microscopy with x-ray microanalysis
vol. 4, pp. 113-120 (online: 01 June 2011)
Research Articles
Tolerance to heavy metal stress in seedlings of three pine species from contrasting environmental conditions in Chile
vol. 9, pp. 937-945 (online: 12 August 2016)
Research Articles
Effect of salt and drought on growth, physiological and biochemical responses of two Tamarix species
vol. 8, pp. 772-779 (online: 25 March 2015)
Research Articles
Preliminary results of the tolerance to inorganic contaminants and phytoextraction potential of twelve ornamental shrub species tested on an experimental contaminated site
vol. 11, pp. 442-448 (online: 18 June 2018)
iForest Database Search
Search By Author
Search By Keyword
Google Scholar Search
Citing Articles
Search By Author
Search By Keywords
PubMed Search
Search By Author
Search By Keyword