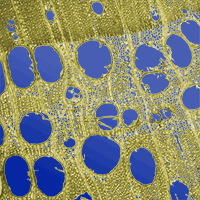
Climatic fluctuations trigger false ring occurrence and radial-growth variation in teak (Tectona grandis L.f.)
iForest - Biogeosciences and Forestry, Volume 9, Issue 2, Pages 286-293 (2015)
doi: https://doi.org/10.3832/ifor1100-008
Published: Sep 28, 2015 - Copyright © 2015 SISEF
Research Articles
Abstract
The objective of this study was to examine the interaction of extreme growth years in teak (Tectona grandis) with climatic conditions of current, antecedent and subsequent years, in order to explain the nature and the effects of climatic variability on teak growth in northeastern Thailand. A 33-year tree-ring index was constructed and extreme growth years during the period 1976-2008 were identified. A superposed epoch analysis (SEA) was used to study the interaction of climatic data and extreme growth years. Extreme growth years were derived from eight wider and seven narrower annual rings identified using the Cropper’s method. Seventeen false rings were detected using the threshold value ≥ 80% of false ring occurrence for all samples in each growth year. False ring occurrence was associated with narrow ring width formation and triggered by increasing maximum and mean temperatures at the beginning of the rainy season (May to August). In the third year after false ring formation, we observed a pattern of wet year occurrence when annual rainfall and relative humidity in September to December were higher than in adjacent years. Moreover, in the sixth year before false ring formation, a wet year was observed when relative humidity in September to December was higher than in adjacent years. Wider ring width index occurring in a particular year was found to be triggered by a decrease in maximum and mean temperatures in May to August of the current year, suggesting that wet years promote teak growth. The third year after the formation of wider rings was characterized by a low annual rainfall. Our results showed that drought years trigger false ring and narrow ring formation, while wet years trigger wide ring formation in teak. A cycle of wet years between the sixth year prior to, and the third year after, the formation of false rings was also observed, as well as the occurrence of drought in the third year since the formation of wide rings. False ring, narrow ring and wide ring occurrences appear to be good indicators of a 3-6 year climate fluctuation pattern, similar to that of the El Niño-Southern Oscillation (ENSO) cycle in this region.
Keywords
Extreme Event, False Ring, Pointer Year, Superposed Epoch Analysis, Teak (Tectona grandis), Tree-ring
Introduction
Teak (Tectona grandis L.f.) is one of the most well known tropical tree species for its high-quality and prized timber ([37], [3]). Teak wood is suitable for multiple uses due to its various favorable characteristics and properties, including fine grain, beautiful golden color, and durability with respect to weather, insects and fungi ([22]). Archaeological studies showed that teak was widely used in Egypt, India and China more than 2500 years ago ([16]). Teak is naturally distributed in tropical monsoon Asia, including India, Myanmar, Laos PDR, Indonesia and Thailand ([44], [49]). However, the increasing demand of this quality timber has caused widespread planting even in areas outside the natural distribution regions, such as the plantations in Southeast Asia, Africa and South America ([24]).
It is important to understand factors affecting teak growth, to ensure sustainable production of high-quality teak wood and appropriate forest management practices. Several studies indicate that teak is a deciduous to semi-deciduous tree growing in a wide range of climatic and edaphic conditions ([42]). Kaosa-ard ([23]) also reported some environmental factors controlling the distribution and growth of teak such as rainfall (500-5000 mm year-1), soil (best on deep and well-drained alluvial soils with relatively high mineral salts), light intensity (75-90%) and temperature (27-36 °C).
Recently, IPCC ([21]) reported global average surface temperature increasing over the period 1880-2012 by about 0.85°C. Analysis of Thailand meteorological data in the past six decades indicated little changes in rainfall and increases in annual mean, minimum and maximum temperature, especially in the last decade ([47]). The fluctuation of some climatic variables may affect tree growth and adaptation because they are important environmental factors determining ecological niches of tree species and their distribution patterns ([45]).
Several researchers have suggested that drought causes significant losses in growth of teak and other plants ([30], [1], [14], [11]). A growth study of juvenile teak, which involved 20 days drought followed by 5 days rehydration treatments, indicated that growth declined due to drought stress and rapidly recovered a few days after rehydration ([20]). In rainy season, pre-monsoon showers broke the cambial dormancy and radial-growth was initiated ([32]). Wood formation started after the budding and continued until the end of the rainy season ([27]), while drought during active growing season caused false ring formation in teak ([31], [28]). Rao & Rajput ([37]) found the maximum radial-growth of teak during the monsoon period, while Kokutse et al. ([25]) reported that annual ring width is strongly depended on mean monthly temperature and is associated with bud break during the rainy season.
Teak has been widely used for tree-ring analysis, to determine climate-growth response. Several studies discovered the significant positive relationship between rainfall and teak growth in the monsoon season ([33], [39], [36], [5]). Ram et al. ([35]) also found that the moisture availability in the post-monsoon period of the previous year had a significant role in the development of teak growth in the current year.
In this paper, we identified extreme events (pointer years), which were derived from the variation of teak ring width index and false ring formation. The interaction of these pointer years with antecedent and subsequent climatic conditions were then examined with the aim of understanding the nature and effects of climatic variability on teak growth in northeastern Thailand. Instead of analyzing the climate-growth response using simple correlation and multiple regression analysis, we used the superposed epoch analysis (SEA) to establish the climatic fluctuations triggering false ring occurrence and radial-growth variation in teak.
Material and methods
Study site
The study site was located at the Wang Nam Kiao Forestry Student Practice Station (WNKFSPS) of the Faculty of Forestry, Kasetsart University in the Nakhon Ratchasima province, lower northeastern Thailand (14° 29′ N, 101° 56′ E) at 339 m a.s.l (Fig. 1). Most of the teak growing on this site formed false rings, which normally are obstacles for tree-ring analysis. However, they provided an opportunity to study factors triggering false ring formation.
Climatic data
The longest climatic data of total monthly rainfall (RF), relative humidity (RH) and monthly temperature - maximum (Tmax), minimum (Tmin), and mean (Tmean) temperature - for forty years (1969-2008) were derived from the nearest meteorological station at the Sakaerat Environmental Research Station (SERS). Based on RF and Tmean, climate was classified into wet and dry periods during March to November and December to February, respectively (Fig. 2). Total monthly rainfall rapidly increased at the beginning of wet period (March to May), followed by a short period of drought during June to August. The highest rainfall was recorded at the end of the wet period in September-October, and the rainfall amount rapidly decreased from November to February (Fig. 2). Maximum, minimum, and mean monthly temperature slightly increased from the beginning of the year in the dry period to the highest temperature at the beginning of the wet period in April, and gradually decreased to the lowest temperature in the dry period at the end of the year (Fig. 3).
Fig. 2 - Mean monthly total rainfall and temperature in the period 1969-2008, with indications of wet and dry periods (source: Sakaerat Environmental Research Station, Nakhon Ratchasima province, Thailand).
Fig. 3 - Monthly variation of maximum (Tmax), minimum (Tmin) and mean (Tmean) temperature in the period 1969-2008 (source: Sakaerat Environmental Research Station, Nakhon Ratchasima province, Thailand).
Methods
A total of seven natural teak trees that were dominant and had a symmetrical crown and straight trunk were selected from a small forest stand of approximately 15 teak trees growing on well drained soil. From each sample tree, four increment cores were taken at breast height (1.3 m) in cardinal directions (north, east, west and south) using an increment borer. Following the standard procedures of dendrochronology ([43]), twenty eight increment cores were carefully fixed on support wood slabs and gently polished until tree-rings on transverse surfaces were clearly visible. This was done at the Laboratory of Tropical Dendrochronology (LTD), Kasetsart University, Faculty of Forestry (KUFF) in Bangkok (Thailand). Annual rings and intra-annual rings were identified using the techniques of visual cross-matching ([17]) and vessel size investigation ([28]). Annual ring widths were measured at a resolution of 0.001 mm with the accuracy of 0.010 mm m-1 using the TA Unislide Tree-Ring Measurement System (Velmex Inc., New York, USA) interfaced with a microcomputer and a 4x to 40x magnified stereo microscope. Measurement errors were corrected with a computer-assisted software of cross-dating and quality control procedures, namely the COFECHA program ([18]). Annual ring width series were standardized to minimize age effect and maximize the observation of the environmental factors of interest using the ARSTAN software package ([8]). Expressed Population Signal (EPS) was calculated to determine the usefulness of the standardized annual ring width index based on their sample sizes and correlation values. Wigely et al. ([48]) suggested that the index supported by EPS values ≥ 0.85 could be accepted as reliable chronology for tree-ring analysis. Additionally, the number of identified false rings in each year of all sample cores was counted and converted to the percentage of false ring frequencies in each year.
Extreme growth fluctuations, called pointer years, were extracted from the standardized tree-ring chronology using the Cropper’s method ([10], [38]). Pointer years were calculated as the normalized differences between the standardized growing value in the year i
and the mean of the standardized growing values within a five-year moving average, called moving windows, as follows (eqn. 1):
where Z
i is the pointer year index value in the year i
(i
= 1964, 1965,..., 2006), X
i is the standardized growing value in the year i
, mean
[window
] is the arithmetic mean of ring width within the moving windows of X
i-2, X
i-1, X
i, X
i+1, X
i+2, and stdev
[window
] is the standard deviation of ring width within the moving windows of X
i-2, X
i-1, X
i, X
i+1, X
i+2
Values of Z
i which were ≥ 0.75 or ≤ -0.75 were defined as positive (wider ring) or negative (narrower ring) pointer years, respectively ([46]). In the case of false-ring occurrence, pointer years were detected using the threshold of 80% or higher in each year. Using this threshold, at least 6 trees from the total of 7 teak sample trees were found to have false rings in year i
.
Superposed epoch analysis (SEA) is a statistical method originally used to test for significant relationships between the occurrence of a specific event and the strength of recruitment in the corresponding years ([29]). SEA was used to examine the relationship between extreme events (-0.75 ≥ Z
i ≥ 0.75) and false ring frequencies (≥ 80%) and time series of annual and seasonal climatic data (January to April, May to August and September to December). The climate data, included RF, Tmax, Tmean, Tmin and RH. The relationship between false ring pointer years and standardized annual ring width index was also examined. SEA compared these time series with extreme events by superposing windows of concurrent and lagged climatic conditions for each extreme growth year. Simulations for 1000 trials with 28376 random seed values were used to develop confidence intervals of the means, with the aim of determining whether climate was significantly different from average during six years prior and four years after extreme growth years. In this study, SEA was carried out using the EVENT software package ([19]).
Results
Tree-ring width data
Cross-dating was successful in all 28 teak cores, though the identification of false rings was difficult and time consuming. False rings occurred in both earlywood and latewood, as one or more rows of paratracheal parenchyma and small vessels scattered nearby dense fibers without paratracheal parenchyma (Fig. 4). The average annual ring width series and the standardized annual ring width index are shown in Fig. 5. Mean sensitivity (MS, the mean relative change between adjacent ring widths - [17]), standard deviation, and autocorrelation of these ring width series were 0.422, 0.421 and 0.170, respectively. The average annual ring width series rapidly increased during the period 1962-1964, gradually decreased during the period 1965-1979, and stabilized during the period 1980-2008 (Fig. 5b). When the age trend at the beginning of tree growth was removed, the standardized ring width index fluctuated around and close to the mean value of 1 (Fig. 5b). During 1976-2008, the EPS values were at least 0.85, indicating a reliable chronology, while EPS values obtained for previous periods were lower than such threshold, likely due to the reduced sample sizes, indicating unreliable chronologies (Fig. 5c, Fig. 5d). Thus, the low EPS chronologies were discarded from this study.
Fig. 5 - Annual ring width index of teak (Tectona grandis) in the period 1962-2008. (a) average annual ring width series; (b) annual ring width index; (c) EPS values; (d) sample sizes. The gray shaded area indicates the period of lower reliability of the annual ring width index due to smaller sample sizes.
Pointer year identification
The pointer years of fast (wider annual ring) and slow (narrower annual ring) growth derived using the Cropper’s method are shown in Fig. 6. Teak rapid growth with pointer year index value (Z
i) ≥ 0.75 occurred in 1964, 1967, 1971, 1976, 1978, 1983, 1986, 1991, 1994, 1999 and 2000, while growth suppression (Z
i ≤ -0.75) occurred in 1968, 1973, 1979, 1984, 1987, 1990, 1995, 1998 and 2001. Due to the unreliability of annual ring width index prior to 1976, previous pointer years in the period 1962-1975 were not considered in this study.
Fig. 6 - Pointer years derived from annual ring width index of teak (Tectona grandis). Years with significant pointer year index values (-0.75 ≥ Z
i ≥ 0.75) above the upper and below the lower dashed lines (black column) indicate the wide and narrow ring width pointer years, respectively.
Visual cross-matching and vessel size investigation indicated that intra-annual growth rings occurred within actual annual rings (Fig. 4). The successful cross-dating using the COFECHA software also confirmed the accuracy of the intra-annual growth ring identification. The years 1968, 1970, 1972, 1977, 1979, 1980, 1981, 1982, 1984, 1987, 1988, 1989, 1990, 1993, 1996, 1997, 1998, 2002, 2005 and 2006 were defined as false ring pointer years, when false ring occurrence was at least 80% (22 sample cores - Fig. 7). As previously mentioned, the pointer years before 1976 were rejected from this study due to the unreliability of the annual ring width index.
Fig. 7 - False ring frequencies in each growing year. False ring pointer years (≥ 80% false ring occurrences) are represented by black columns.
Climate-pointer year interaction
The superposed epoch analysis (SEA) indicated the existence of a climate-pointer year interaction, as reflected by the significant relationship between the increase of Tmean and Tmax in the rainy season (May to August: p≤0.05 - Fig. 8b, Fig. 8c) with the occurrence of false rings and the negative values of annual ring width index (p≤0.05 - Fig. 8a). Additionally, there was a significant positive relationship between the false ring occurrence and the relative humidity (RH) in the transition period to the rainy and cold season (September to December - Fig. 8d). A cycle of wet periods in the sixth year prior to, and the third year after, the occurrence of the false rings was detected. Moreover, an increasing annual rainfall departure from the mean of annual rainfall index was found in the third year after false ring occurrence (p≤0.05 - Fig. 8e). In years in which Tmean and Tmax for the rainy season (May to August) were significantly lower (p≤0.05 - Fig. 8f, Fig. 8g), annual ring width index values were larger than those of other adjacent years. In the third year after the occurrence of a wider annual ring, the annual rainfall was significantly lower than that of other adjacent years (p≤0.05 - Fig. 8h).
Fig. 8 - Superposed epoch analysis (SEA) showing the departures of actual from simulated events: (a) annual ring width index; (b) Tmean (May to Aug); (c) Tmax (May to Aug); (d) RH (Sep to Dec); (e) annual rainfall of years with false ring pointer year occurrence; (f) Tmean (May to Aug); (g) Tmax (May to Aug) and annual rainfall were significantly related with wide annual ring occurrences. Dashed and solid lines indicate confidence intervals at 95% and 99%, respectively based on 1000 random simulations. Significant departures (p≤0.05) from random expectations are indicated by stars.
Although the narrow annual rings generally occurring in drought years were significantly associated with false ring formation, there was no significant relationship with the recorded climatic data. Fig. 9 summarizes the patterns of formation of false rings in teak, and their association with the narrow ring width and drought conditions in the growing year, and with the wet conditions in the sixth year prior to false ring occurrence, as well as with the third year after. The formation of wider annual rings was found to be linked with wet conditions in the growing year and with drought conditions in the third year after the occurrence of the wider annual rings.
Fig. 9 - Cycle of false ring formation, narrow ring and wide ring formations in teak, related to the cycle of drought and wet occurrences.
Discussion
Teak in this study area was appropriate for investigating environment-growth interaction, especially in term of climate fluctuation, because it formed wide and narrow rings intermixed through time (a phenomenon called sensitivity). The teak annual ring width index showed a high mean sensitivity (MS) value of 0.42, fairly close to MS values (0.5) observed for natural teaks growing in mountainous areas of northern Thailand ([33]). Interestingly, the MS of Thai teak in northwestern Thailand was only 0.27 ([6]), and that of the Indian teak varied by site ranging from 0.22 to 0.43 ([4], [39], [36], [35], [5]). Although Bhattacharyya et al. ([4]) suggested that MS value of 0.22 was a moderate value, the amount of variation that could be explained by climate was approximately 57.15%. In case of MS value = 0.5, Pumijumnong et al. ([33]) suggested that growth variability of northern Thailand teak triggered by climate was approximately 61%.
In this study, SEA results showed that teak false rings were associated with narrow ring width, and drought during the rainy season. Priya & Bhat ([31]) and Palakit et al. ([28]) reported false ring formation in both earlywood and latewood teaks. Artificial drought during the early growing season also induced false ring occurrence in teak ([32]). Palakit et al. ([28]) also confirmed that increasing temperature followed by increasing rainfall at the end of the monsoon period stimulated teak false ring occurrence. As for other tree species, Cherubini et al. ([7]) suggested that false ring formation in Mediterranean trees was caused by the interruption of the normal growth during the season caused by either a dry or wet period. These false rings could be identified by carefully studying xylem cells at the transition from the thin band of latewood to earlywood cells. This transition was gradual, diffuse, and not as abrupt or sharp as in annual ring boundaries. It was similar to the variation of vessel lumen area in Erica arborea, whose annual rings with intra-annual density fluctuations (IADFs) suddenly decreased in vessel lumen size at the middle of earlywood and formed a band of latewood-like cells ([15]). Battipaglia et al. ([2]) also found false ring formation in earlywood or latewood related to drought. Nabais et al. ([26]) suggested that the correlation between climate and IADFs is driven by site conditions and is less related to the species. Copenheaver et al. ([9]) suggested that false rigs in intermediate and co-dominant trees were due to tree growth suppression more than climatic conditions.
False ring occurrence in each growing year was was related to the occurrence of narrow annual ring and increasing temperature, but no relationships were detected with annual rainfall and relative humidity (RH). The relationship between false ring occurrence and climatic data in the adjacent years was unclear at this time. A spectral analysis of the northwestern Thai teak chronology over the past 448 years indicated a significant range of teak growing cycle related to El Niño-Southern Oscillation (ENSO) variability spanning 2.2-4 years ([6]). This was similar to the cycle of Moisture Index (MI) in each of 3.5 years reconstructed from teak ring index in India ([35]). Solomon et al. ([41]) also suggested that historical El Niño events occurred about every 3-7 years, while Singhrattna et al. ([40]) found a significant correlation between summer monsoon precipitation and large scale climatic features of the Pacific sea surface temperatures (SSTs) and ENSO. Therefore, the fluctuation of ENSO causing precipitation might induce the variation of tree growth, and foster cycles of 3 to 6 years annual rainfall and RH.
Tree growth rates are also related to climatic fluctuation. This study found an increasing annual ring width index of teak with decreasing Tmean and Tmax in the rainy season. These results were similar to other dendroclimatological studies of teak. In Thailand, Buckley et al. ([6]) found a significant relationship of teak growth rate with reduced Tmean at p≤0.05. Moreover, it was consistent with the study of Pumijumnong et al. ([34]), who reconstructed temperature from teak ring index, and found a negative relationship between temperature and teak growth. Teak annual ring variation in India also showed a significant negative correlation with temperature ([39], [36], [35]).
In this study, the decrease in annual rainfall in the third year after the occurrence of a wide annual ring width was another interaction between climate and pointer years, suggesting the existence of an underlying climatic cycle. D’Arrigo et al. ([12], [13]) found a strong correlation of the reconstructed Palmer Drought Severity Index (PDSI) and warm pool sea surface temperature (SST), based on Indonesian teak ring width series, representing approximately 5-7 year ENSO cycle. This could mean that the cycle of extreme wet or dry year occurred every 5-7 years, similar to our study of the interaction between annual rainfall and wide annual ring width index. The interpretation is that false ring associated with narrow ring width occurred in a dry year (high temperature) followed by wet year in the third year. As well, wider rings occurred in a wet year of low temperature followed by a cycle of drought year three years later.
Although this study could not find a significant relationship between rainfall and teak growth in any particular year, several studies have suggested increasing tree growth with abundant rainfall and moisture ([39], [4], [36], [34], [33]). After the occurrence of a wider annual ring width, the amount of annual rainfall three years later was significantly lower than other adjacent years. This cycle covered six year intervals, similarly to the climatic fluctuation due to ENSO effect describe above. Although Tmean and Tmax which were below the average induced wider annual ring formation in teak, the study of Vongvisessomjai ([47]) indicated increasing trends of annual mean, minimum and maximum temperatures in Thailand, especially during the last decade. Therefore, teak growth rate may decrease due to the limitation of increasing temperature. This is particularly important for the growth of trees in both natural forest and plantations, which provide direct benefit in terms of timber and indirect benefit in terms of carbon sequestration. On the other hand, the formation of false rings is an indicator of unfavorable environmental factors affecting tree growth during the growing periods, as well as the formation of narrow rings. These observations can contribute to the development of strategies for adaptation to global warming and climate change mitigation, especially on plants which are primary producers (autotrophic) in terrestrial ecosystems.
Conclusions
This study extracted pointer years of large, small, and false ring occurrences from teak annual ring width series. High maximum and mean temperatures in May to August triggered the formation of false rings due to drought occurrences during the beginning of the growing period. Additionally, false ring occurrence was found to be associated with small annual ring widths. In the third year after the occurrence of false rings in a particular drought year, relative humidity in September to December and annual rainfall were higher than in other adjacent years; and in the sixth year before false ring formation, annual rainfall was higher than in other adjacent years. Decreased maximum and mean temperature in May to August (wet period) in a particular year induced large annual ring width formation. In the third year after large annual ring occurrence in a particular wet year, annual rainfall was lower than in other adjacent years. Climatic fluctuation patterns were found not to be significantly associated with the pointer years of small annual ring widths. While climatic cycles are not fully understood, the observations in this study appear to suggest that the cycle swings back and forth every 3-6 years, similarly to the ENSO cycle.
Acknowledgements
The authors wish to thank Dr. A.Y. Omule, visiting researcher at the Kasetsart University Faculty of Forestry (KUFF), for his valuable comments and suggestions in scientific methodology, data analysis and manuscript revision. This project was partly funded by a grant from the Center for Advanced Studies in Tropical Natural Resources, National Research University-Kasetsart University, Bangkok (Thailand).
References
CrossRef | Gscholar
Gscholar
Gscholar
Gscholar
Gscholar
Gscholar
Gscholar
Gscholar
Gscholar
Gscholar
CrossRef | Gscholar
Gscholar
Authors’ Info
Authors’ Affiliation
Faculty of Environment and Resource Studies, Mahidol University, 73170 Nakhon Pathom (Thailand)
Khwanchai Duangsathaporn
Laboratory of Tropical Dendrochronology (LTD), Department of Forest Management, Faculty of Forestry, Kasetsart University, 10900 Bangkok (Thailand)
Center for Advanced Studies in Tropical Natural Resources, National Research University-Kasetsart University, Kasetsart University, 10900 Bangkok (Thailand)
Department of Forest Biology, Faculty of Forestry, Kasetsart University, 10900 Bangkok (Thailand)
Corresponding author
Paper Info
Citation
Palakit K, Duangsathaporn K, Siripatanadilok S (2015). Climatic fluctuations trigger false ring occurrence and radial-growth variation in teak (Tectona grandis L.f.). iForest 9: 286-293. - doi: 10.3832/ifor1100-008
Academic Editor
Paolo Cherubini
Paper history
Received: Aug 07, 2013
Accepted: Apr 28, 2015
First online: Sep 28, 2015
Publication Date: Apr 26, 2016
Publication Time: 5.10 months
Copyright Information
© SISEF - The Italian Society of Silviculture and Forest Ecology 2015
Open Access
This article is distributed under the terms of the Creative Commons Attribution-Non Commercial 4.0 International (https://creativecommons.org/licenses/by-nc/4.0/), which permits unrestricted use, distribution, and reproduction in any medium, provided you give appropriate credit to the original author(s) and the source, provide a link to the Creative Commons license, and indicate if changes were made.
Web Metrics
Breakdown by View Type
Article Usage
Total Article Views: 50335
(from publication date up to now)
Breakdown by View Type
HTML Page Views: 43108
Abstract Page Views: 2138
PDF Downloads: 3846
Citation/Reference Downloads: 18
XML Downloads: 1225
Web Metrics
Days since publication: 3354
Overall contacts: 50335
Avg. contacts per week: 105.05
Article Citations
Article citations are based on data periodically collected from the Clarivate Web of Science web site
(last update: Feb 2023)
Total number of cites (since 2016): 6
Average cites per year: 0.75
Publication Metrics
by Dimensions ©
Articles citing this article
List of the papers citing this article based on CrossRef Cited-by.
Related Contents
iForest Similar Articles
Research Articles
The effects of fire on Pinus sylvestris L. as determined by dendroecological analysis (Sierra de Gredos, Spain)
vol. 15, pp. 171-178 (online: 09 May 2022)
Review Papers
Tectona grandis Linn. f. secondary metabolites and their bioactive potential: a review
vol. 15, pp. 112-120 (online: 26 March 2022)
Research Articles
Density management diagram for teak plantations in Tabasco, Mexico
vol. 10, pp. 909-915 (online: 01 December 2017)
Research Articles
Study on the chemical composition of teak wood extracts in different organic solvents
vol. 14, pp. 329-336 (online: 09 July 2021)
Research Articles
The effect of provenance of historical timber on tree-ring based temperature reconstructions in the Western Central Alps
vol. 13, pp. 351-359 (online: 25 August 2020)
Research Articles
Heuristic forest planning model for optimizing timber production and carbon sequestration in teak plantations
vol. 10, pp. 430-439 (online: 24 March 2017)
Research Articles
Nutrient accumulation and export in teak (Tectona grandis L.f.) plantations of Central America
vol. 8, pp. 33-44 (online: 04 June 2014)
Review Papers
Climate change impacts on spatial distribution, tree-ring growth, and water use of stone pine (Pinus pinea L.) forests in the Mediterranean region and silvicultural practices to limit those impacts
vol. 14, pp. 104-112 (online: 01 March 2021)
Research Articles
Modifying harvesting time as a tool to reduce nutrient export by timber extraction: a case study in planted teak (Tectona grandis L.f.) forests in Costa Rica
vol. 9, pp. 729-735 (online: 03 June 2016)
Research Articles
Tree-ring-based reconstruction of larch budmoth outbreaks in the Central Italian Alps since 1774 CE
vol. 12, pp. 289-296 (online: 27 May 2019)
iForest Database Search
Search By Author
Search By Keyword
Google Scholar Search
Citing Articles
Search By Author
Search By Keywords
PubMed Search
Search By Author
Search By Keyword