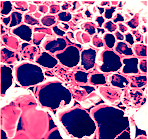
Mapping Cadmium distribution in roots of Salicaceae through scanning electron microscopy with x-ray microanalysis
iForest - Biogeosciences and Forestry, Volume 4, Issue 3, Pages 113-120 (2011)
doi: https://doi.org/10.3832/ifor0563-004
Published: Jun 01, 2011 - Copyright © 2011 SISEF
Research Articles
Abstract
A screening in hydroponics was carried out to assess the potential of Cd uptake and metal accumulation in roots of 4 poplar and 2 willow clones. Rooted cuttings were exposed for 3 weeks to 50 μM Cd sulphate in a growth chamber. Salicaceae clones were discriminated in their potential to absorb and distribute Cd in root tissues considering the interaction between metal and macronutrients. SEM-EDXMA was useful to investigate structural modifications and to identify in situ concentration and distribution of elements in roots, establishing indirect correlations between accumulation and localization of the metal. Overall, observations suggested differential patterns between species and clones in accumulating Cd within the root profile. In conclusion, Cd accumulation in root levels would hardly be a useful indicator of the ability of plants to translocate metal, independently from other parameters, while Cd location in the root cross-section levels could suggest clone-specific control mechanism to mineral uptake.
Keywords
Cadmium, EDXMA-SEM, Salicaceae clones, Phytoremediation, Roots
Introduction
Heavy metals currently and hazardously affect human, physical and biological components, inducing environmental pollution mainly caused by industrial activities. Among heavy metals, Cd is of particular concern due to its widespread occurrence and its high toxicity. Cd pollution is increasing in the environment due to mining, industrial usage and anthropogenic activity ([28], [40]). Salt et al. ([33]) observed the ability of plants to translocate significant quantities of Cd to aerial parts, which promoted the idea to use plants to clean up polluted soils, a process called phytoremediation.
Poplars and willows have the potential to provide a cheap method of cleaning up Cd-contaminated soils because of their high biomass production and high Cd bioaccumulation coefficient (e.g., [11], [32]). Plant biomass, metal tolerance, and metal accumulation in roots and leaves vary greatly between clones ([12]). Phytoextraction potential of a clone is primarily determined by metal concentration and by biomass production ([24]). The variability of Cd distribution among leaves, stem, and roots provides candidate Salicaceae for specific phytoremediation processes of contaminated waters and soils ([30]). Considering the impact of Cd on Salicaceae rooting, Lunácková et al. ([25]) observed seriously damaged root parts, apex, rhizodermis and cortex, while the structure of central cylinder remained unchanged following treatment, showing resistance of root apical meristem and accumulation of darkly stained material around the central cylinder.
Cadmium comes into plants through the root system. Several transporters have been identified as playing a potential role in the uptake, including the ZIP family (ZRT1/ IRT1-like proteins - [9]), Ca channels ([29]) or members of the Nramp family ([26]), which are normally responsible for the uptake of Zn2+, Fe2+, Ca2+ or Mn2+. Once transported across the plasma membrane inside the roots, heavy metal ions may be translocated, in some cases, to the aerial part of the plant via the xylem. Besides, compared to other metals (i.e., As), Cd has high trend to accumulate also in other organs than the roots, like stems and leaves ([6], [39]). However, roots are interested by stress adjustments earlier than leaves ([20]). Stress exposure in roots occurs immediately upon the beginning of the treatment, when the Cd content in roots is very high ([21]). Cadmium ions cross plasmalemma and internal membranes through transporters /channels with a low specificity ([6]). However, the mechanism, accounting for the transport of heavy metals across membranes in plants and its regulation, is far from understood.
Scanning electron microscopy allows for the observation of samples, at high magnifications and good field depth with a minimum preparation, and with the possibility to combine structural and analytical information by energy-dispersive x-ray microanalysis and digital images (SEM-EDXMA). Although SEM-EDXMA has been used with some success to plot the subcellular distribution of metals (e.g., [16], [17]), a poor sensitivity and interference with other cations (e.g., K) may limit Cd detection. Nevertheless, metal distribution within plant organs and tissues may be a good indicator of detoxification and tolerance mechanisms. In this sense, hydroponic screenings show several advantages ([12]) in selecting from a considerable number of individuals while reducing the variability due to the environmental factors ([31]).
The knowledge of Cd effects on the absorption, accumulation and distribution of essential elements, such as Ca, Fe and P, in roots is still marginal and remains to be investigated. In the case of the addition of Ca in a culture medium polluted by Cd, an increase of biomass production in tomato plants was observed by Boulila Zoghlami et al. ([4]). Cocozza et al. ([7]) showed increasing Fe and decreasing P concentrations in root tissues of poplar clones subjected to excess Cd. Cadmium may inhibit Fe translocation from roots to shoots, inducing the inhibition of enzyme Fe(III)-chelate reductase ([1]), and consequently Fe accumulation in roots ([15]).
In a companion study, Zacchini et al. ([39]) defined Cd tolerance, accumulation and translocation ability, in poplar and willow clones treated with Cd in hydroponic systems. The present study aimed at identifying the distribution of Cd and essential elements in root tissues of six candidate clones of Salicaceae, with screening purposes. We focused on the investigation of the Cd allocation strategies, observing the metal distribution at the root level and root cross-section level, and describing symptoms of Cd toxicity or interference, considering the interaction with others chemical elements. The specific objectives of the study were to: (i) validate whether short-term Cd treatment induced differential Cd absorption in the selected clones; (ii) investigate the Cd allocation in root levels (apex, central and distal zone) and across cross-sections (central cylinder, cortex and epidermis); (iii) to establish a link between the distribution of Cd and that of essential elements in roots.
Materials and methods
Plant material and growth conditions
Woody cuttings of Salicaceae clones (A4A and I-214 Populus x canadensis Mönch.; Poli and 58-861 Populus nigra L.; SS5 Salix alba and Cretone Salix sp.) with uniform size (20 cm in length and diameter larger than 1 cm) were collected from mother plants at IBAF-CNR (Montelibretti - Roma, Italy). Cuttings were grown in pots suitable for hydroponic culture (diameter 13-15 mm) with 3 L of third-strength Hoagland’s nutrient solution, pH 6.5 ([2]). The growth conditions were highly controlled with metal halide lamps (Powerstar HQI-TS - Osram, Munich, Germany) providing a photon flux density of 300 μmol m-2 s-1 for 14 h at 25 °C. During the 10 h dark period the temperature was 20 °C. The relative humidity was 60-70%. Experimental details can be found in Zacchini et al. ([39]) and Pietrini et al. ([30]).
After 3 weeks of development, five homogeneous plants per clone were selected and randomly assigned to two groups of treatment: control (0 μM) and Cd-treatment (50 μM CdSO4 - Sigma, St. Louis, USA) for three weeks. Each treatment group consisted of five cuttings from each clone. The nutrient solutions were replaced entirely twice a week to prevent depletion of metals and nutrients and to expose plants to a constant metal concentration. An aeration system based on pumps was used to avoid lack of oxygen.
Root sampling
The length of the main root was measured using caliper after three weeks of Cd-treatment, when plants were gently removed from hydroponics.
Three segments of primary roots were taken from each plant and immediately plunged in a solution of 3% glutaraldehyde (0.01 mol l-1 phosphate buffer) for microscopic analysis. These segments were approximately 0.5 cm long: the root apex, the central zone corresponded to the middle of primary root, and the distal zone at the end of primary root.
Energy-dispersive x-ray microanalysis (EDXMA) and scanning electron microscope (SEM)
Chemical elements were determined in roots of control and treated plants using scanning electron microscopes (SEM) equipped with energy-dispersive x-ray detector (EDX), which produces point-specific measurements. Root samples were fixed in 3% glutaraldehyde solution (C5H8O2, 0.01 mol l-1phosphate buffer) to maintain protein structures, and then post-fixed for 3h in 1% osmium tetroxide (OsO4) to fix proteins and lipids and to improve the contrast of electron microscope pictures. Specimens were subsequently rinsed in fresh buffer and dehydrated through a series of ascending ethanol-water solutions (20-30-40-60-80-95-100%), with a last wash in acetone for a better CO2 substitution during the dehydration procedure at a pressure of 1200 bars. Afterward, root samples were dried to critical-point (critical point drier K850 - Emitech, Ashford, UK) to produce an electrically conductive surface and thus prevent charging under the electron beam, and then covered with carbon (EDXMA) and gold (SEM) through sputtering (sputter coater K550 - Emitech, Ashford, UK) under vacuum. Carbon was used instead of metal coating to avoid interference with measured elements, while gold was used to improve conductivity and increase topographic contrast. Observations were done in a SEM (Zeiss DSM 940A, Oberkochen, Germany) operating at 10 kV.
Micrographs of roots
Micrographs were developed for different root levels: the apex, the central and the distal zones. For each root portion, EDXMA was conducted per specific dot considering positions across sections: the central cylinder, the cortex and the epidermis (Fig. 1). Mapping of elements in cross-sections was also produced through EDXMA, as shown in detail in Fig. 2.
Statistical analysis
The experiment was set up in a completely randomized design with five replicate plants for each treatment (n = 5). Data were averaged on a plant basis and subjected to analysis of variance (ANOVA) to test significant differences between the main effect of genotype, treatment and interaction terms. The Student’s t-test (pairwise) was used to compare Cd concentration means for these clones. Significant effects were considered at P ≤ 0.05.
Results
Root length
Cretone, I-214, A4A and Poli showed a decrease in root length upon Cd exposure comparing to control plants (Fig. 3), and a significant interaction clone x treatment was found (Fig. 3). Instead, 58-861 and SS5 grew under Cd treatment with no significant reduction in root length.
Fig. 3 - Root length measured at the end of Cd exposure, in treated and control plants, for each clone. Data are means ± SE (n = 5). Asterisks on the histograms indicate significant differences between treatments for each clone (ANOVA test). The analysis of variance for clone and treatment effects is reported in the box. (*): P < 0.05; (**): P < 0.01; (***): P < 0.001; (NS): not significant.
Cadmium and macronutrient distribution in roots
Cadmium and macronutrient contents in roots of selected clones were calculated as percentage amount on a dry weight basis of analyzed sections and are reported in Tab. 1, Fig. 4 and Fig. 5. Macronutrients were not detected in all analyzed points, because of detection limits. Cadmium content in control plants was generally below or near the detection limit and is not shown in Tab. 1.
Tab. 1 - Cadmium contents determined by SEM-EDXMA in control and treated roots of poplar and willow clones at root levels and cross-sections (in % weight, mean ± SE). One-way ANOVA was performed for Cd in root levels and cross-sections. (*): P < 0.05; (**): P < 0.01; (***): P < 0.001; (NS): not significant.
Level | Zone | Cretone | SS5 | I-214 | 58-861 | A4A | Poli |
---|---|---|---|---|---|---|---|
Root | apex | 0.425 (0.2) | 0.943 (0.6) | 3.435 (0.2) | 1.518 (0.7) | 2.878 (0.6) | 1.458 (0.1) |
central zone | 2.262 (1.9) | 1.480 (0.1) | 2.789 (1.3) | 4.623 (1.3) | 1.215 (0.3) | 0.757 (0.3) | |
distal zone | 1.666 (0.1) | 2.519 (0.6) | 2.501 (0.4) | 2.833 (0.8) | 1.708 (0.5) | 0.796 (0.4= | |
p-level | NS | *** | * | *** | *** | ** | |
Root cross-section | central cylinder | 0.623 (0.5) | 1.673 (0.5) | 2.475 (0.6) | 1.339 (0.5) | 1.644 (0.5) | 0.725 (0.3) |
cortex | 1.160 (0.4) | 1.703 (0.2) | 2.728 (0.6) | 4.289 (2.2) | 2.417 (1.0) | 1.166 (0.4) | |
epidermis | 2.570 (1.8) | 1.809 (1.1) | 3.522 (1.1) | 3.545 (0.8) | 1.740 (0.5) | 1.120 (0.3) | |
p-level | * | NS | *** | * | *** | * |
Fig. 4 - Fe, Ca and P determined by SEM-EDXMA in root levels (apex, central and distal zone), in control and treated roots of poplar and willow clones. Data are expressed in % weight mean ± SE.
Fig. 5 - Fe, Ca and P determined by SEM-EDXMA in root cross-sections (central cylinder, cortex and epidermis), in control and treated roots of poplar and willow clones. Data are expressed in % weight mean ± SE.
Cadmium contents were significantly different between root levels in each clone, except for Cretone, and overall higher in 58-861, I-214 and A4A (Tab. 1). Clone 58-861 showed relatively high Cd content in the central zone, A4A and Poli in the apex, and SS5 in the distal zone (Tab. 1). Furthermore, Cd distribution was significantly variable between cross-sections, except for SS5 (Tab. 1). Relatively high Cd contents were measured in the cortex of 58-861, A4A and Poli, in the epidermis of I-214 and Cretone (Tab. 1).
Total contents of Cd, Ca and P in the root system were significantly different between clones (Tab. 2). Interactions “clone” x “root level” for Ca and “clone” x “root cross-section” for P were not significant (Tab. 2).
Tab. 2 - Two-way ANOVA was performed for Cd, Fe, Ca and P (root levels: apex, central and distal zone; cross-sections: central cylinder, cortex and epidermis). (*): P < 0.05; (**): P < 0.01; (***): P < 0.001; (NS): not significant.
Elements | Clone(C) | Root Level (RL) |
Root Cross-Section (RCS) |
C x RL | C x RCS |
---|---|---|---|---|---|
Cd | ** | NS | * | * | NS |
Fe | NS | NS | ** | * | ** |
Ca | * | ** | *** | NS | * |
P | *** | *** | NS | *** | NS |
In 58-861 (Fig. 4), Fe contents in central and distal zones were higher for treated roots (P < 0.05). In Poli, Fe contents were higher in root apexes and lower in the distal zone of treated roots (P < 0.001). In Cretone, Fe contents were higher in the central zone of treated roots (P < 0.05), while in the distal zone Fe was below the detection limit. In Poli, Ca showed lower contents in the central and the distal zone of treated roots (P < 0.05). In A4A, P contents were higher in the central zone of treated roots (P < 0.001). In Poli, P contents were lower in root levels of treated plants (P < 0.05). In Cretone, P was detected only in the distal zone of treated roots (P < 0.05).
In 58-861 (Fig. 5), significant differences in Fe and Ca contents were found through cross sections, being higher in epidermis and cortex of treated roots (P < 0.01). In I-214, Ca varied significantly between cross sections (P < 0.05), resulting lower in treated roots. Instead, P contents did not vary through cross sections of roots.
Relationships between elements were analyzed, being significant for each clone only in the case of Cd-Ca contents (Fig. 6). The relationships showed higher slopes in willow than poplar clones, with willows showing higher Cd contents at similar Ca contents than poplars. The relationships were highly variable between poplars, evidencing two opposite cases, 58-861 with high Cd and Ca contents, and Poli, characterized by low contents.
Discussion
The present paper was part of a project aimed at screening poplar and willow clones with tolerance to excess Cd and uptake capacity for remediation of heavy metal polluted soil. The selection of high performing clones was based on metal accumulation. Overall, all the selected clones analyzed in the present experiment showed a considerable potential for Cd uptake in roots. This ability is in agreement with previous results obtained in companion studies ([39], [30]).
Root length was unaffected by excess Cd in 58-861 and SS5. Root length defines the capability to absorb water and nutrients, and consequently metal uptake is more strongly related to root length than root weight ([3], [37]). Root elongation could offer a simple screening parameter for highlighting different sensitivity to Cd in clones of poplar and willow ([39]). A fine, widely branched root system was considered a good selection criterion for plant species in phytoremediation ([27]).
Microanalysis allowed describing some Cd allocation mechanisms in roots of these Salicaceae, which may support different strategies for tolerating metal, depending on exclusion and detoxification mechanisms. The short-term metal exposure was sufficient for Cd detection and illustrated a clone-specific distribution of elements in roots tissues. Total Cd content showed higher ability of poplar clones to remove metal from the solution than willow clones (see also [39]), which could imply a substantial capability for more advantageous translocation of metal to the aboveground plant structures. The integrity of root system involved in Cd uptake, coupled with the capacity of translocation to shoots, represents an important factor in screening clones for phytoremediation ([23], [34], [22]).
A remarkable Cd accumulation was identified in root levels, showing clone-specific Cd thresholds. A great Cd accumulation in roots was found in 58-861 and I-214, while this was not the case in Poli, Cretone and SS5. At first glance, 58-861 with unaltered root length under excess Cd could represent a good candidate for phytoremediation. On the opposite side, SS5 showed high Cd content in the distal zone of its roots, which may suggest a low transport efficiency of Cd, but also an efficient transport mechanism along root and a rapid metal translocation to leaves. Again, higher Cd contents in root apex than distal zone were observed in A4A and Poli, suggesting a slow metal translocation. Whereas, an intermediate solution for metal transportation could be identified in 58-861, showing high Cd content in the central zone. Furthermore, Pietrini et al. ([30]) observed a decreased transpiration in Cd-treated plants that implies an effect of Cd on plant water relations. Hence, results on Cd allocation at root level could not be conveniently used in understanding the efficiency or the rapidity of metal transportation through the system, if uncoupled to a comprehensive ecophysiological analysis.
I-214 and Cretone were characterized by high Cd contents in the epidermis, which might suggest slow or reduced metal mobility towards the central cylinder. Whereas, 58-861, A4A and Poli had high Cd contents in the cortex. Cd was not detected in high percentage in internal tissues, central cylinder, being important for identifying any anatomical barrier to metal movement, as obstruction to the distribution of elements from the epidermis to the central cylinder. This, in general, could give control over mineral uptake to the plant, but can also represent a tolerance strategy in phytoremediation systems. On the other hand, differential Cd localization in cross sections could suggest clone-specific metal mobility through internal root tissues. Other than exclusion strategy, internal detoxification is also an important strategy in metal tolerance, and these clones may have evolved different strategies for metal tolerance (e.g., [5]).
A partial root barrier may facilitate while a tight barrier may obstruct the uptake of water, ions and nutrients at the basal root zones (e.g., [35], [10]), therefore, these mechanisms could be likewise adopted by plants to control the metal and toxic elements distribution in roots. In this contest, the Casparian strip could be an obligatory passage to filter the aqueous absorbed solution, from the cortex to the central cylinder in roots ([18]), thus limiting Cd distribution crossways the root. In Fig. 7, sequential details show the significance of these anatomical structures in providing direction for studies on the control of metal diffusion in root tissues. Accordingly, the exclusion ability conferred by root barriers is presumably an important mechanism for Cd translocation to leaves, which might define clone-specific aptitude to preserve aboveground structures by toxic elements and to absorb metals for environmental cleaning. Micrographs did not show damages and necrosis induced by excess Cd in root tissues of any of the tested clones.
Fig. 7 - Details of ambient temperature scanning electron micrograph for 58-861 root cross-sections, proceeding from 500 to 40 µm of magnification.
An interesting relationship was found between metal and Ca contents, suggesting that the accumulation of Cd did not interfere with macronutrient absorption, while these clones cope with metal stress. There is no specific transporter for selective Cd uptake, but the metal could also permeate through Ca channels, being both divalent cations ([19], [29]). The distribution of Ca in roots was not affected by Cd, except in Poli (root levels), in 58-861 (root cross-section), and in I-214 that showed lower Cd contents in treated than control roots. Results on Ca distribution in roots might certificate efficient transport Ca-channels under excess Cd, and/or metal concentration below critical threshold. Commonly, high concentrations of heavy metals may block plant water channels and also affect ion channels and transport of osmotically active elements, which provide the driving force for water transport ([14]).
Both high contents of Cd and Fe were found in root portions, such as in central and distal zones for 58-861, and in apex for Poli. Fodor et al. ([15]) showed that Cd contents increased in root and shoot with Fe-chelates, while, in contrast, Cohen et al. ([8]) observed that Fe deficiency induced the expression of transporter, which might facilitate the transport of heavy-metal divalent cations, such as Cd2+ and Zn2+, in addition to Fe2+. Possible mechanisms of enhanced Cd uptake could be explained by high affinity of nutrient transporter for metal uptake. For instance, Cohen et al. ([8]) and Vert et al. ([36]) observed the induction of a Fe transporter that could account for enhanced uptake of divalent cations, including Cd and Fe respectively, in Fe-deficient plants.
In addition, P was not differently distributed in root cross-sections, but it was high when Cd content was low, such as in central zone for A4A and Poli. Williams & David ([38]) observed that Cd uptake was also affected by the distribution of phosphate in the root zone, because the addition of phosphate led to the proliferation of roots in the soil phosphate-treated layers. As a result, experiments in hydroponics do not represent the best environment to define the role of P in Cd uptake ([13]).
Overall, Cd tolerance could be defined by metal distribution and by lack of interference with the distribution of macronutrients into root tissues. Again, dynamics of Cd distribution in roots could be also responsible for the clone-specific ability to cope with high Cd concentrations and for their overall high tolerance to Cd. Finally, the large root concentrations observed in this experiment, although probably partly due to high Cd availability in hydroponics, might indicate that metal removal by roots at the end of the remediation process would allow extraction of an additional amount of Cd.
The accumulation and distribution of Cd in roots were important traits for the evaluation and screening of these clones under excess Cd. Clone 58-861 could maintain large and widely resistant root system absorbing high metal content, and thus has great potential applications in large-scale phytoremediation projects. As opposite, clone Poli might either have high metal translocation to leaves (see also [39]), or low potential to absorb high Cd contents. Clone SS5 was not affected by Cd excess at the level of root length, though showing metal content comparable to other clones.
We conclude that Cd accumulation in root levels cannot be unquestionably used as indicator of the ability of Salicaceae to translocate metal, while Cd localization in root cross-sections would suggest clone-specific control mechanisms to mineral uptake. However, information obtained by SEM-EDXMA could contribute to programs aimed at elucidating functional and structural aspects of plant-metal interaction.
Acknowledgements
We acknowledge the Project “Molecular, physiological, and agronomic analyzes for selecting and managing Salicacee in phytoremediation” (MIUR, PRIN 2008) for financial support and IBAF-CNR for experimental maintenance.
References
Gscholar
CrossRef | Gscholar
Gscholar
Gscholar
CrossRef | Gscholar
Authors’ Info
Authors’ Affiliation
R Tognetti
Dipartimento di Scienze e Tecnologie per l’Ambiente e il Territorio (STAT), Università del Molise, c.da Fonte Lappone, I-86090 Pesche (Italy)
Dipartimento di Scienze e Tecnologie Agro-Alimentari Ambientali e Microbiologiche, Università del Molise, v. De Sanctis, I-86100 Campobasso (Italy)
Corresponding author
Paper Info
Citation
Cocozza C, Maiuro L, Tognetti R (2011). Mapping Cadmium distribution in roots of Salicaceae through scanning electron microscopy with x-ray microanalysis. iForest 4: 113-120. - doi: 10.3832/ifor0563-004
Paper history
Received: Oct 05, 2010
Accepted: Mar 01, 2011
First online: Jun 01, 2011
Publication Date: Jun 01, 2011
Publication Time: 3.07 months
Copyright Information
© SISEF - The Italian Society of Silviculture and Forest Ecology 2011
Open Access
This article is distributed under the terms of the Creative Commons Attribution-Non Commercial 4.0 International (https://creativecommons.org/licenses/by-nc/4.0/), which permits unrestricted use, distribution, and reproduction in any medium, provided you give appropriate credit to the original author(s) and the source, provide a link to the Creative Commons license, and indicate if changes were made.
Web Metrics
Breakdown by View Type
Article Usage
Total Article Views: 58557
(from publication date up to now)
Breakdown by View Type
HTML Page Views: 49830
Abstract Page Views: 3067
PDF Downloads: 4249
Citation/Reference Downloads: 32
XML Downloads: 1379
Web Metrics
Days since publication: 5155
Overall contacts: 58557
Avg. contacts per week: 79.51
Article Citations
Article citations are based on data periodically collected from the Clarivate Web of Science web site
(last update: Mar 2025)
Total number of cites (since 2011): 18
Average cites per year: 1.20
Publication Metrics
by Dimensions ©
Articles citing this article
List of the papers citing this article based on CrossRef Cited-by.
Related Contents
iForest Similar Articles
Research Articles
Analysis of biometric, physiological, and biochemical traits to evaluate the cadmium phytoremediation ability of eucalypt plants under hydroponics
vol. 10, pp. 416-421 (online: 24 March 2017)
Research Articles
Distribution and concentration of cadmium in root tissue of Populus alba determined by scanning electron microscopy and energy-dispersive x-ray microanalysis
vol. 1, pp. 96-103 (online: 20 May 2008)
Research Articles
Can species Cedrela fissilis Vell. be used in sites contaminated with toxic aluminum and cadmium metals?
vol. 14, pp. 508-516 (online: 11 November 2021)
Research Articles
Effect of cadmium (Cd) and lead (Pb) soil contamination on the development of Hymenoscyphus fraxineus on Fraxinus excelsior and F. angustifolia seedlings
vol. 16, pp. 307-313 (online: 09 November 2023)
Research Articles
Assessment of cadmium tolerance and phytoextraction ability in young Populus deltoides L. and Populus × euramericana plants through morpho-anatomical and physiological responses to growth in cadmium enriched soil
vol. 10, pp. 635-644 (online: 01 June 2017)
Review Papers
A review of the performance of woody and herbaceous ornamental plants for phytoremediation in urban areas
vol. 13, pp. 139-151 (online: 14 April 2020)
Review Papers
Monitoring the effects of air pollution on forest condition in Europe: is crown defoliation an adequate indicator?
vol. 3, pp. 86-88 (online: 15 July 2010)
Research Articles
Heavy metal accumulation characteristics of Nepalese alder (Alnus nepalensis) growing in a lead-zinc spoil heap, Yunnan, south-western China
vol. 7, pp. 204-208 (online: 27 February 2014)
Review Papers
Heavy metals and woody plants - biotechnologies for phytoremediation
vol. 4, pp. 7-15 (online: 27 January 2011)
Research Articles
Revealing the physiological basis of forester’s choice of poplar clones (Populus spp.)
vol. 17, pp. 156-164 (online: 14 June 2024)
iForest Database Search
Search By Author
Search By Keyword
Google Scholar Search
Citing Articles
Search By Author
Search By Keywords
PubMed Search
Search By Author
Search By Keyword