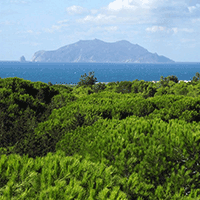
Climate change impacts on spatial distribution, tree-ring growth, and water use of stone pine (Pinus pinea L.) forests in the Mediterranean region and silvicultural practices to limit those impacts
iForest - Biogeosciences and Forestry, Volume 14, Issue 2, Pages 104-112 (2021)
doi: https://doi.org/10.3832/ifor3385-013
Published: Mar 01, 2021 - Copyright © 2021 SISEF
Review Papers
Abstract
Stone pine (Pinus pinea L.) has been cultivated since centuries in Mediterranean areas for its products and economic benefits, including edible pine nuts, timber, mushrooms, firewood, and grazing. However, current management objectives of stone pine stands also include recreational use, biodiversity conservation, protection from soil erosion, and CO2 fixation. Stone pine stands are considered to be among the ecosystems most vulnerable to climate change, and the current increase in drought frequency in the Mediterranean Basin has been shown to negatively impact their long-term establishment. Understanding the effects of climate change on the distribution, tree-ring growth and water use of stone pine forests can help assessing the adaptive capacity of the species, and developing management programs aimed at its conservation. This paper reviews the impacts of climate change on stone pine in the Mediterranean region. The high sensitivity of stone pine to climate change has been widely demonstrated in that: (i) climatic models predict the loss of suitable habitats and the shift of its geographical distribution in the next future; (ii) tree-ring analysis showed that winter and spring rainfalls have positive effects on growth, whereas high spring temperature has a negative effect; (iii) the strategy of stone pine to cope with water deficit affects the processes regulating its growth, including wood formation, leading to peculiar tree-ring anatomical features such as intra-annual density fluctuations. The silvicultural interventions and the most effective management strategies for stone pine forests are reviewed and discussed in the context of current climate change in the Mediterranean Basin.
Keywords
Stone Pine, Climate Change, Spatial Distribution, Tree-ring, Silvicultural Practices, Mediterranean Area
Introduction
Climatic models predict an increase in aridity in many parts of the world during the twenty-first century, which is likely to be more intense in the Mediterranean Basin than in other regions ([50]). Climate forecasts for the Mediterranean region anticipate increasing temperatures, decreasing rainfall, longer dry spells, more frequent heat waves, and heavier precipitation events, all of which will exacerbate the existing problems of soil loss and desertification ([44]). The strong irregularity of rainfall in the Mediterranean area, which can trigger severe/extreme droughts and floods, results in a high plant vulnerability to interannual rainfall variability and extreme event occurrence ([34]). Mediterranean forests provide a variety of goods (timber, firewood, and mainly non-wood forest products such as pine nuts, cork, aromatic plants, game, and mushrooms) and high-value services (recreation, protection from erosion, livestock grazing, biodiversity conservation, CO2 sequestration, and water balance regulation), which are important for ensuring the maintenance of human society. Multifunctionality is therefore an important characteristic of Mediterranean forest management ([72]).
Sensitivity to climate change and anthropogenic disturbances are common features of Mediterranean forests, which grow under dynamic and manipulated environmental conditions ([24]). Many of the projected changes in climate, as well as the indirect effects of these changes, are likely to have a negative impact on forest systems ([42]). The current increase in drought frequency has been shown to detrimentally affect the long-term establishment of Mediterranean forests. Among other effects, an increase in drought events might have an adverse impact on plant regeneration ([28]). Freire et al. ([34]) attest that the ongoing climate change is causing a decrease in rainfall over all seasons, and especially in the spring during cone growth, and it is more severe in the most critical regions for stone pine. Correspondingly, the increase in temperature, mainly in summer, leads to the death of cones with one or two years of maturation. Dendroecological studies in the Mediterranean basin have demonstrated that tree-ring data can be used as climate proxies in this region ([36], [88]). Tree-ring widths usually show variation over the life of a tree; therefore, such age/size trends need to be estimated and removed from time series in order to detect the evidence of climatically-driven tree growth. Regarding the interdependence of increasing temperatures and decreasing water availability, Sánchez-Salguero et al. ([80]) showed that growth is more sensitive to drought than to temperature in several Mediterranean species, which is in accordance with the findings by Calama et al. ([14]). Understanding the effects of climate change on the ecology of Pinus pinea forests can help to assess the adaptive capacity of the species, and develop management programs aimed to ensure the conservation of its populations ([67]).
The study of current and future potential distribution of suitable habitats for stone pine under climate change is critical ([3]). It is well known that climate plays a determining role in the large-scale distribution of species ([39]). Species distribution models (SDMs) are used to determine the ecological niche of species, and estimate their potential distribution ranges in terms of regional ecology and biogeography ([32]). Projections of future climate conditions in the Mediterranean Basin predict substantial changes, such as increased average temperature, reduced precipitation, and alterations in annual patterns ([91]).
In this context, it is important to understand the impact of these changes on the distribution of stone pine in the Mediterranean forests by spatial modeling, as well as growth responses to changes in precipitation and temperature through tree-ring analyses, and the strategies of the species to cope with water deficit, in order to introduce silvicultural practices to limit these impacts. The objective is to use the available results of research to provide guidance for the management of stone pine stands established in the Mediterranean region in the context of ongoing climate changes.
Importance of stone pine stands in Mediterranean forests
This review focuses on an emblematic Mediterranean species, stone or umbrella pine (Pinus pinea L.), a native/naturalized species to Southern Europe ([78]) spread from the Portuguese Atlantic coast to the shores of the Black Sea and the slopes of Mount Lebanon (Fig. 1), from sea level to 1000 m, and occasionally up to 1600 m a.s.l. ([78]). Stone pine is widely present in coastal habitats, where it has extensive ecological, landscape, recreation, and soil conservation uses. According to Mutke et al. ([63]), stone pine forests are estimated to cover 0.7 million ha across the Mediterranean region (Fig. 2). The most important distribution areas are in Spain (470,000 ha), Portugal (80,000 ha), Turkey (50,000 ha), and Italy (40,000 ha). More than half of this area is the result of protective afforestation ([54]).
Fig. 1 - Main distribution of Mediterranean stone pine forests ([19]).
Stone pine plantation dates back to the Roman period, and it was traditionally cultivated for timber and pine nuts. However, its recreational and landscape value has increased in recent decades ([56]), and is currently used for the consolidation of sand dunes and protect soil from erosion ([24]). Indeed, its multi-layered root system allows mature trees to extract water at different depths and from various sources ([23]). Along the Italian coastline, Pinus pinea forests have an important environmental and historical value; the species has been systematically planted here since ancient times, mostly for cone and wood production, as well as for coastline protection ([4]).
Pinus pinea forests are usually managed as multifunctional forests that provide timber, biomass, non-wood forest products (such as pine nuts and truffles - [11]), soil protection, sand dune stabilization, biodiversity refuge, space for public and recreational activities, and carbon sequestration ([54]). Yet, the most profitable activity for forest owners is cone production, more than timber and other products ([69]). Given the high nutritional value and the unique flavor of stone pine nuts, their demand is globally increasing, with the consequent increase in market price ([30]). According to Loewe & Delard ([49]), the prices of Pinus pinea edible nuts has increased from €25 kg-1 in 2010 to €45 kg-1 in 2013. Stone pine kernel production in Italy ranges between 40 and 120 kg ha-1, which is equivalent to 10 to 30 kg of pine nuts ha-1 ([62]). Moreover, Ovando et al. ([69]) showed that stone pine afforestation offers positive net benefits to landowners, taking into account carbon prices of up to 45 € tC-1 (12.3 € tCO2-1). Furthermore, stone pine has proven to be a suitable species in combined agroforestry systems for both nuts and timber production, intercropped with agricultural crops, and animal grazing ([49]). Those authors found that the annual income derived from crops and animals is relevant for the household economy, especially during the first years.
Recently, Calama et al. ([15]) reported a generalized decline in the kernel-per-cone yield in Pinus pinea forests located in different Spanish regions, revealing reductions of up to 50% in the final kernel-per-cone yield in the most drought affected regions. Such reduction over different years and provenances with contrasting climates suggests the implication of a biotic factor that can be aggravated in extreme drought years. Further, Natalini et al. ([67]) suggested that the Pinus pinea species has a plastic response to warmer and drier climates that can vary among populations, and some measure of such variability should be considered in long-term forecasts of vegetation dynamics.
Spatial modelling of stone pine distribution under climate change
Akyol & Orucu ([3]) found that the most important bioclimatic variables affecting the potential distribution of P. pinea are the minimum temperature of the coldest month (Bio6), annual precipitation (Bio12), and precipitation of the wettest quarter (Bio16); whereas, Serra Varela ([82]) found that annual mean temperature (Bio1), temperature seasonality (Bio4), precipitation seasonality (Bio15), precipitation of the warmest quarter (Bio 18), and precipitation of the coldest quarter (Bio 19) are the main drivers of stone pine distribution. Simulation models under two different future climate change scenarios predicted that P. Pinea will lose suitable habitats and will shift toward northern and higher elevation sites. For example, Akyol & Orucu ([3]) showed that P. pinea in Turkey will shift its geographical distribution in the future and experience losses of habitat, particularly in the western and southern parts of Turkey. According to Akyol & Orucu ([3]), the potential distribution of P. pinea in the years 2050 and 2070 will decrease under the representative concentration pathway (RCP) 4.5 and RCP 8.5 scenarios. Pecchi et al. ([73]) reported that by 2050 under RCP 4.5 scenario, the spatial distribution of suitable habitats for stone pine pure stands will significantly decrease in Italy compared to other hardwood species.
Freire et al. ([34]) reported that the impacts of medium-term climate change on Mediterranean forests are the displacement and migration of species from south to north, and from inland to the coast ([35]). According to Lopez-Tirado & Hidalgo ([50]), stone pine could expand its potential area in southern Spain by approximately 17%. Overall, stone pine would be the most suitable pine species in the western and northeastern zones of the study area. Similar results were obtained by Bede-Fazekas et al. ([9]), who stated that most of the distribution of stone pine in Spain will remain viable by the middle twenty-first century.
Calama et al. ([12]) analyzed the spatial and temporal patterns of variability in cone production in stone pine forests located in the Spanish Northern Plateau. Those authors found that the annual production of stone pine cones at the tree level showed a skewed and zero-inflated distribution, together with a large interannual variability, with a regional average ranging from 0.2 to 6.3 kg tree-1. This variation was largely synchronized among the trees and stands throughout the study area, confirming the masting habit of the species. Apparently, climatic factors are the main determinants of the masting behavior, explaining about 60%-80% of the temporal variation in cone production at the regional scale over a series of 13 years, correctly discriminating between good and bad crop years.
Temperature and precipitation effects on tree-ring growth
In a study on the variation of stone pine productivity in relation to climate in Spain, Natalini et al. ([65]) found that winter and spring rainfall has a positive effect on growth, whereas high spring temperature negatively affects stone pine growth. These authors found that growing performances are positively correlated with the Palmer Drought Severity Index (PDSI) and annual rainfall, and negatively correlated with the maximum average annual temperature, which agrees with the findings of Loewe et al. ([48]) for height growth in Chile.
Precipitation effects
Dendroclimatological analyses indicate that the positive moisture balance in the spring is the main factor favoring the radial growth of Pinus spp. in the Mediterranean regions ([77]). Winter precipitation is largely responsible for growth rates of stone pine ([85], [86]), and the high sensitivity of growth to precipitation has been confirmed by Raventos et al. ([79]). Loewe et al. ([47]) identified climatic variables (e.g., annual water deficit) that significantly influence seed number per cone in stone pine, and also the biometeorological variables that account for the physiophenological phases involved in this trait determination. Akkemik ([2]) found that precipitation in the current year has a significantly positive influence on the tree-ring growth, and observed a significant positive relationship between tree-ring width and monthly precipitation. Cherubini ([20]) concluded that precipitation plays the most important role in tree-ring growth in Pinus pinea L. Calama et al. ([14]) detected a positive relationship between rainfall and growth, extending back to the precipitation of previous autumn and winter seasons. Similar findings have been reported in previous studies on dendroecological growth-climate relationships for the species ([57], [66], [67], [41]). Total rainfall occurring during the period from October of the previous year to September of the current growth year as well as mean temperature in May and June, are the main climatic factors driving secondary growth in Pinus pinea ([14]). Novak et al. ([68]) reported that dry conditions during January to May and high temperatures during late winter and spring were the main climatic factors promoting missing rings in Pinus pinea. This has suggested that the observed increase in missing rings could be related to stress conditions caused by reduced precipitation ([25]). El-Khorchani et al. ([29]) also noted the impact of hydric balance on diameter growth, and water stress can be intensified in sandy soils, where water availability decreases drastically in dry years ([84]). Accordingly, Mazza & Manetti ([58]) reported that low precipitation is the main factor causing a decrease in radial growth, an effect that lasts over long periods. Mazza et al. ([57]) showed a clear grouping of P. pinea tree ring growth related to regional variation in climatic features, which likely reflects the existence of different responses strongly dependent on geographical variability in rainfall inputs during the periods that most influence tree ring formation. The decrease in winter-early spring rainfall appeared to be the principal climate-driven growth pattern distinguishing the chronologies.
Temperature effects
The most important environmental variable affecting survival and distribution of Pinus pinea in the Mediterranean is the average temperature of the coldest month ([85], [86]). Loewe et al. ([47]) showed that temperature has the highest impact on stone pine growth; in particular, vertical growth was more than twice in sites with low annual average temperature and high winter thermal oscillations. Tree ring development in P. pinea is negatively influenced by drought in the late spring and summer (from March to September) and enhanced by a positive moisture balance in the previous winter season, as reflected by the positive relationship between radial growth and Palmer Drought Severity Index (PDSI) values ([70], [77]). Previous studies indicated a close relationship between stone pine radial growth and climatic factors, highlighting that tree ring formation in this species is sensitive to drought ([79], [25], [68], [57]). Thus, tree ring analysis in this species can be potentially useful in climate change studies in the Mediterranean basin, which is considered particularly vulnerable to climate alterations ([37]).
Akkemik ([2]) found that the temperature at the beginning of the growing period can have a significantly positive effect on tree ring growth, and concluded that Pinus pinea L. is a dendroclimatologically sensitive species. P. pinea has a drought-tolerant strategy, with a strongly reduced photosynthetic activity under water stress and elevated vapor pressure deficits ([5]), as commonly observed in summer and early autumn ([83]). These events might be detrimental for the radial growth of the species, inducing a decline of natural and artificial populations across most of its current range ([77]). Thuiller et al. ([85]) highlighted the importance of extreme temperature in the ability of this species to survive and grow, thus affecting its geographical distribution. A negative relationship between temperature and growth has previously been observed in this species ([67]), as well as in other Mediterranean pines ([53]). Natalini et al. ([65]) also stressed the species vulnerability under increasing temperature due to climate change. Consequently, heat tolerance should be considered a key breeding trait for enhancing growth performances of stone pine. In Tunisia, Thabeet et al. ([84]) reported a negative correlation between average temperature and growth, but with a superior threshold (16 °C). Novak et al. ([68]) found that dry conditions from January to May, and high temperatures during late winter and spring were the main climatic factors promoting missing rings in Pinus pinea. Despite similarities, Pinus halepensis has proven to be more sensitive to drought than P. pinea early along the growing season, whereas the latter seems to be more sensitive to temperature variations during winter. Additionally, in both species, we found that the determining temperature has increased in recent periods. Late winter and spring maximum daily temperature values, associated with high evapotranspiration, were shown to negatively affect cell enlargement. However, minimum temperature was positively related to tracheid lumen size just before the period of active xylem formation. Moreover, Pinus pinea is sensitive to freezing temperatures ([1]).
Water use by stone pine forests
Pinus pinea is is a drought-avoiding isohydric species with a shallow root system which is able to reduce water flow during aridity and to recover when water becomes available again ([55], [90]). The contrasting physiological strategies of Pinus pinea to cope with water deficit influence the regulation of growth processes, such as wood formation, leading to peculiar tree-ring anatomical features such as intra-annual density fluctuations ([89]). In a study focused on anatomical traits and composition of carbon and oxygen stable isotopes in the intra-annual density fluctuations (IADFs) occurring in tree rings, Zalloni et al. ([89]) showed that the period of formation of IADF is autumn and the influence of climate on IADF occurrence is species-specific. Natalini et al. ([67]) found that the growth-climate correlations varied by site and period. Intra-annual density fluctuation in stone pine has been related to the alternation of drought and rainy periods either in summer or autumn ([17], [64], [90]). The tree-ring growth response of Pinus pinea during winter, previously reported for Spain and Italy ([25], [57], [67]), may reflect the physiological activity of this species during winter ([71]). Balzano et al. ([6]) found that in Mediterranean trees, cambium often produces several bands of alternating early- and latewood during a calendar year, resulting in the formation of IADFs. Tree-ring analysis in Pinus pinea showed a IADF region enriched in δ 13C with an increasing trend in δ 18O, which suggests stomatal closure, and the decrease in stomatal conductance likely contributed to the increase in δ13C at the IADF level ([26]). The high values of δ13C and δ18O, along with the larger tracheids in the IADF region of Pinus pinea, suggest an enhancement of conductive efficiency leading to strict stomatal control with the aim of avoiding dehydration ([8]). According to Balzano et al. ([6]), stone pine trees on the Vesuvius Mountain (near Naples, Italy) showed uninterrupted wood production from January 2015 until the end of January 2016. The authors showed differences in cambium activity in late autumn among different years; in November 2015 and 2018, the cambium was still active and no longer active, respectively. Since radial growth generally starts at the treetop, the decreased availability of carbohydrates may cause reduced wood formation in the lowermost part of the tree. This was particularly noticeable in the Pinus halepensis, but not in P. pinea ([68]).
Pinus pinea earlywood anatomical features are the result of the typical double constraint of Mediterranean climate on tree growth: water shortage and, to a lesser extent, low winter temperature ([20]). Based on records of annual growth rate, the response of stone pine to climate over the last 50 years also suggests the influence of precipitation and temperature regimes on growth ([24]). These variables affect the soil water balance and are taken as the main factor driving tree growth decline, with a cumulative effect over consecutive years ([56]). Mazza & Manetti ([58]) demonstrated the dependence of the species on long periods of water supply and its capacity of using the water content stored in previous rainy years. High air temperatures stimulate evapotranspiration and water loss ([7]), thus leading to the drought-avoidant response and reduced stomatal conductance ([89]), as reflected by the presence of IADFs and high values of δ13C and δ18O in the wood of P. pinea. Intra-annual density fluctuations in Pinus pinea tree rings were also suggested to be formed during autumn months, as shown by the significant correlation found between IADF δ18O values and mean temperature ([17], [64], [90]).
According to Castagneri et al. ([18]), xylem anatomy of Pinus pinea is strongly related to the environmental conditions occurring in the previous season, particularly regarding tree-ring carbon composition, but it is not affected by summer drought. Indeed, earlywood is likely formed using both recently and formerly assimilated carbon, while latewood mostly relies on carbon accumulated many months prior to its formation. The direct relationship between xylem formation and intra-seasonal precipitation distribution is reflected in the intra-ring pattern of lumen size; earlywood tracheids are influenced by precipitation occurring before and during xylogenesis ([16], [88]); latewood is mostly formed after the harsh summer period, when sandy soil is dry, photosynthesis is reduced ([13], [31]), and the limited amount of photosynthates available is used for metabolic functions ([27]). Under these conditions, xylem formation may mostly depend on reserves. Furthermore, latewood δ13C was minimally associated with climatic conditions immediately before or during its formation, but was highly associated with the previous year δ13C and with temperature occurring several months before. Therefore, we suppose that the carbon used used for latewood formation came from mixing pools of different ages, in part older than one year ([87]).
Managing stone pine forests under climate change
Rainfall reduction and temperature increase are challenging to stone pine management for cone production in the Mediterranean climate region. Therefore, it is necessary to adapt traditional silvicultural guidelines for stands facing climate change consequences, by reducing stand density over their lifetimes in order to ensure tree production in healthy mixed even-aged stands. In a study of the impact of climate and management variables in stone pine, Pereira et al. ([74]) proposed a plantation management model for timber production (Tab. 1). Loewe et al. ([47], [48]) reported that stone pine fertilization applied during two consecutive years is a useful tool to enhance DBH and height, and one year-old conelet production. These results confirm the benefits of establishing fertilized plantations for increasing growth and fruit production. Irrigation had an important effect on fruiting, but the effects on radial growth were not significant. Contrastingly, the irrigation effect was positive on both height and DBH growth when combined to fertilization (fertirrigation) in a 75 year-old plantation of limited growth and no management established in a clay-sand soil ([75]), with increases from 2 to 15 mm in diameter. Loewe et al. ([47]) observed a positive effect on vertical growth, in agreement with the findings reported by Loewe & Delard ([46]). Freire et al. ([34]) highlighted the importance of maintaining a small ratio between tree height and diameter, as it makes trees less susceptible to cavitation due to the reduced effort to transport water from the soil to leaves, as well as capable of maintaining their stomata open for longer periods, thereby increasing carbon assimilation for growth and cone production.
Tab. 1 - Model of plantation management for timber production. Source: Pereira et al. ([74]) in Sbay & Hajib ([81]).
Age (years) | Operations and density ha-1 |
---|---|
0 | 850 trees ha-1 |
10 | Thinning (41 % cut : remains 500 trees ha-1) |
20 | Pruning mainly low branches |
40 | Thinning (45 % cut : remains 225 trees ha-1) |
80 to 150 | Final cut (rotation) leaving 10 trees ha-1 (seed trees) |
Forestry aimed to promote adaptation to climate change is based on the implementation of practices aimed to reduce vulnerability, and increase the adaptive capacity of forest species in relation to the most limiting factor in the Mediterranean area, i.e., water deficit ([35]). To achieve this objective, each individual tree must keep the highest possible vigor in order to be less susceptible to pathogen attacks and more resistant to drought events ([43]). Traditionally, Pinus pinea stands have been managed following a uniform shelterwood system, with a three stage regeneration cut, and two or three thinnings during rotation ([61], [74]). Freire et al. ([33]) described silvicultural interventions appropriate for a stand of pine nuts (Tab. 2). Thinning regulates intra-specific and interspecific competition ([14]), thus increasing the availability of water and nutrients for each tree. Also, thinning favors soil parameters ([56]) and tree characteristics ([60]), and mitigates the negative effects of drought ([35], [38]). According to McDowell et al. ([59]), thinning promotes physiological responses such as photosynthetic rate, carbon assimilation, and stomatal conductance, and reduces the effect of pests and diseases ([21]), promoting the stability of stands by reducing the height/diameter ratio of trees ([35], [10]), which is considered a mitigation measure against the detrimental effects of climatic changes ([56]). Freire et al. ([34]) proposed an optimal stand density, maximum crown diameter, cone number, weight per tree, and average cone production per ha (Tab. 3). In Tunisia, systematic thinning is applied in certain stands (Fig. 3) and sometimes the final cut, in silvicultural interventions (Fig. 4).
Tab. 2 - Silvicultural systems for stone pine (source: [33]).
Operation | Correia & Oliveira ([22]) | Louro et al. ([51]) | INRB ([40]) |
---|---|---|---|
Initial density | 625 trees ha-1 | 500 to 600 trees ha-1 | 208 to 400 trees ha-1 |
Pruning | Removal of branches without female flowers (without indication of periodicity) | 1/3 branches removal: | 1/3 till 2/3 branches removal: |
Between 8 and 12 years | Between 5 and 6 years | ||
Between 20 and 25 years | Between 10 and 12 years | ||
Removal of branches without female flowers: | Between 20 and 25 years | ||
Between 35 and 40 years | - | ||
Between 50 and 60 years | - | ||
Thinning | At 10 years till 500 trees ha-1 | 400 trees ha-1 | Between 10 and 12 years |
At 15 years till 300 trees ha-1 | Between 20 and 25 years till 352 trees ha-1 | Between 20 and 25 years | |
At 31 years till 100 trees ha-1 | Between 25 and 30 years till 281 trees ha-1 | - | |
- | Between 35 and 40 years till 225 trees ha-1 | No information on density after thinning | |
Final density | 100 trees ha-1 | 225 trees ha-1 | Between 100 and 120 trees ha-1 |
Mean Distance | 10 m | 6.7 m | Between 10 and 9.2 m |
Regeneration cut (rotation) | At 80 years | Between 80 and 100 years | No information about the regeneration cut |
Tab. 3 - Proposals for final stand density (N), maximum crown diameter (Mcw), cone number (Mnc), weight (Mwc) per tree and average cone production per ha (Wc) ([34]).
N (trees ha-1) |
References | Mcw (m) |
Mnc (m) |
Mwc (kg) |
Wc (kg ha-1) |
---|---|---|---|---|---|
28 | Freire et al. ([33]) | 18.9 | 2136 | 500.4 | 1124.3 |
75 | Kuçuker & Baskent ([45]) | 11.6 | 1871 | 322.4 | 604.5 |
78 | Pique-Nicolau et al. ([76]) | 11.3 | 400 | 117.8 | 582.7 |
100 | Pereira et al. ([74]) | 10.0 | 349 | 90.4 | 422.7 |
120 | INRB ([40]) | 9.1 | 349 | 90.4 | 277.2 |
150 | Manso et al. ([52]) | 8.2 | 349 | 90.4 | 243.6 |
225 | Louro et al. ([51]) | 6.7 | 92 | 22.4 | 196.8 |
Conclusions
Stone pine (Pinus pinea L.) is naturally distributed in the Mediterranean forest ecosystem, and is frequently employed in afforestation practices because of its ecological, economic, and aesthetic characteristics. It is among the main species that provide an important contribution to the national economy, especially in terms of non-wood products. Climate change has negative effects on stone pine forests that have limited suitable habitats currently threatened by climatic change. Climatic models using different future scenarios predict that P. pinea will undergo habitat loss, thus shifting its geographical distribution. Tree-ring analysis showed that winter and spring rainfall has positive effects on growth, whereas high spring temperature has the opposite effect. Stone pine growth is positively correlated with the Palmer Drought Severity Index (PDSI) and with annual rainfall, and negatively correlated with the maximum average annual temperature.
Forest management could play a fundamental role in reducing the potential impact of climate change on forest ecosystems. Good management of stone pine forests and plantations should ensure effective adaptation to climate change. Silvicultural practices should be aimed at increasing species richness, favoring hardwoods currently growing under conifer canopy, as well as stimulating natural regeneration and gene flow. Special attention should be given to support migration processes, given the predicted shift of suitable habitats for the species.
Conflict of interest
The authors declare that they have no conflict of interest.
Acknowledgments
The authors would like to thank the Faculty of Science, Princess Nourah Bint Abdurrahman University, Riyadh (Saudi Arabia) and Silvo-Pastoral Institute of Tabarka, University of Jendouba, Jendouba (Tunisia).
References
Online | Gscholar
CrossRef | Gscholar
CrossRef | Gscholar
Gscholar
Gscholar
CrossRef | Gscholar
Online | Gscholar
Gscholar
Gscholar
Gscholar
Gscholar
Gscholar
Gscholar
Gscholar
Gscholar
Gscholar
Gscholar
Gscholar
Gscholar
Gscholar
CrossRef | Gscholar
Gscholar
Gscholar
Gscholar
Authors’ Info
Authors’ Affiliation
Wahbi Jaouadi
Silvo-Pastoral Institute of Tabarka, B.P 328, 8110 Tabarka, University of Jendouba (Tunisia)
Wahbi Jaouadi
Souheila Naghmouchi 0000-0001-5093-810X
National Institute of Research in Rural Engineering, Waters and Forests, BP 10, Hédi Karray Street, Menzeh IV, Ariana 2080, University of Carthage (Tunisia)
Biology Department, College of Science Princess Norah Bint Abdulrahman University, Riyadh (Saudi Arabia)
Corresponding author
Paper Info
Citation
Mechergui K, Saleh Altamimi A, Jaouadi W, Naghmouchi S (2021). Climate change impacts on spatial distribution, tree-ring growth, and water use of stone pine (Pinus pinea L.) forests in the Mediterranean region and silvicultural practices to limit those impacts. iForest 14: 104-112. - doi: 10.3832/ifor3385-013
Academic Editor
Silvano Fares
Paper history
Received: Feb 25, 2020
Accepted: Dec 28, 2020
First online: Mar 01, 2021
Publication Date: Apr 30, 2021
Publication Time: 2.10 months
Copyright Information
© SISEF - The Italian Society of Silviculture and Forest Ecology 2021
Open Access
This article is distributed under the terms of the Creative Commons Attribution-Non Commercial 4.0 International (https://creativecommons.org/licenses/by-nc/4.0/), which permits unrestricted use, distribution, and reproduction in any medium, provided you give appropriate credit to the original author(s) and the source, provide a link to the Creative Commons license, and indicate if changes were made.
Web Metrics
Breakdown by View Type
Article Usage
Total Article Views: 37404
(from publication date up to now)
Breakdown by View Type
HTML Page Views: 31360
Abstract Page Views: 2572
PDF Downloads: 2962
Citation/Reference Downloads: 7
XML Downloads: 503
Web Metrics
Days since publication: 1467
Overall contacts: 37404
Avg. contacts per week: 178.48
Article Citations
Article citations are based on data periodically collected from the Clarivate Web of Science web site
(last update: Mar 2025)
Total number of cites (since 2021): 12
Average cites per year: 2.40
Publication Metrics
by Dimensions ©
Articles citing this article
List of the papers citing this article based on CrossRef Cited-by.
Related Contents
iForest Similar Articles
Review Papers
Impacts of climate change on the establishment, distribution, growth and mortality of Swiss stone pine (Pinus cembra L.)
vol. 3, pp. 82-85 (online: 15 July 2010)
Research Articles
Impact of climate change on tree-ring growth of Scots pine, common beech and pedunculate oak in northeastern Germany
vol. 9, pp. 1-11 (online: 13 October 2015)
Review Papers
Should the silviculture of Aleppo pine (Pinus halepensis Mill.) stands in northern Africa be oriented towards wood or seed and cone production? Diagnosis and current potentiality
vol. 12, pp. 297-305 (online: 27 May 2019)
Research Articles
Predicting the effect of climate change on tree species abundance and distribution at a regional scale
vol. 1, pp. 132-139 (online: 27 August 2008)
Research Articles
Tree-oriented silviculture: a new approach for coppice stands
vol. 9, pp. 791-800 (online: 04 August 2016)
Research Articles
Decline in commercial pine nut and kernel yield in Mediterranean stone pine (Pinus pinea L.) in Spain
vol. 13, pp. 251-260 (online: 03 July 2020)
Short Communications
Growth of Stone pine (Pinus pinea L.) European provenances in central Chile
vol. 10, pp. 64-69 (online: 29 August 2016)
Research Articles
Effects of traditional coppice practices and microsite conditions on tree health in a European beech forest at its southernmost range
vol. 9, pp. 673-681 (online: 12 March 2016)
Research Articles
Twenty years of conversion: from Scots pine plantations to oak dominated multifunctional forests
vol. 10, pp. 75-82 (online: 19 October 2016)
Research Articles
Scots pine’s capacity to adapt to climate change in hemi-boreal forests in relation to dominating tree increment and site condition
vol. 14, pp. 473-482 (online: 18 October 2021)
iForest Database Search
Search By Author
Search By Keyword
Google Scholar Search
Citing Articles
Search By Author
Search By Keywords
PubMed Search
Search By Author
Search By Keyword