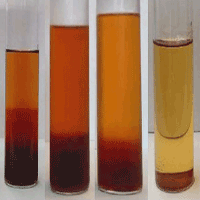
Tectona grandis Linn. f. secondary metabolites and their bioactive potential: a review
iForest - Biogeosciences and Forestry, Volume 15, Issue 2, Pages 112-120 (2022)
doi: https://doi.org/10.3832/ifor3714-015
Published: Mar 26, 2022 - Copyright © 2022 SISEF
Review Papers
Abstract
Tectona grandis Linn. f. (teak) is one of the most appreciated high-quality timber all over the world due to its economic value and wide array of applications. This tropical hardwood presents outstanding characteristics like pleasing aesthetic appearance, strength, lightness, ease of working, dimensional stability, and decay resistance. The latter quality is mainly ascribed to its extractives, which contain biologically active compounds (mainly quinones and anthraquinones) that confer a natural resistance against termites and fungi. This review focuses on teak secondary metabolites and the bioactivity potential of heartwood extractives. Furthermore, it covers the generalities of the teak tree and gives an overview on the approaches aimed to valorize the wastes from woodworking enterprises as a possible source of functional extractives and as an eco-friendly raw material. Notwithstanding the efforts made to elucidate the compounds present in teak wood, further research is needed to understand the chemical bases of its natural resistance to decay. Moreover, there is a lack of economic, technical, and ecotoxicity feasibility studies regarding extractives as a source of bioactive molecules for pharmaceutical, food, or cosmetics purposes.
Keywords
Extractives, Natural Resistance, Bioactivity, Secondary Metabolites, Teak Heartwood
Introduction
Tectona grandis Linn. f. (teak) is part of the high-value hardwood market and plays an essential role in the forest economy of many tropical countries. According to the Food and Agriculture Organization of the United Nations (FAO), teak is at the top priority of species to be conserved and managed due to its economic value, which includes timber, pulp food, wood energy, and non-forest products. Nonetheless, teak covers a minor share of world timber production and trade (less than 2% of total tropical woods - [42]).
Teak occurs naturally in India, Myanmar, Thailand, and Laos People’s Democratic Republic ([36]). However, it has been successfully established in other regions thanks to its wide tolerance to different climatic conditions. Teak planted forests have attracted investment from the private sector in several countries in Africa and Latin America ([26], [44]).
Teak timber shows exceptional resistance to weather, non-corrosive properties, high hardness, durability, small shrinkage, beautiful texture, and appearance ([36]). These characteristics make teak wood suitable for many purposes like luxurious furniture, deck, ship and boatbuilding, railway sleepers, construction industry, carving, veneer, and ornaments ([86]). Moreover, it is considered a meaningful constituent in traditional medicine ([76]). Among the effects attributed to their extractives are biocidal, allelopathic, therapeutic, and antioxidant ([88], [107]).
Several studies dealt with the identification, quantification, and evaluation of bioactive compounds from teak wood. This review summarizes the research related to teak secondary metabolites and the bioactivity potential of heartwood extractives, sorted by type of extraction solvent. It also highlights the valorization of teak residues and the potential use of teak secondary metabolites in the cosmetic or food industry. This work then proposes a resource for comparing and analyzing the work carried out, offering a structured overview, which is summarized in Fig. 1.
Fig. 1 - Graphic summary of the main sources of extractives from teak (Tectona grandis Linn. f.) wood and their applications.
Generalities
Taxonomic description
Tectona grandis Linn. f. is a deciduous tree of the Lamiaceae family. Tab. 1presents its taxonomic hierarchy according to the Integrated Taxonomic Information System, taxonomic serial no. 32247 ([32]). Three species belong to the genus Tectona, namely T. philippinensis Benth. and Hooker f., T. hamiltoniana Wall., and T. grandis Linn. f.
Tab. 1 - . Tectona grandis Linn. f. taxonomic hierarchy.
Kingdom: | Plantae |
Subkingdom: | Viridiplantae |
Infrakingdom: | Streptophyta |
Superdivision: | Embryophyta |
Division: | Tracheophyta |
Subdivision: | Spermatophytina |
Class: | Magnoliopsida |
Superorder: | Asteranae |
Order: | Lamiales |
Family: | Lamiaceae |
Genus: | Tectona |
Species: | Tectona grandis Linn. f. |
Tectona philippinensis (Philippine teak) is locally known as Malabayabas (Lobo, Batangas), Malapangit (Tagalog), and Bunglas (Panay Bisaya). It is endemic to the Philippines, occurring in Luzon Island and Iling Island. This tree is classified as an endangered species. Philippine teak may have potential as a genetic resource for future teak breeding programs aimed at improving supplies of this highly popular wood ([20]). It is a small to a medium-sized tree (up to 15 m) with thin and flaky bark and bright purple, bluish to lilac blooms ([11]). Timber is used in the construction industry, for building ships, bridges, heavy-duty furniture, and locally as firewood. In addition, its wood and leaves have medicinal value ([87], [7]). The trade of Philippine lumber is mainly domestic rather than international ([20]).
Tectona hamiltoniana (Dahat teak) is confined to the dry zone of central Myanmar like Magway and Mandalay. It naturally occurs in areas where annual rainfall is between 400 and 800 mm. It is, therefore, drought-tolerant. This species is a medium-sized deciduous tree of approx. 8 m in height. Dahat teak wood shows favorable properties, such as high stability and density, and very high natural resistance to insects. Furthermore, its bark is utilized for medical purposes. Nevertheless, it is economically not as important as Tectona grandis Linn. f. ([66]).
Tectona grandis Linn. f. is also known as sagwan, saigun, sagon and sagun (Hindi), sagavani (Kannada), kyun (Burmese), jati, deleg, and kulidawa (Malay, Javanese and Indonesian), sak and mai-sak (Lao, Sino-Tibetan), saguan and teak (Nepali) dalanang and djati (Filipino), teca (Spanish), teak tree (English), and teck (French, Italian). Tectona grandis is a large, deciduous tree that can reach a height from 20 to 35 m, and a diameter at breast height (DBH) from 29 to 54 cm in 50 years ([80]). The stem is straight with rough and fissured bark of brown color, usually cylindrical but becoming fluted and slightly buttressed at the base when mature. The roots are thick and often no deeper than 30 cm because they are sensitive to oxygen deficiency; however, they extend laterally up to 15 m from the stem ([78], [33]). The teak tree has an open crown with many small branches ([76]). Leaves are opposite, broadly elliptic, obovate, from 11 to 85 cm long and 6 to 50 cm broad, glabrescent above and stellate-pubescent below, at the base rounded to acute, in apex obtuse to acute, and for petiole stout from 5 to 6 cm long ([80], [33]). The teak tree commonly begins to flower six years after planting, but plentiful flowering occurs after 15 years ([80]). Flowers usually appear during the rainy season, and trees tend to flourish synchronously ([78]). Teak flowers are small (from 6 to 8 mm in size) and white or rarely purplish pink color. They occur in large inflorescences or panicles (from 20 to 90 cm), which initiate from terminal buds of stem and branch shoots. Teak fruit is a drupe, globose, from 5 to 20 mm in size, enclosed by an accrescent calyx with thick shaggy exocarp of matted hairs, epicarp inflated, spongy, and staellate pubescent, endocarp stony, 4-celled, seeds 1-4, oblong and exalbuminous ([80]).
Teak trees exhibit significant morphological differences due to genetic and environmental factors. Therefore, the provenance, natural forests or plantations, largely affect characteristics such as aesthetic qualities, texture, heartwood-sapwood ratio, mineral content, and resistance to pests and diseases ([44]).
Geographic requirements
Teak plantations are well grown in tropical and sub-tropical zones. It has a distribution range from the longitude of 73° E in India to 104° 30′ in Thailand. The northern boundary limit of teak is about 25° 30′ N in Myanmar; and its southern boundary limit lies from 9° N in India through 15°- 16° N in Myanmar to 16° 30′ N in Thailand ([36]). The altitude can vary from the sea level up to 1200 m a.s.l.
Teak plantations require well-drained alluvial soils, and quite moisty and tropical climate for good development. The best conditions for growing teak are soils derived from rocks of volcanic origin (pH between 6.5 and 7.5), temperatures between 13 and 40 °C, intensity of full day-light between 75% and 94%, and annual rainfall from less than 900 to 3500 mm ([36], [80]).
Geographic distribution
Natural forests
Natural teak forests are located in the Indian Peninsular, Myanmar, Thailand, and Laos People’s Democratic Republic ([36]). It is estimated that these forests cover approximately 29 million ha in these four countries. Teak wood from natural forests commercialized on the market comes exclusively from Myanmar, where teak plantations cover 16 million ha ([44]).
It is generally accepted that the quality of teak wood from virgin forests, where the rotation time may exceed 100 years, is distinctly superior to that from plantations. The exceptional decay resistance of teak wood from natural sites is worldwide appreciated ([108]). Unfortunately, native forests are declining, especially old-growth and high-quality reservoirs. Among the main causes are overexploitation, deforestation, agricultural expansion, grazing, and conversion to other land uses. As a result, the natural populations are endangered ([44]).
Although teak timber from natural forests represents just a minor share of the global trade, it is a relevant luxurious hardwood resource, emerging with an increasing growth rate, and this trend is likely to continue. In addition, the teak tree is easily adaptable to climatic conditions, and it needs short rotations. For these reasons many countries in tropical Asia, Africa, and Latin America are making investments in teak plantations ([9], [43]). The private sector manages 88% in Central America and 99% in South America ([42]).
Planted forests
It is assumed that Tectona grandis was exported for the first time from its endemic occurrence to Java, Indonesia, between the fourteenth and sixteenth centuries. In Africa, the introduction of teak in trial gardens was attributed to the German colonial administration in the nineteenth century. It is supposed that the African landraces came from India, Myanmar, or Thailand ([105]). Regarding Latin America, the first pure teak plantation was established in 1913 in Trinidad and Tobago with seeds coming from Tenasserim, Myanmar. However, it was until 1928 when a definitive planting program in Trinidad and Tobago started. In 1926, another introduction was carried out in Panama with seed from Sri Lanka ([37]).
Teak planted forests expand in around 70 tropical countries. According to various assessments, they cover between 4.35 and 6.89 million ha (1.1% of the world’s plantations), of which more than 80% grow in Asia, 10% in Africa, and 6% in tropical America. Nevertheless, these figures may underestimate the real planted area as there is no available data in all countries with teak plantations ([44]). It is noticeable that about 77% of planted teak forests are younger than 20 years.
Currently, this species reaches 74% of the planted area of tropical hardwoods, representing the most grown species of this category, which also includes mahogany (Swietenia macrophylla), red cedar (Cedrela odorata), and rosewood (Dalbergia sissoo). The establishment of teak plantations is expected to increase in the next years, and taking into account the decrease of teak supply from natural forests, timber from plantations is a promising source for the long-term market ([9]).
T. grandis extractives
Secondary metabolites (SM), also known as extractives in the context of forest products ([13]), are non-structural chemical compounds produced through metabolic pathways derived from the primary metabolic pathways. The secondary metabolites are classified according to their biosynthetic routes and structure and are classified into four major groups: phenolic compounds, terpenes, nitrogen-containing compounds and sulfur-containing compounds ([79], [34] - Fig. 2).
Unlike primary metabolites (e.g., fatty acids, proteins, carbohydrates, and nucleic acids), secondary metabolites are not involved in primary functions like cell development, or reproduction, but in the interaction of the cell with its environment. They are essential to plant survival ([106]), acting in the protection and defense against biotic (e.g., attack by insects and pathogenic microbes) or abiotic (e.g., drought and competition) stress ([79], [30]). Trees defend against insects and pathogens using both constitutive SM, always present, to avoid initial attack and induced SM to limit damage after an attack ([30]).
Many authors have identified a direct relationship between wood durability and extractives content. The content of teak extractives depends on environmental and genetic factors, radial position, and tree age ([58], [75]). Regarding radial position, some works indicate that the concentration of extractives is higher in heartwood than in sapwood. Normally, it increases radially towards the outer heartwood. Nonetheless, the sapwood and the transition zone between them also contain some chemicals ([101], [73]). For instance, Niamké et al. ([73]) have identified tectoquinone and hydroxycinnamic in the sapwood. They claim that the main teak extractives are synthesized in the aging sapwood (transition zone). The differences recognized along the radial position were attributed to the possible transformation of these compounds during the heartwood formation and maturation process as in other species. In addition, Kokutse et al. ([41]) reported that the inner teak heartwood is less resistant to pathogen attack than the intermediate or outer heartwood. This reduction in durability may be due to a decrease in extractives present in the heartwood nearer the pith, or an aging of these extractives.
Concerning the age of the tree, there is a general perception of a positive correlation between the tree age and the amount of total extractives ([29], [101]).
Secondary metabolites in T. grandis heartwood
Tab. S1 (Supplementary material) displays the secondary metabolites identified in Tectona grandis heartwood and the solvents used for their extraction. The predominant compounds are naphthoquinones and anthraquinones ([107]). Up to 691 metabolites have been identified in teak wood (both primary and secondary). Compared to the sapwood, 93 differential SMs were found in the heartwood, of which 16 may be related to its durability and color ([109]).
The color and smell of teak wood are affected by its secondary metabolites. The extractives contain chromatics compounds with structures that impart color to the wood, such as phenolic hydroxyl group, carbonyl group, and double bonds. It is supposed that 2-methylanthraquinone confers the special color to teak wood ([86]).
Teak bark has a complex composition that contains a considerable amount of phenolic compounds (e.g., tannins, naphthoquinones), steroids (e.g., sterols), and terpenic compounds. Baptista et al. ([5]) characterized the bark of 50-60-year-old teak trees from East Timor, quantifying the extractives content obtained from soxtec extractions using dichloromethane, ethanol, and water as solvents. The average total extractives was 10.7% of the total dry weight, of which 84% were assigned to polar extractives removed with ethanol and water, and 16% to waxes and other non-polar compounds removed with dichloromethane. They also reported an effect of particle size on the extraction efficiency, with 30% more extractives recovered from fine fractions compared to the coarse ones.
Bioactive potential of T. grandis extractives
Natural durability is defined as the ability of a timber species to prevent attacks caused by wood-destroying agents with-out any physical or chemical modifications ([100]). Teak wood is very resistant to wood-destroying fungi without additional preservation, even under high wood moisture conditions or soil contact ([108]). This resistance is attributed mainly to the quantity and type of secondary metabolites present in their extracts, such as naphthoquinones, that act as defensive compounds to environmental stresses ([41], [40], [8]). However, durability also depends on several factors, including hardness, density, location of origin, and stand management practices ([62]).
Tab. S2 (Supplementary material) summarizes the research carried out on the bioactive potential of Tectona grandis extractives, according to the solvent used for the extractions. These figures confirm that teak extracts have an attractive termicidal and fungicide potential. Some authors attributed their bioactivity not only to the toxicity of extractives but to a synergic effect of toxic and antioxidant properties ([27]).
Although teak resistance to wood-destroying organisms is often attributed to secondary metabolites, there is no consensus among researchers on the compounds responsible for its natural durability. On the one hand, some authors attribute it to compounds such as tectoquinone, lapachol, and deoxylapachol. Lukmandaru ([54]) showed that tectoquinone has a strong termicidal activity. Haupt et al. ([29]) also identified tectoquinone as a bioactive compound to inhibit fungal growth. Accordingly, Niamké et al. ([73]) found a strong correlation between resistance to decay and 2-hydroxymethyl anthraquinone and tectoquinone contents. According to Thulasidas & Bhat ([101]), 2-methyl anthraquinone and 1.4-naphthoquinone are the principal components that influence the durability and dimensional stability of teak. On the other hand, studies conducted by Windeisen et al. ([108]) suggest that tectoquinone and 2-hydroxymethylanthraquinone has no effective potential with regard to durability. These authors propose that other compounds, such as squalene, may contribute to durability by forming a hydrophobic barrier or as a precursor of toxic triterpenic compounds. Similarly, tests carried out by Haupt et al. ([29]) showed that deoxylapachol has no fungicidal effect, but it could be a precursor of tectoquinone.
Aqueous extracts from teak wood are supposed to present low amounts of compounds. In general, these extracts are not associated with natural wood resistance, as compared to those extracted with ethanol or other solvents. However, Brocco et al. ([8]) found a positive fungicide effect of teak heartwood hot-water extracts in assays with white and brown-rot fungi. Furthermore, Lanka & Parimala ([49]) reported a significant antibacterial and antifungal activity of teak bark water extracts.
In addition to affect resistance to wood decay, teak extractives also show a remarkable therapeutic and pharmacological activity ([76], [88], [107]). For examples: antibacterial ([71], [39], [46], [49]), antioxidant ([46]), analgesic ([24]), antipyretic ([98]), anti-inflammatory ([24]), hypoglycemic ([107]), diuretic ([76]), gastroprotective ([94]), hemolytic anemia ([19]), antiviral (chikungunya - [92]), antiplasmodial ([45]), and cytotoxic ([46]) effects, among others. Beyond these properties, extractives from teak leaves could have an allelopathic potential in controlling weeds and pests ([60]).
Extractives in T. grandis leaves
Tab. S3 (Supplementary material) shows the most important secondary metabolites identified in Tectona grandis leaves. Macías et al. ([59]) identified and isolated several compounds extracted from methylene chloride (DCM/H2O) and ethyl acetate (EtOAc/H2O) fractions of aqueous extracts from teak leaves (extraction at room temperature for 24 h). They found apocarotenoids and demonstrated their phytotoxicity activity; for instance, 3β-hydroxy-7.8-dihydro-β-ionol could have an allelopathic effect on lettuce and tomato. In a further study, they also isolated diterpenes and triterpenes. Furthermore, they elucidated the structure of abeograndinoic acid and demonstrated the high phytotoxic activity of 2-oxokovalenic acid and 19-hydroxyferruginol ([60]). Some studies suggest that triterpenic acids like oleanolic and ursolic or their glycosides (present in dichloromethane extracts) are involved in the resistance to termite and fungal attacks ([64], [107]).
Along with the above results, Lacret et al. ([48]) isolated lignans, norlignans, and other phenolic compounds from dichloromethane and ethyl acetate fractions of aqueous extracts from teak leaves (extraction at room temperature for 24 h - Tab. S3 in Supplementary material). They also carried out a test (etiolated wheat coleoptiles bioassay) demonstrating that these compounds could be involved in the defense mechanism of this plant. The most active compound was lariciresinol. It is also remarkable that they isolated the norlignans (tectonoelin A and tectonoelin B) for the first time from Tectona grandis. In another study, they likewise isolated anthraquinones under the same conditions ([47]).
Nayeem & Karvekar ([70]) found phenolic acids (e.g., gallic acid and ellagic acid) and flavonoids (rutin and quercetin) in methanolic extracts (soxhlet extraction) from teak. They also demonstrated in a previous study the wound healing, analgesic, and anti-inflammatory activity of the methanolic extract from teak leaves ([61]). Further, Kopa et al. ([45]) reported the presence of anthraquinones, naphthoquinones, and triterpenes in the EtOAc soluble fraction of methanol extracts (extraction at room temperature for 72 h). They also identified the quinizarine for the first time.
Since the lignocellulosic material is affected by biophysical characteristics, such as climate, soil, rainfall, tree age, and genetic factors ([101]), it is important to obtain precise information of its chemical composition to evaluate its potential. Furthermore, extractives have significant differences from tree to tree and even within an individual tree, hence the standardization and prediction of their performances represents a huge challenge ([40])
T. grandis structural composition analysis
Along with extractives and their bioactivity potential, the structural composition is relevant for teak wood characterization. Tab. S4 (Supplementary material) summarizes several studies made on the structural carbohydrates of teak wood. In addition, Tab. 2shows the monosaccharides composition of teak wood.
Tab. 2 - Tectona grandis monosaccharide composition. Sources: (1) Miranda et al. ([67]); (2) Baptista et al. ([5]).
Monosaccharide | Heartwood 1 (wt. % of wood) |
Sapwood 1 (wt. % of wood) |
Bark 2 (wt. %) |
---|---|---|---|
Glucose | 44.6 | 43.7 | 61.7 |
Xylose | 8.3 | 7.8 | 20.4 |
Mannose | 3.2 | 3.1 | 5.6 |
Arabinose | 0.6 | 0.6 | 4.2 |
Galactose | 0.6 | 0.5 | 3.1 |
Rhamnose | 0.2 | 0.5 | 5.0 |
Regarding T. grandis volatiles from the breakdown of hemicellulose, cellulose, and lignin fractions, Balogun et al. ([4]) carried out assays on teak chips from a timber processing plant in Nigeria, finding 55 compounds from the pyrolytic conversion process analyzed by gas chromatography-mass spectrometry (GC-MS); among the most abundant products, they found carbon dioxide (10.4%), acetic acid (6.4%), furan +2-butanone (5.7%), levoglucosan (5.2%), and trans-coniferyl alcohol (4.6%). Products from hemicellulose decomposition (that occurs below 265 °C) were acetic acid and 2-furaldehyde (furfural). From the decomposition of cellulose (325-357 °C) arises levoglucosan. The major phenolic compounds identified from the lignin fraction were isoeugenol (2.7%), acetoguaiacone (2.1%), 4-vinylguaiacol (2.0%), methyl guaiacol (1.6%), guaiacol (1.4%), and vanillin (1.2%); the latter results are expressed as a percentage of total chromatogram area.
Lourenço et al. ([51]) identified 127 compounds from teak pyrolysis (Py-CG-MS/ FID), reporting the following major lignin-derived compounds for heartwood: 4-vinylsyringol (6.9%), trans-coniferyl alcohol (4.4%), coniferaldehyde (2.0%), 4-vinylguaiacol (1.6%), 4-methylguaiacol (1.6%), and vanillin (1.5%). From polysaccharides, the main compounds produced were levoglucosan (14.4%), 2-hydroxymethyl-5-hydroxy-2.3-dihydro-4H-pyran-4-one (3.6%), and furfural (1.2%). Their results suggested that the high lignin content in teak wood, particularly the enrichment in the monomeric lignin unit guaiacyl, might contribute to the wood strength and high durability.
Valorization of teak residues
The use of forest and timber industry residues (wood shavings and sawdust) presents many advantages, including low cost, eco-friendly disposal, and vast availability, being among the most abundant renewable sources of biomass ([6], [23], [1]).
The ordinary use of woody wastes is as biofuel feedstock or disposal in landfills. By contrast, innovative alternatives for their valorization have recently emerged, for instance, as raw material for activated carbon production ([1], [10]); as construction materials like clay matrix bricks ([6]); as a source for rearing edible insects ([103]); in biocomposites manufacturing ([102]); and biomass-derived fuel, bio-ethanol, and bio-diesel production ([53]).
Teak sawdust has been used for high activated carbon production. It is a promising precursor due to its high carbon content (50%). Activated carbon is an efficient and non-expensive adsorbent of gaseous and aqueous phase pollutants such as pesticides and phenolic compounds ([68], [83]). Cansado et al. ([10]) studied the high porous carbon production from teak sawdust activated by carbon dioxide (physical activation) and its application in the removal of pesticides (4-chloro-2-methyl-phenoxyacetic acid) in liquid phase. They observed a removal capacity similar to that obtained through commercial adsorbents, concluding that feasible adsorption could be attained with adsorbents produced from sawdust wastes.
Mohanty et al. ([68]) investigated the carbon prepared from teak sawdust, which was chemically activated by zinc chloride and tested for phenol removal. The activated carbon showed a high surface area (about 585 m2 g-1) and an interesting adsorption capability (2.82 mg g-1 at pH 3.5). Ismadji et al. ([31]) prepared activated carbon from vacuum pyrolysis char of teak sawdust, which developed mainly microporous structures. The results obtained suggest that this material is an adequate precursor for activated carbon, with surface area and pore volume of 1150 m2 g-1 and 0.43 cm3 g-1, respectively.
Tree bark is also considered a viable adsorbent, as it contains a high amount of tannin. It is assumed that the polyhydroxyl groups of tannin are active in the adsorption process ([3]). The adsorption capability of pine bark has been studied with good results, for instance, with phenols ([104]) and polycyclic aromatic hydrocarbon removal ([50]), and as activated carbon precursor for removing organochlorine pesticides ([97]). Regarding teak bark, it has been tested as a powder for the adsorption of methylene blue in batch process, with varying initial concentration of adsorbate, agitation time and speed, pH, and particle size. Teak bark was used without treatment but washed with distilled water and sun-dried after grinding, showing an outstanding adsorption capacity of 333.3 mg g-1 ([83]).
Additionally, woody biomass wastes are a sustainable option for building material production, such as ceramic bricks. The residues could be incorporated in the bricks at the beginning of the process in replacement of clays in an amount lower than 10% w/w ([6]).
Another possibility to recycle woody residues is the preparation of composites. The aforementioned advantages and their low abrasiveness make residues suitable as fillers in wood-polymer composites (WPC - [84]). Composites are mainly manufactured with a plastic matrix and wood additives may be used. Among the most commonly used wood species for the above use are pine, maple, and oak. Patel & Rawat ([82]) evaluated WPC with different charges of teak wood flour, finding that the composite consisting of 10% of wood mixed with 15% of gum rosin had excellent impact strength, high abrasive wear resistance, and high ductility.
Taking into account that extractives play an essential role in the natural resistance of wood, they can be valorized as potential preservatives to protect other tree species with lower resistance to decay ([8]). Wood extracts have been used in commercial products for the treatment against rot, decay, and insect damage, for instance, Cedarshield® that contains natural cedarwood oil. Moreover, the allelopathic potential of teak is an opportunity to explore as a source of natural herbicidal ([48]). Nonetheless, it is crucial to take into account the limitations of using natural compounds in wood protection products, as for example, the contrasting results from laboratory studies with field trials, the narrow range of activity of some compounds, the legislation and registration of new compounds or formulations, and the risk to human health and environment due to their intrinsic biological activity ([95]).
Teak extractives can also be a promising source of bioactive compounds. Among the molecules that could be exploited in the pharmaceutical, cosmetic or food industry are squalene, lapachol, 2-methylanthraquinone, and anthraquinone-2-carboxylic acid.
Squalene (2.6.10.15.19.23-hexamethyl-2.6, 10.14.18.22-tetracosahexaene) is a triterpene that acts as intermediate in the biosynthesis of phytosterol or cholesterol in plants, animals and humans. Deep-sea shark liver oil represents the richest natural source of squalene. There are important reasons limiting the use of this source, such as the presence of persistent organic pollutants in the sea (still found in the purified squalene), the regulations against the overfishing of sharks, and the increasing trend among the consumers towards the use of plant-based products. Squalene has multiple applications due to its unique properties. It presents remarkable antioxidant and antibiotic activities derived from its chemical structure of six double bonds. The use of squalene has been cited in formulations of nutraceuticals and cosmetics products (e.g., lipsticks, makeup, and hair products). It offers a wide range of benefits such as emollient, hydrating, high spreadability, light consistency, non-greasy texture, rapid transdermal absorption, and reduction of skin damage by UV radiation. Moreover, it has been used in healing eczema and in the protection against aging and wrinkles. It is also used in the preparation of stable emulsion as an adjuvant for vaccine delivery, stimulating the immune response and increasing the patient’s response to the vaccine. Furthermore, investigations are undergoing on the potential of squalene in cancer therapy ([85]). For the abovementioned reasons, it exists a great interest in finding new natural, plant sources for squalene. The most relevant plant sources of squalene are oils extracted from amaranth, olive, palm, rice, wheat germ, grape seed, peanut, and soybean ([65]). Most vegetal oils are obtained by mechanical pressing or extraction with solvents like hexane. Recently, the biosynthesis of squalene from microbial sources has also been studied as an alternative, using yeast, bacteria, algae, and microalgae.
Concerning the squalene market, its size is projected to reach USD 184 million by 2025 from USD 129 million in 2020, at a CAGR (compound annual growth rate) of 7.3%. The growth in the cosmetic and pharmaceutical industry, along with the increasing research in the oncology sector, are the major factors driving the growth of the squalene market ([63]).
Another interesting phytoconstituent of teak wood is lapachol (4-hydroxy-3-(3-methylbut-2-enyl)naphthalene-1.2-dione); it is a natural naphthoquinone widely distributed in plants belonging to the family Bignoniaceae, such as Tabebuia avellandedae (lapachol tree, Brazilian taheebo), Catalpa ovata G.Don. Tecomella undulata (Sm.) Seem., but it has also been found in other families from the order Lamiales ([16]), such as Tectona grandis L.f. Lapachol is known for its anti-inflammatory, analgesic ([18]), antibiotic ([91]), antimalarial ([110]), anticancer ([35]), antibacterial and antifungal properties ([89]). There is a growing interest in the study of the pharmacological activity of lapachol and its derivatives in the treatments of tumors and the cytotoxic effects against different cancer types ([77], [52]). This compound displays antiproliferative activity and antimetastatic effects both in vitro and in vivo tests ([21]). Additionally, it is used as a coloring matter to dye textile fibers ([96]).
Regarding 1.4-naphtoquinone (naphthalene-1.4-dione), it is a naturally occurring compound in several families of higher plants, such as Plumbaginaceae, Juglandaceae, Boraginaceae, Dioncophyllaceae, Verbenaceae, Scrophulariaceae, Avicenniaceae, Balsaminaceae, Bignoniaceae, Gentianaceae, and Droseraceae. It is also present in algae, fungi, some animals, and in the product of metabolism of some bacteria. It shows antimalarial, antibacterial, antifungal, and anticancer activities ([14]). Besides, 1.4-naphtoquinone has been pointed out as a potential pharmacophore for inhibiting both monoamine oxidase and DNA topoisomerase activities, this latter associated with tumor activity ([17]).
With respect to one of the most studied quinones in teak extracts, namely 2-methylanthraquinone (2-methyl-9.10-anthracenedione), this compound and its derivatives are important as intermediates for manufacturing various kinds of vat dyes and brilliant blue (turquoise blue) disperse dyes ([28]).
Finally, anthraquinone-2-carboxylic acid (9.10-dihydro-9.10-dioxo-2-anthracene carboxylic acid, AQ-2-CA) is a polyphenol found in fruits, vegetables, herbs, which is widely abundant in Tabebuia avellandedae (Brazilian taheebo). It has proved anti-inflammatory and antinociceptive activity in vivo, and it has potential in the development of functional foods ([81]). In addition, some studies suggest that AQ-2-CA has a strong and long-acting anti-passive cutaneous anaphylaxis activity. Its therapeutic use could also be considered in the treatment of asthma ([15]).
The above studies indicate that the secondary metabolites found in teak wood have quite attractive properties and a real potential to be used in cosmetic or nutraceutical products, in the dyeing industry, or for medical and pharmaceutical purposes.
Conclusions
Teak timber is extensively recognized not only for its physical and aesthetic qualities, but also for its natural resistance, which is associated with the chemical composition of its extractives. This study provides a concise overview of the research work done so far in order to identify and quantify the compounds present in teak and their influence on defense against degradation agents. Teak phytoconstituents have been shown to exhibit a wide range of significant biological activities. For instance, they are active against fungi and termites, and show biocidal, allelopathic therapeutic, and antioxidant effects. In addition, teak extractives are a promising source of bioactive compounds. Further studies are still required to exploit secondary metabolites beyond research purposes and to project their use in the industry sector.
Acknowledgements
The authors are grateful to the National Council of Science and Technology of Mexico (CONACYT) for its financial support.
References
CrossRef | Gscholar
CrossRef | Gscholar
Gscholar
Gscholar
Online | Gscholar
Online | Gscholar
Gscholar
CrossRef | Gscholar
CrossRef | Gscholar
CrossRef | Gscholar
Gscholar
Gscholar
CrossRef | Gscholar
Authors’ Info
Authors’ Affiliation
Virginie Vandenbossche 0000-0002-1294-7002
Gerard Vilarem
Laboratoire de Chimie Agro-industrielle - LCA, Université de Toulouse, Institut National Polytechnique de Toulouse - INP, 6 Allée Emile Monso, BP 44362, 31030 Toulouse Cedex 4 (France)
Corresponding author
Paper Info
Citation
Chávez-Salgado LP, Vandenbossche V, Vilarem G (2022). Tectona grandis Linn. f. secondary metabolites and their bioactive potential: a review. iForest 15: 112-120. - doi: 10.3832/ifor3714-015
Academic Editor
Luigi Todaro
Paper history
Received: Dec 07, 2020
Accepted: Jan 07, 2022
First online: Mar 26, 2022
Publication Date: Apr 30, 2022
Publication Time: 2.60 months
Copyright Information
© SISEF - The Italian Society of Silviculture and Forest Ecology 2022
Open Access
This article is distributed under the terms of the Creative Commons Attribution-Non Commercial 4.0 International (https://creativecommons.org/licenses/by-nc/4.0/), which permits unrestricted use, distribution, and reproduction in any medium, provided you give appropriate credit to the original author(s) and the source, provide a link to the Creative Commons license, and indicate if changes were made.
Web Metrics
Breakdown by View Type
Article Usage
Total Article Views: 37608
(from publication date up to now)
Breakdown by View Type
HTML Page Views: 31432
Abstract Page Views: 2905
PDF Downloads: 2847
Citation/Reference Downloads: 4
XML Downloads: 420
Web Metrics
Days since publication: 1191
Overall contacts: 37608
Avg. contacts per week: 221.04
Article Citations
Article citations are based on data periodically collected from the Clarivate Web of Science web site
(last update: Mar 2025)
Total number of cites (since 2022): 9
Average cites per year: 2.25
Publication Metrics
by Dimensions ©
Articles citing this article
List of the papers citing this article based on CrossRef Cited-by.
Related Contents
iForest Similar Articles
Research Articles
Bioactivity of ethanol extracts from Eucalyptus bosistoana F. Muell. heartwood
vol. 12, pp. 467-473 (online: 14 October 2019)
Research Articles
Study on the chemical composition of teak wood extracts in different organic solvents
vol. 14, pp. 329-336 (online: 09 July 2021)
Research Articles
Density, extractives and decay resistance variabilities within branch wood from four agroforestry hardwood species
vol. 14, pp. 212-220 (online: 02 May 2021)
Research Articles
Analysis and evaluation of the impact of stand age on the occurrence and metamorphosis of red heartwood
vol. 10, pp. 605-610 (online: 15 May 2017)
Research Articles
Can bark stripping cause red heartwood formation in beech stems?
vol. 11, pp. 251-258 (online: 12 March 2018)
Research Articles
Climatic fluctuations trigger false ring occurrence and radial-growth variation in teak (Tectona grandis L.f.)
vol. 9, pp. 286-293 (online: 28 September 2015)
Research Articles
Chitosan exhibits variable effects on pine (Pinus sylvestris L.) and alder (Alnus glutinosa L.) growth and secondary metabolism
vol. 17, pp. 236-244 (online: 06 August 2024)
Research Articles
Nutrient accumulation and export in teak (Tectona grandis L.f.) plantations of Central America
vol. 8, pp. 33-44 (online: 04 June 2014)
Research Articles
Heuristic forest planning model for optimizing timber production and carbon sequestration in teak plantations
vol. 10, pp. 430-439 (online: 24 March 2017)
Research Articles
Interaction between planting spacing and wood properties of Eucalyptus clones grown in short rotation
vol. 14, pp. 12-17 (online: 02 January 2021)
iForest Database Search
Search By Author
Search By Keyword
Google Scholar Search
Citing Articles
Search By Author
Search By Keywords
PubMed Search
Search By Author
Search By Keyword