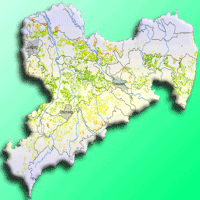
Linking biomass production in short rotation coppice with soil protection and nature conservation
iForest - Biogeosciences and Forestry, Volume 7, Issue 6, Pages 353-362 (2014)
doi: https://doi.org/10.3832/ifor1168-007
Published: May 19, 2014 - Copyright © 2014 SISEF
Research Articles
Collection/Special Issue: RegioResources21
Spatial information and participation of socio-ecological systems: experiences, tools and lessons learned for land-use planning
Guest Editors: Daniele La Rosa, Carsten Lorz, Hannes Jochen König, Christine Fürst
Abstract
Biomass from short rotation coppice (SRC) plantations has attracted widespread attention as a component of new sustainable energy concepts. Nevertheless, as yet the surface area of SRC plantations in Europe is relatively low compared to other biomass producing land-use systems. This is somewhat incomprehensible because it has been shown that SRC systems also offer distinct ecological benefits. Therefore, greater consideration of the related ecosystem services should be incorporated into land-use planning processes. Presented in this study is a conceptual framework for the integration of soil protection and nature conservation into the spatial prioritization of areas suitable for SRC. This approach includes the development of a site-specific yield model and the identification and classification of criteria and indicators for both soil protection and nature conservation. The basic concept for planning procedures was established and tested for the State of Saxony (Germany). Existing constraints were identified, and could be attributed mainly to the availability of adequate information at different spatial scales. The regional-scale study emphasized the considerable biomass potential of SRC and the related synergy effects for soil protection and nature conservation. Future work should focus on the local (district, farm) and catchment scale and attempt to integrate additional aspects such as hydrological ecosystem services and carbon sequestration.
Keywords
Short Rotation Coppice, Yield Estimation, Soil Protection, Nature Conservation, Land Use Planning
Introduction
A goal of the European Union states that up to the year 2020 the contribution of renewable sources of energy to total primary energy consumption should increase to a proportion of 20 % ([16]). Plantations of fast growing tree species with short rotations and subject to coppice management (SRC), grown on arable land for the purposes of heat or combined heat and power production are seen as a very effective means to achieve increased environmental benefits. In comparison to other biomass production systems, such as oil seed rape for oil production or maize for gasification, SRC is characterized by a positive greenhouse gas balance ([22]) and therefore low CO2 emission reduction costs ([35]). Furthermore, SRC has positive effects on soil ecology ([27]) and water quality ([39]), and increased biodiversity ([34], [19]). The aforementioned benefits provided to society by SRC may be summarized under the heading of ecosystem services ([8]). It is also suggested from an economic perspective that lignocellulose supply systems like SRC should be stimulated in order to develop the high inherent potential for the production of bioenergy within Europe ([4], [10]). At present, however, the area of SRC in Europe with around 50 000 ha remains rather low in comparison with other biomass systems, e.g., maize silage production for biogas on 700 000 ha in Europe ([12]). There are many possible reasons for this, for example:
- low availability of arable land for the establishment of SRC systems due to competition with food production;
- biased market situation brought about by counterproductive subsidies in the agricultural biomass production sector ([35], [36], [4]);
- few best practice examples sufficiently highlighting the benefits of SRC ([21]);
- prejudices on the part of environmentalists and the public against land-use alterations ([32]);
- legislative restrictions placed on landowners;
- reduction of landowners’ opportunities to adjust agricultural production in reaction to short-term market developments as SRC is a long term commitment;
- a high proportion of arable land is merely leased and not owned;
- no adequate consideration of SRC in land-use planning procedures to date.
Some of the obstacles mentioned may be reduced only through modifications to the political framework conditions. However, existing knowledge with regard to SRC must also be better communicated, in order to promote broader acceptance amongst the various stakeholder groups ([42]).
The authors hypothesized that an integrated (“holistic”) planning approach would be useful to develop greater practical evidence of SRC. In a first step, the potential effects of biomass production in SRC with regard to nature conservation and soil protection should be recognized as being important components of ecosystem services ([8]). Therefore, existing synergy effects should be depicted and assessed, e.g., within planning processes or formulation of subsidy programs, as should potential trade-offs.
The aim of the study was to develop a conceptual framework for the planning of SRC at a regional scale in the Federal State of Saxony (Germany). This included: (1) a conceptual model to predict SRC biomass production on the basis of available soil and climate data; (2) defining priority and exclusion areas in relation to soil protection; (3) defining priority and exclusion areas in relation to species and habitat protection and legally protected areas; (4) application of the results within a GIS environment.
Materials and methods
Study area
The Federal State of Saxony is located in eastern Germany. It covers a total area of 18415 km2. The state can be classified into three main geographical regions along a site gradient running from north to south, namely the lowlands, the hilly region and the low mountain range region (Tab. 1).
Tab. 1 - Main geographic regions of Saxony, and the corresponding climate and soil characteristics.
Geographic region |
Altitude [m asl] |
Mean annual air temperature [°C] |
Mean annual precipitation [mm] |
Predominant soil type |
---|---|---|---|---|
Lowlands | 80 - 200 | 8.0 - 9.0 | 550 - 650 | Sandy Cambisols |
Hilly region | 100 - 400 | 7.5 - 9.0 | 480 - 800 | Luvisols |
Low mountain range | 400 - 1215 | 4.0 - 8.0 | 700 - >1 000 | Sceletic Cambisols |
Agriculture is the predominant land use in the Saxony lowlands, particularly in the more fertile areas located at the transition to the hilly loess region. There are also large areas to the north and northeast of the lowlands that have been affected by lignite open pit mining and subsequent reclamation. Forest cover amounts to 27 % of the lowland surface area.
The hilly region of Saxony is a typically agrarian landscape with large open fields featuring only very few structural elements such as trees and shrubs. Forests cover only 12 % of the state’s hilly region. Due to their fine texture and the intensive farming, the soils are often prone to erosion by wind and water.
Forest cover in the low mountain range (Ore Mountains) is 42 % of the surface area ([40]). Arable land uses still predominate in the lower parts, but with increasing altitude grasslands become dominant and in the upper parts forest. Most of Saxony’s rivers spring from the Ore Mountains, making the region extraordinarily important for public water supply.
Estimation of the site-specific production of biomass in SRC
It has been shown that under the prevailing site conditions in Germany, biomass production by hybrid poplars exceeds that of willow clones in most cases ([21]). The first step in this study was to employ the empirical yield model developed by Ali ([3]) to predict the production potential of SRC hybrid poplar on arable land in Saxony. The model includes climate variables (mean precipitation and temperature values during the growing season) and the plant available water capacity (AWC
RD) depending on texture and rooting depth. The German site quality index for arable soils (“Ackerwertzahl” - AZ) was also implemented. The AZ may vary between 7 (very poor) and 100 (very good). In the case of sites with AZ = 50 the harvested yield of the reference crop wheat is roughly 50 % of that of sites with AZ = 100 (for further details on soil parameters, see [1]). However, digital data of AZ values is currently not always available at a high spatial resolution. In the case of Saxony, digital AZ data exists only in the form of an aggregated value at the community administration level ([25]). This limits the spatial resolution of the modeling results. One of the simulation scenarios developed by Ali ([3]) was taken as a basis for further downscaling (clone: Populus nigra x P. maximowiczii, cv. Max; planting density: 10000 cuttings ha-1; biomass production: average annual growth in tDW ha-1 year-1 at the age of 9 years). It is generally accepted, that on former agricultural land the crucial factor for productivity of SRC is water availability ([33]). Therefore, only soil physical and climatological site indicators were used in the following procedure for downscaling (see scheme in Fig. 1).
Fig. 2 - Relationship between AWCRD and biomass production (biomass = 7.384 · ln (AWCRD) - 24.4; R²=0.89, p<0.001). The minimum for biomass production was fixed at AWCRD = 60 mm (data source: Ali 2009) .
Due to the strong nonlinear correlation between potential biomass production BP
pot and AWC
RD (Fig. 2), the potential biomass production could be expressed as follows (eqn. 1):
This relationship was used to downscale to a higher spatial resolution on the basis of the soil map for Saxony 1:200 000 ([24]).
In a second step, we implemented reduction factors (RF
) ranging from 0 to 1 for low temperature RF
T and drought RF
D, respectively. Employing this ecophysiologically-oriented approach, the lower boundaries for the empirical growth model were defined. This accounted for the existing limits of site gradients in Saxony. The low temperature response followed certain assumptions:
- no biomass production at a mean annual temperature < 5 °C;
- favorable conditions for biomass production from a mean annual temperature of 6.5 °C (here 75 % of the optimal biomass production potential); and
- no limitations at mean annual temperatures ≥ 8 °C.
Petzold et al. ([31]) showed that as the growing season advances, the transpiration of poplar plantations on sites without groundwater access in the drier regions of northern Saxony is controlled by a reduction in water availability ([23]). Based on long-term average climatological conditions we assumed that by the end of June, halfway through the growing season, ≈ 50 % of the biomass production is achieved. Afterwards growth may be severely limited by soil water shortage. Consequently, a drought index I
D was defined as follows (eqn. 2):
where AWC
RD [mm] is the available water capacity at the effective rooting depth and CWB
Summer [mm] is the accumulated climatic water balance during the period April-September. CWB
Summer was calculated as the difference between precipitation and potential evapotranspiration over grass (FAO). All of the climate data (period 1991-2005) originates from SMUL ([43]).
Smoothing functions for temperature and drought response were adjusted according to Fig. 3.
Fig. 3 - Response functions for (A) low mean annual air temperatures (temperature reduction factor RF
T = 0.014·T3 - 0.384·T2 + 3.538·T - 9.82) and (B) drought (drought reduction factor RF
D; for I
D ≤ 0: RF
D =5.92·10-4·I
D+1; for ID>0: RF
D=1).
Growth conditions can improve significantly on warm sites where tree roots have access to groundwater, even in the event of a low climatic water balance. By contrast, sites with long periods of water logging and cold sites are not suitable for poplar cultivation. These specific site conditions were accounted for by incorporating the factor groundwater F
GW as follows:
F
GW = 0; exclusion of poplar SRC at mean upper groundwater level (MUGL) at soil depth ≤ 4 dm (alternative: Alnus glutinosa - see below);F
GW = 1.25; biomass production at mean annual temperatures ≥ 8 °C and 4 dm < MUGL ≤ 10 dm (if temperature < 8 °C thenF
GW =1);F
GW = 1.1; biomass production at mean annual temperatures ≥ 8 °C and 20 dm > MUGL > 10 dm (if temperature < 8 °C thenF
GW =1);
Information pertaining to this site property was derived from soil profile descriptions included in the digital soil map ([24]).
Finally, the complete site-specific biomass production BP
for poplar was calculated as follows (eqn. 3):
Alder (Alnus glutinosa) is recommended as an alternative species for SRC cultivation on very wet sites with MUGL ≤ 4 dm below the surface. This tree species is characterized by a growth culmination at a later age and so grows slower than poplar ([26]). An overall biomass production of 4 tDW ha-1 year-1 was assumed for all wet sites.
The cultivation and production of poplar biomass on warm and dry sites is very uncertain and can drop below 5 tDW ha-1 year-1, or possibly even fail completely. Under such conditions, black locust (Robinia pseudoacacia) provides an alternative, even though the forecast yields may be low. Black locust biomass production may range from 3.3 tDW ha-1 year-1 ([5]) on reclaimed sites formerly used for surface mining of lignite in southern Brandenburg, and up to 5.5 tDW ha-1 a-1 in northeast Brandenburg ([30]). For dry sites characterized by mean annual temperatures > 8 °C and a forecast poplar biomass yield according to eqn. 3 of < 5 tDW ha-1 year-1, black locust SRC was recommended, with an assumed overall biomass production of 5 tDW ha-1 year-1.
Assessment of possible synergies with nature conservation, landscape and soil protection
Data, legal framework, data processing
A fairly good data foundation on features pertaining to soil protection, land-use distribution and nature conservation exists for Saxony. The data used for the analysis and evaluation of specific areas in this study was provided by the Saxon State Office for Environment, Agriculture, and Geology (LfULG). In most cases it was possible to use pre-processed spatial data:
- digital maps of biotope types and land use types derived from color infrared orthophotos (status: 2005);
- digital soil maps 1:200 000 and 1:25 000;
- digital maps of the vulnerability of soils to erosion by water and wind (for more details, see [17]);
- digital terrain model (grid width: 20 m);
- digital maps of protected areas according to various nature conservation regulations;
- digital maps of biotopes valuable for nature conservation, including all legally protected biotopes (status: 2009);
- red lists of threatened species and maps detailing the occurrence of selected threatened species.
Some legal regulations also served to define the specific protection status of certain areas:
- Habitats Directive ([14]) and Birds Directive of the European Union ([13]);
- Saxony Nature Conservation Act ([37]) and Saxony Water Act ([38]);
- EC cross compliance regulations in agriculture ([15]).
The assessment of the risk of erosion by water followed the empirical model provided by the Universal Soil Loss Equation (USLE) developed by Wischmeier & Smith ([45]) and adapted for natural conditions in Germany ([41] - eqn. 4):
where A
is the long term average soil loss [t ha-1 year-1], R
is the rainfall erosivity index [N h-1 year-1], K
is a factor for the soil erodibility [t h ha-1 N-1], S
and L
are topographical factors accounting for slope steepness and length [-], C
is the plant cover factor [-] and P
is a factor that takes account of specific erosion control practices [-].
The interpretations of soil data with regard to single and combinations of the USLE factors transcend the minimum standards of the EC’s cross compliance regulations ([15]). For example, the vulnerability analysis also contains spatial information concerning erosion-prone steep slopes and areas affected by the potential accumulation of surface run-off.
A simplified assessment of the risk of erosion by wind is based on an evaluation of the soil texture, the soil organic matter content in the upper soil, the mean annual wind speed at a height of 10 m, the crops cultivated and the crop rotation, and the height of and distance to wind breaks ([24], [9]). All of the data and additional information were processed in a geographic information system (GIS).
Definition of synergy classes
The effects of SRC cultivation on nature, landscape and soil protection may range from positive to negative, or they may be neutral. Therefore, a classification scheme was developed including seven different categories of synergy (Tab. 2). We used a method that included objective and subjective elements within a GIS, known as a spatial expert system ([28]).
Tab. 2 - Overview of synergy classes for the combination arable land and SRC with regard to the conservation of nature, landscape, and soil.
Synergy class | Description | |
---|---|---|
No. | Denotation | |
1 | very high synergy | expected synergy effects |
2 | high synergy | expected synergy effects but less than in class 1 |
3 | verify synergy | specific evaluation may reveal either a “synergy” or “no synergy” |
4 | No synergy - risk | no synergy effects expected; cultivation of SRC poses a risk |
5 | No synergy - exclusion | cultivation of SRC poses a high risk to nature and the landscape |
0 | neutral - neither synergy nor risk |
neutral area; neither synergy effects nor risks expected |
9 | not assessed | area not evaluated (all land-use types except arable land) |
Several stakeholders from the Saxon State Office for Environment, Agriculture, and Geology, scientists and independent consulting engineers dealing with ecosystem services were involved in the evaluation procedure. Their expert knowledge with regard to landscape ecology, nature and landscape conservation, soil protection, surface water quality, species protection, agricultural management, and biomass production was structured during workshops and personal communication. Furthermore, experts on state subsidies in support of landscape protection were also included. The goal of this procedure was to define indicators facilitating a final categorization of site types according to the classification presented in Tab. 2.
Results
Site-based estimation of biomass production potential
The site-based potential for biomass production was estimated for arable land only. An application of the biomass model for other land-use types was ruled out due to the expected risk of negative impacts of biomass production in terms of soil protection (see Tab. 3). Roughly 617 400 ha, or 88 % of the arable land of Saxony, are suitable for poplar SRC, with a mean biomass production potential of 12 tDW ha-1 year-1 (± 3, standard deviation). A total of 82 900 ha, or 12 % of total arable land in Saxony, were deemed to be suited to alder cultivation, with a mean forecast yield of 4 tDW ha-1 year-1. The potential area of SRC with black locust was 3400 ha (< 1 % of the total arable land), with an assumed biomass production potential of 5 tDW ha-1 year-1. The distribution of the arable area according to different biomass production classes is illustrated in Fig. 4.
Tab. 3 - Criteria and indicators for the identification of soil protection synergy effects arising from SRC on arable land in the State of Saxony. (a): Areas without data or assessment were classified to synergy class 9; (b): The total area corresponds to the whole of Saxony (1 845 488 ha). The value given for each criterion is always relative to the total area of Saxony. (c): Includes aspects of natural and cultural history. Assessment of dominant soil forms only. (d): Category includes bogs and swamps, neglected grassland, rocky and dwarf shrub areas, forests, coppice and hedgerows. (e): No data.
Criterion | Indicator | Classes | Synergy classa |
Area [ha] |
Proportion of total areab [%] |
---|---|---|---|---|---|
Risk of erosion through accumulation of surface run-off (Thalweg erosion) |
soil loss & catchment size |
< 15 t ha-1 and < 2 ha or n.d.e | 0; 9 | 1802500 | 97.7 |
≥ 15 t ha-1 and ≥ 2 ha | 1 | 42908 | 2.3 | ||
Prone to water erosion (K·R·L·S ) (sheet and rill erosion) |
Soil Loss | < 15 t ha-1 or n.d.e | 0; 9 | 1369861 | 74.2 |
15 - < 30 t ha-1 | 2 | 193888 | 10.5 | ||
≥ 30 t ha-1 | 1 | 281739 | 15.3 | ||
Particularly susceptible to erosion; short but steep slopes (sheet and rill erosion) |
factor K·S |
< 0.9 or n.d.e | 0; 9 | 1771993 | 96 |
≥ 0.9 | 1 | 73496 | 4 | ||
Prone to wind erosion ([24]) | degree of potential danger |
none to low or n.d.e | 0; 9 | 1498391 | 75 |
medium | 2 | 183379 | 9.9 | ||
high to extremely high | 1 | 163719 | 8.9 | ||
Wetlands ([24]) | degree of waterlogging | none to medium or n.d.e | 0; 9 | 1543863 | 78.3 |
high to extremely high | 4 | 301625 | 16.3 | ||
Soils worth protecting ([24]) | Potential for development of biotopesc |
none to low or n.d.e | 0; 9 | 1321056 | 71.6 |
high to very high | 3 | 524432 | 28.4 | ||
Current land use according to the main classes contained on the digital land-use maps |
land-use type | diverse land usesd | 5 | 559301 | 30.3 |
grassland | 4 | 306848 | 16.6 | ||
arable land | 1 | 713439 | 38.7 | ||
water bodies | 0 | 40134 | 2.2 | ||
settlement, infrastructure | 9 | 225766 | 12.2 |
Fig. 4 - Potential surface area and site-based biomass production in SRC on arable land in the State of Saxony. The species considered are poplar, alder, and black locust.
Soil protection
The assessment of synergy effects for soil protection on arable sites with SRC was based on seven criteria (Tab. 3), which we- re applied to the total area of Saxony (1 845 488 ha). The main evaluation results are presented in Tab. 3. More than 25 % of the state’s total area, for example, is prone to water erosion. Therefore, perennial crops like trees managed as SRC would have high and very high synergy effects in terms of reducing the erosion potential.
Nature conservation
The discussion process involving nature conservation stakeholders resulted in the application of four synergy classes: 0 (neutral), 1 (very high synergy - Tab. 4), 3 (verify synergy - Tab. 5) and 5 (exclusion - Tab. 6). Areas deemed to have a very high synergy amount to 26 % of Saxony’s total arable land area and mainly are located in the sparsely wooded regions in central and northern Saxony (Tab. 4). The establishment of a biotope network may also prove beneficial (very high synergy), if SRC with edges comprising native tree and shrub species and with a rotation period ≥ 10 years. A visual evaluation was based on merged soil protection and nature conservation data sets (results not shown).
Tab. 4 - Areas characterised by very high synergy if planted with SRC (synergy class 1: “very high”). (a): Less areas of synergy classes 3 and 5.
Synergy class 1 very high synergy |
Criterion | Total area in Saxony [ha] |
Arable landa [ha] |
Proportion of total arable land [%] |
---|---|---|---|---|
Sparsely wooded regions | Regions where Saxony’s regional planning body recommends an increase in the forest cover | 472478 | 180686 | 25.7 |
Buffer along water edges | After a 10 m strip to allow for close to nature development (see Tab. 5) a 20 m strip of SRC should follow (vegetative filter strip) | 104667 | 6509 | 0.9 |
Total area, adjusted by overlapping areas within this synergy class | 555341 | 184045 | 26.1 |
Tab. 5 - Areas requiring further evaluation and the consideration of specific protection goals; assessment on a regional and local scale (synergy class 3: “verify synergy”). (a): Less areas of synergy class 5. (b): Search area with current and potential occurrence of rare or threatened wild plants of arable land.
Synergy class 3 verify synergy |
Criterion | Total area in Saxony [ha] | Arable landa [ha] | Proportion of total arable landa [%] |
---|---|---|---|---|
Landscape conservation area (protected landscape according to IUCN classification) |
Damage and impairment of protection goals defined in management plans to be avoided; responsibility of district administration |
557018 | 173020 | 24.6 |
Sites of community importance (SCI) according to EU Habitats Directive | Damage and impairment of conservation goals of SCIs to be avoided |
168667 | 6397 | 0.9 |
Special protected area (SPA) according to EU Birds Directive | Damage and impairment to conservation goals of SPAs to be avoided |
248965 | 45791 | 6.5 |
Biosphere reserve: development zone (or transition area according to UNESCO definition) | Damage and impairment to specific management goals of development zones to be avoided |
16952 | 4802 | 0.7 |
Nature park (protected landscape according to IUCN classification) | Impairment of goals defined in management plans to be avoided (e.g., recreational function); responsibility of district administration | 198796 | 30761 | 4.4 |
Field margins, sites with rare or threatened wild herb species | Occurrence of rare or threatened (critically endangered, endangered or vulnerable) plant species | 143744 | 84550 | 12.0b |
Areas recommended for biomass production with black locust (Robinia pseudoacacia) | Damage to protected biotopes to be avoided by ensuring a minimum distance of 500 m to black locust SRC |
3408 | 3391 | 0.5 |
Buffer around high priority protected areas | Damage to protected objects in top priority protected areas (see Tab. 5; e.g., shading of margins rich in species) to be avoided by installing a buffer of 20 m | 3337 | 699 | 0.1 |
Habitats of rare and threatened or legally protected wild species |
Damage to be avoided; cultivation of SRC in strips may be an alternative to SRC in closed blocks. Further evaluation needed |
no specific area for evaluation, data incomplete |
- | - |
Total area, adjusted by overlapping areas within this synergy class | 913715 | 272074 | 38.6 |
Tab. 6 - Areas with no nature conservation synergies (synergy class 5: “exclusion”). (a): SächsNatSchG ([37]); (b): SächsWG ([38]).
Synergy class 5 no synergy - exclusion |
Criterion | Total area in Saxony [ha] | Arable land [ha] | Proportion of total arable land [%] |
---|---|---|---|---|
National park; entire area | Long term development and protection of natural self-regulation (processes without human interference) have top prioritya |
9354 | 180 | <0.1 |
Biosphere reserve; core area and buffer zone | Protection goals have top priority as core areas (strict nature reserve) and buffer zones (habitat and species management areas) are nature reserves by lawa | 13165 | 465 | <0.1 |
Nature reserve (IUCN categories: strict nature reserve and habitat/ species management area) |
Protection goals have top priority by lawa | 51861 | 1180 | 0.2 |
Natural monuments (areas up to 5 ha) |
Protection goals have top priority by lawa | 6500 (approx.) |
250 (approx.) |
<0.1 |
Worth protecting biotopes (selective biotope mapping) |
Protection goals have high priority, legally designateda protected biotopes are included. Damage forbidden | 97724 | 1649 | 0.2 |
Water edges | A strip of 10 m alongside waterway should be allowed to develop in a way that is close to natureb |
56483 | 4675 | 0.7 |
European hamster (C. cricetus) habitats |
Protected according to annex IV of the Habitats Directive ([14]). Habitat must be preserved. Cultivation of SRC in strips may be possible. Further evaluation needed. | 10401 | 8727 | 1.2 |
Total | 193704 | 16363 | 2.3 |
For an area > 272 000 ha, or 38 % of Saxony’s arable land, an additional, more detailed evaluation (synergy class 3) is needed in order to come to a final synergy classification (Tab. 5). Special knowledge of the landscape characteristics and the specific protection goals is necessary. To achieve this, an assessment should be made on a local scale in cooperation with stakeholders from the district or even municipality level.
Areas not suitable to SRC plantations (Tab. 5) consist of sites that are top priority for nature conservation goals, as defined by law. Overall, 2.3 % of Saxony’s total arable land, or 16 363 ha, was grouped in synergy class 5. Finally, 231 669 ha respectively 33 % of the arable land were assessed to be neutral if planted with SRC. That means neither synergy nor risk is expected on these sites.
Synthesis
The results of the assessment of the soil protection and nature conservation features were merged within a GIS. Where more than one synergy class was found for a particular area on the basis of the different criteria, the inferior synergy class was prioritized.
The arable land deemed to have high and very high synergy ratings amounted to 236 462 ha. The site-based potential for biomass production was related to the synergy values derived for the respective areas (Fig. 5). As expected, larger areas with a high biomass production potential were identified in the hilly loess region, which is dominated by large-scale agricultural cultivation. This region is characterized by a low proportion of forest cover and often at risk for soil erosion, but also high soil quality and moderate climate conditions. Consequently, the average site-specific biomass production is also rather high in this region, with ≥ 15 tDW ha-1 year-1 (Fig. 6). Large areas with somewhat lower production potential can be found at the transition to the drier lowlands of Saxony. Potential areas for SRC were also identified at the transition to the Ore Mountains in southern Saxony. However, the expected biomass production in this latter region is mainly < 10 tDW ha-1 year-1 due to a shorter growing season and lower soil quality.
Fig. 5 - Site-based biomass production potential of SRC plantations on arable land deemed to have either a high or a very high synergy effect with respect to soil protection and/or nature conservation within the State of Saxony (Germany). Boundaries are natural landscape units (“Makrochoren”).
Fig. 6 - Site-based biomass production of SRC plantations on areas identified as having a high or very high synergy effect with respect to soil protection and/or nature conservation goals.
Of the whole arable land area assessed, 152 907 ha (21 %) were classified as having a very high synergy effect; 83 555 ha (12 %) high synergy; 313 718 ha (45 %) need further evaluation; 95 087 ha (13 %) have no synergy but potentially pose a risk; 16 610 ha (2 %) have no synergy and should be completely excluded from SRC; the effect of SRC is deemed neutral on 50 932 ha (7 %) and 1387 ha (< 1 %) were not assessed (see relative proportions in Fig. 7).
Fig. 7 - Distribution of synergy classes with respect to SRC on arable land after all soil protection and nature conservation assessment criteria are merged. (n.a.): not assessed.
Discussion
The range of the site-based biomass production estimates attained in this study compares with independent results from demonstration plots in Germany ([21]). They found average poplar SRC yields of 6 to 18 tDW ha-1 year-1 depending on soil and climatic conditions. However, it should be noted that available information regarding biomass yields in Saxony has been collated from experimental and demonstration plots. This often results in a bias in the findings in favor of higher yields than can be achieved under actual management conditions. At the same time, ongoing improvements to management technologies will counterbalance these possibly too optimistic biomass production estimates ([29]).
The assessment of the impact of SRC on areas with regard to soil protection excluded land presently used as grassland (mainly pasture). The main concerns here were a distinct loss of soil organic carbon, leaching of nitrate into the groundwater, and the release of carbon dioxide into the atmosphere due to increased mineralization following conversion from grassland to SRC ([11], [7]). However, a land use change from grassland to SRC does not necessarily imply high losses of soil organic carbon ([12]). Hence, the total green house gas balance will be highly dependent on type and management of grassland, cultivation practice, in particular ploughing as well as the lifetime and the re-cultivation technique after abandonment of SRC plantations ([12]).
The scale of the study design was the regional level. This information may serve for a rational allocation of subsidies to encourage SRC and maximize synergy effects according to the site conditions in different regions. However, the relatively large area of synergy class 3 “verify synergy” needs to be refined and tested at a lower (farm, district) level. Detailed knowledge of objects of nature and landscape protection, and existing management plans for protected areas under the responsibility of district authorities should be incorporated in an ongoing and refined appraisal process. For better incorporation of potential biotope networks, however, a more detailed assessment according to the type of biotope and other overlapping criteria is necessary. A key problem for each assessment remains the definition of criteria and appropriate indicators. In particular, indicators for the assessment of the effects of SRC on biodiversity still rely on existing data sets not specific to SRC. It would appear to be necessary to monitor the actual effects on biodiversity of newly established SRC on arable land for the development and adaptation of appropriate indicators.
Another planning approach, the sustainability appraisal framework (SAF), also includes the economic and social implications of a land-use change due to increased biomass production ([20]). These authors adopted an approach in which environmental and physical constraints are mapped with the identification of stakeholder aspirations. The abundance of butterflies was found to be an appropriate indicator for the assessment of the biodiversity of different biomass crop systems. However, which mechanisms are suited to implementing the identified synergy effects in practice remains open. Fohrer et al. ([18]) combined the agri-economic model ProLand, for the generation of spatially distributed land-use scenarios, the grid based YELL model, to simulate the distribution of breeding habitats of key species, and finally the hydrological model SWAT, to assess changes in land use on hydrologic ecosystem services. With this combined model framework, Fohrer et al. ([18]) demonstrated multidimensional trade-offs. This approach may represent an important information base for decision makers. Nevertheless, the authors highlighted that the uncertainty associated with the model is high and needs to be addressed. The priority areas identified for SRC in this study may also serve as an input for a spatially explicit modeling of scenarios and for an integrated assessment of hydrologic ecosystem services, like flood protection, water quality or groundwater recharge ([6]) at the catchment scale. Such efforts are currently being undertaken as part of the joint research project AgroForNet([2], [44]).
Conclusions
The conceptual framework for planning SRC and the incorporation of nature conservation and soil protection goals presented in this paper yields spatially explicit information concerning priority areas and the site-specific biomass production potential at the regional scale. The results may serve as important basic information supporting the allocation of subsidies for a joint improvement of biomass production, soil protection, and nature conservation.
However, the approach for yield estimation is limited to agricultural site conditions. An equivalent evaluation for grassland (mainly pasture) is lacking as yet but should be carried out. Further research is needed to determine whether advanced SRC cultivation techniques like direct planting, planting in strips, and the avoidance of deep ploughing when the plantation is established on grassland sites may prevent excess mineralization of soil organic matter. Nevertheless, it is clear that grassland characterized by a low level of management intensity is often of high nature conservation value and should not converted into SRC.
To prevent wind erosion, it may be sufficient to plant fast growing tree species in strips rather than blocks. This would mean that smaller areas are needed to maximize synergy effects. The optimal land-use type in susceptible regions will, therefore, more closely resemble an agroforestry system than a conventional SRC plantation.
Further development of the framework should also include other ecosystem services related to soil and water resources (e.g., carbon sequestration, flood protection, groundwater recharge). Another focus should be the refinement of the planning tool at the local (farm, district) scale. Stakeholders must be involved at each step in order to make the greatest use of existing knowledge, particularly information on biodiversity, and to ensure broad acceptance of planning outcomes. A gap in the existing knowledge was identified with respect to the uncertain soil ecological impact of advanced SRC establishment techniques on sites which hitherto have been used as grassland. Finally, indicators for better assessment of biodiversity in SRC should be developed on the basis of current monitoring programmes.
Acknowledgements
The study was funded by the Saxon State Ministry of the Environment and Agriculture (SMUL). We acknowledge the valuable comments of two anonymous reviewers.
References
Gscholar
Online | Gscholar
Gscholar
Gscholar
CrossRef | Gscholar
Online | Gscholar
Gscholar
Gscholar
Gscholar
Gscholar
Gscholar
Gscholar
Gscholar
Gscholar
Gscholar
Authors’ Info
Authors’ Affiliation
Public Enterprise Sachsenforst, Competence Center for Wood and Forestry, Bonnewitzer Str. 34, 01769 Pirna (Germany)
TU Dresden, Institute of International Forestry and Forest Products, Pienner Str. 19, 01737 Tharandt (Germany)
Consulting Engineers, Ingenieurbüro Feldwisch, Karl-Phillip-Str. 1, 51429 Bergisch Gladbach (Germany)
Office for Nature Conservation and Sustainable Land Use, Rittergut 16, 01909 Großhartau (Germany)
TU Dresden, Institute of General Ecology and Environmental Protection, Pienner Str. 7, 01737 Tharandt (Germany)
Saxon State Agency for Environment, Agriculture and Geology, Postfach 540137, 01311 Dresden (Germany)
TU Dresden, Institute of Soil Science and Site Ecology, Pienner Str. , 01737 Tharandt (Germany)
Corresponding author
Paper Info
Citation
Petzold R, Butler-Manning D, Feldwisch N, Glaser T, Schmidt PA, Denner M, Feger K-H (2014). Linking biomass production in short rotation coppice with soil protection and nature conservation. iForest 7: 353-362. - doi: 10.3832/ifor1168-007
Academic Editor
Marco Borghetti
Paper history
Received: Oct 31, 2013
Accepted: Nov 14, 2013
First online: May 19, 2014
Publication Date: Dec 01, 2014
Publication Time: 6.20 months
Copyright Information
© SISEF - The Italian Society of Silviculture and Forest Ecology 2014
Open Access
This article is distributed under the terms of the Creative Commons Attribution-Non Commercial 4.0 International (https://creativecommons.org/licenses/by-nc/4.0/), which permits unrestricted use, distribution, and reproduction in any medium, provided you give appropriate credit to the original author(s) and the source, provide a link to the Creative Commons license, and indicate if changes were made.
Web Metrics
Breakdown by View Type
Article Usage
Total Article Views: 46875
(from publication date up to now)
Breakdown by View Type
HTML Page Views: 38867
Abstract Page Views: 2360
PDF Downloads: 4523
Citation/Reference Downloads: 35
XML Downloads: 1090
Web Metrics
Days since publication: 3632
Overall contacts: 46875
Avg. contacts per week: 90.34
Article Citations
Article citations are based on data periodically collected from the Clarivate Web of Science web site
(last update: Feb 2023)
Total number of cites (since 2014): 6
Average cites per year: 0.60
Publication Metrics
by Dimensions ©
Articles citing this article
List of the papers citing this article based on CrossRef Cited-by.
Related Contents
iForest Similar Articles
Review Papers
Remote sensing-supported vegetation parameters for regional climate models: a brief review
vol. 3, pp. 98-101 (online: 15 July 2010)
Research Articles
Comparison of soil CO2 emissions between short-rotation coppice poplar stands and arable lands
vol. 11, pp. 199-205 (online: 01 March 2018)
Review Papers
Accuracy of determining specific parameters of the urban forest using remote sensing
vol. 12, pp. 498-510 (online: 02 December 2019)
Review Papers
Remote sensing of selective logging in tropical forests: current state and future directions
vol. 13, pp. 286-300 (online: 10 July 2020)
Research Articles
Efficient measurements of basal area in short rotation forests based on terrestrial laser scanning under special consideration of shadowing
vol. 7, pp. 227-232 (online: 10 March 2014)
Research Articles
Afforestation monitoring through automatic analysis of 36-years Landsat Best Available Composites
vol. 15, pp. 220-228 (online: 12 July 2022)
Technical Reports
Detecting tree water deficit by very low altitude remote sensing
vol. 10, pp. 215-219 (online: 11 February 2017)
Research Articles
Experimenting the design-based k-NN approach for mapping and estimation under forest management planning
vol. 5, pp. 26-30 (online: 27 February 2012)
Research Articles
Evaluation of hydrological and erosive effects at the basin scale in relation to the severity of forest fires
vol. 12, pp. 427-434 (online: 01 September 2019)
Review Papers
Remote sensing support for post fire forest management
vol. 1, pp. 6-12 (online: 28 February 2008)
iForest Database Search
Search By Author
Search By Keyword
Google Scholar Search
Citing Articles
Search By Author
Search By Keywords
PubMed Search
Search By Author
Search By Keyword