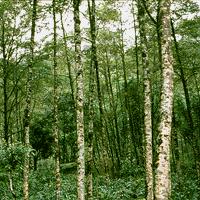
Heavy metal accumulation characteristics of Nepalese alder (Alnus nepalensis) growing in a lead-zinc spoil heap, Yunnan, south-western China
iForest - Biogeosciences and Forestry, Volume 7, Issue 4, Pages 204-208 (2014)
doi: https://doi.org/10.3832/ifor1082-007
Published: Feb 27, 2014 - Copyright © 2014 SISEF
Research Articles
Abstract
A fast-growing alder species native to the eastern Himalayas, Nepalese alder (Alnus nepalensis), has recently received considerable attention in the restoration of contaminated lands due to its significant economic benefits and ecological functions. The bioaccumulation characteristics and phytoremediation potential of naturally regenerated Nepalese alder were evaluated in a lead-zinc spoil heap located in Lancang county, Yunnan province, south-western China. Results showed that bioaccumulation factors (BFs) of A. nepalensis for Zn and Pb were always >1 in slightly contaminated soils (extractable Zn, Pb of 4.2-17.9 and 3.4-13.1 mg kg-1, respectively) and >1 for Cd in contaminated soils (extractable Cd 0.3- 6.8 mg kg-1). By contrast, translocation factors (TFs) for Zn were <1 in all sampling plots, but >1 for Pb in soil slightly contaminated by 13.1 mg kg-1 extractable Pb and >1 for Cd in contaminated soils (extractable Cd 2.6- 6.8 mg kg-1). Significant positive correlations were found between heavy metals (HMs) in roots and extractable HMs in soils (p<0.01) and between HMs in shoots and extractable HMs in soils (p<0.05) except for Cd. Based on the accumulation capacity revealed in this study, we suggest that A.nepalensis is a promising tree species for phytostabilization of zinc and lead in soils slightly contaminated with Zn and Pb and for phytoextraction of cadmium in Cd-polluted soil.
Keywords
Phytoremediation, Nepalese Alder, Alnus nepalensis, Metal Contamination, Bioaccumulation Factor, Translocation Factor
Introduction
Contamination of soil with zinc, lead and cadmium due to residues from metalliferous mining, smelting and other anthropic activities has attracted considerable attention in the past decade ([27]). Because of the high toxicity of heavy metals (HMs) and their undegradable nature, metal pollution of soils has become one of the most serious environmental problems ([10]). In addition, heavy metals can be transferred to the food chain from the soil and seriously affect human health ([1]). First investigated by Chaney in 1983 ([1]), phytoremediation is a pro- mising, ecologically-friendly approach to the remediation of contaminated soil using plants to remove toxic elements from the environment or render them non-toxic ([16], [21]). However, most plants are sensitive to excessive HMs in soil. In nature, only a small fraction of plants that exhibit metal tolerance or super-accumulative capacity (hyperaccumulators) can survive in toxic metal-contaminated soils, and the majority are herbaceous species with small biomasses ([17]). Woody plants or trees with developed root systems and large biomasses are especially attractive for revegetation and phytoremediation in metal-polluted sites ([10], [4]). Additionally, exploring native plants with phytoremediation potential is a particularly important strategy, as indigenous plants are often more dependant in terms of survival, growth and reproduction under environmental stress than exotic plants ([26]).
Native to Pakistan, eastern Nepal, Bhutan, northern India, south-western China, upper Myanmar and parts of Indochina, A.nepalensis is a multipurpose alder species. Characterized by a series of merits such as fast growth and nitrogen fixation, its uses include commercial timber production of moderately soft wood and charcoal feedstock. Moreover, A. nepalensis is a chief associate in various commercial timber plantations, where it not only helps to raise the nitrogen levels of fields but also acts as a pioneer species on denuded habitats with freshly exposed soils, or rocky and eroded slopes in subtropical-to-temperate belts of the Himalaya ([19]). Several other Alnus species have been surveyed to evaluate their phytoremediation potential for metal contaminated soils. For example, Rosselli et al. ([15]) found that Alnus incana could be considered as an excluder of Zn, Cd and Cu. Mertens et al. ([12]) found that Alnus glutinosa showed low foliar heavy metal concentrations and might therefore be suitable for phytostabilization purposes on polluted sites. Lee et al. ([10]) also reported that Alnus hirsuta was a better candidate than Alnus firma for phytoremediation of HM-contaminated soils. However, there is no information about the potential of Alnus nepalensis to remediate Zn-, Pb- and Cd-contaminated soils.
In the present study, the metal accumulation capacity of A. nepalensis growing in a lead and zinc mining spoil heap in Lancang county of Yunnan province (south-western China) was evaluated. The potential application of Alnus nepalensis for phytoremediation of Zn-, Pb- and Cd-contaminated soils is also discussed.
Materials and methods
Site description
The sample site is approximately 3 x 5 km and is located in the tailing of Munai mine in Lancang county, Yunnan province, southwestern China, where zinc and lead mining has been carried out using traditional Chinese methods for approximately 600 years ([5]). An A. nepalensis population has developed and is dominant in the lead-zinc spoil heap. Several shrub, grass and forb species, such as Bidens pilosa, Cynodon dactylon, Ageratina adenophora, Arthraxon hispidus, Pseudocyclosorus esquirolii and Sphenomeris chinensis, are growing in addition to A.nepalensis. Five sampling plots (P1-P5) were selected within the study area. The distances from sampled plots to the lead-zinc smelting facility were 200 m, 500 m, 1700 m, 2800 m and 3800 m, and these distances constituted the gradient of HM concentrations in soils involved in our study.
Sample collection and analysis
At each of the sampling plots, 6 to 10 naturally regenerated A. nepalensis seedlings (approximately 2-3 years old) were randomly chosen. Their roots, shoots (stems + leaves) and rhizosphere soils (depth 10-25 cm, 500 g) were collected in May 2010.
From each sampling plot, 6 plant samples were selected for analysis. The shoots (stems + leaves) and roots of the whole A. nepalensis seedlings were washed thoroughly under tap water (roots were scrubbed with a brush carefully) and then placed in an 80 °C oven to be dried to constant weight. All dried root and shoot samples were ground separately by a mini-vegetation grinder (FZ102, Tianjing City Test Instrument Co. Ltd., China), and 0.5 g shoot and 0.5 g root powder of each sample were then subsampled by the usual method of coning and quartering. These representative subsamples were digested by HNO3 + HClO4, and the HM ion concentrations were determined by flame atomic absorption spectrometry (FAAS) using a Z2000 polarized Zeeman atomic absorption spectrophotometer (Hitachi, Japan). Quantification was carried out with a calibration curve using a graded series of diluted lead (GSW08619: 0, 0.1, 0.2, 0.4, 0.8, 1.2 μg ml-1), zinc (GSW08620: 0, 0.1, 0.2, 0.4, 0.8, 1.2 μg ml-1) and cadmium (GSW08612: 0, 0.1, 0.2, 0.4, 0.8, 1.2 μg ml-1 - National Research Center for Certified Reference Materials, China). A standard reference material of poplar leaves (GBW07604 - National Research Center for Certified Reference Materials, China) was carried through the digestion and analyzed as part of the quality control protocol (accuracies within 100 ± 20 %). A hollow cathode lamp was operated at 4 mA for Cd and 5 mA for Pb and Zn, and the analytical wavelengths were set at 217.0, 213.9 and 228.8 nm for detection of Pb (II), Zn (II) and Cd (II), respectively ([2]).
Rhizosphere soil samples corresponding to the above plants analyzed were also chosen for measuring the concentrations of Zn, Pb and Cd (6 samples for each plot, a total of 30 samples were analyzed). Soil samples were air-dried, ground to fine powder in a mortar, passed through a 2 mm sieve, and divided into two portions by quartering. One portion was directly used for the determination of extractable soil Zn, Pb and Cd, and 5.0 g soil was weighed and put into a 100 ml Erlenmeyer flask. Then, 50 ml of 0.1 M HCl was added, and the solution was shaken for 2 h at 20 °C and filtered into a volumetric flask. Concentrations of Zn, Pb and Cd were determined by FAAS as described above. The other portion was further put through a 0.15 mm sieve for the analysis of soil total HMs. For each soil sample, 0.2 g soil was digested in 5 ml of aqua regia (ultrapure mixture of concentrated HNO3/HCl, 1/3 v/v) in a teflon crucible and diluted with 50 ml distilled water, and then their total HM content was analyzed by FAAS ([18]).
Translocation factors (TF = Cshoot/Croot, i.e., the ratio of HM concentration in shoot to root) were calculated to quantify the translocation of HMs from root to shoot, and bioaccumulation factors (BF = Cshoot/Csoil, i.e., the ratio of HM concentration in shoot to the extractable HMs in the soil) were calculated to quantify the HM accumulation ability of A. nepalensis from soil to shoot ([11]).
Statistical Analysis
Departure from the normal distribution was tested for all variables by Shapiro-Wilk’s test, and Levene’s test was used to verify the equality of variances. HM ion concentration data were subjected to one-way ANOVA to test for differences among the five sampling plots. The threshold for statistical significance was set at α=0.05. The relationships among extractable soil metals and total soil metals, plant metal concentrations, BF and TF were determined by Pearson’s correlation analysis. Statistical analysis was carried out using the SPSS software package (version 17.0).
Results
HM content in soil
Concentrations of HMs in the soils investigated are summarized in Tab. 1. Three plots, P1, P2 and P3, were seriously polluted by Zn, Pb and Cd, and 2 plots, P4 and P5, were slightly contaminated, except for Cd. Extractable HMs in the proportion of the total soil HMs ranged from 2.0 to 22.5 % for Zn, 8.5 to 17.2 % for Pb and 4.8 to 39.3 % for Cd. In addition, a significant, positive correlation between soil total and extractable HMs was observed (Tab. 2).
Tab. 1 - Heavy metal contents in the soil (mg kg-1, mean ± SE, n=6) from the tailing of Munai mine in Lancang county of Yunnan province, south-western China. P1 to P5 represent five sampling plots with different distances (200 m, 500 m, 1700 m, 2800 m and 3800 m) to the lead-zinc smelting facility. Different letters in the same column indicate significant differences at p<0.05 level, according to the LSD test.
Plot | Zn | Pb | Cd | |||
---|---|---|---|---|---|---|
Total | Extractable | Total | Extractable | Total | Extractable | |
P1 | 6285.3 ± 1172.8 a | 1417.1 ± 479.3 a | 36845.5 ± 10895.1 a | 12148.0 ± 1707.0 a | 81.8 ± 43.0 a | 32.4 ± 19.0 a |
P2 | 4147.7 ± 1108.3 b | 900.7 ± 105.6 b | 32323.5 ± 17071.1 a | 5544.4 ± 1032.5 b | 35.9 ± 17.4 b | 13.8 ± 4.3 b |
P3 | 6351.4 ± 1565.7 a | 392.9 ± 80.9 c | 15189.7 ± 20029.1 b | 2699.9 ± 838.1 c | 25.7 ± 14.9 bc | 6.8 ± 1.3 bc |
P4 | 415.8 ± 206.4 c | 17.9 ± 2.6 d | 186.1 ± 13.9 c | 13.1 ± 1.5 d | 12.0 ± 6.3 c | 2.6 ± 1.2 c |
P5 | 212.2 ± 56.1 d | 4.2 ± 2.8 d | 40.5 ± 36.3 c | 3.4 ± 2.0 d | 5.9 ± 3.1 d | 0.3 ± 0.2 d |
Tab. 2 - Pearson’s correlation coefficients between extractable soil metals and total soil metals, plant metal concentrations, bioaccumulation factors (BFs) and translocation factors (TFs). (*): p<0.05; (**): p<0.01.
Metal | Total metal |
Shoot | Root | BF | TF |
---|---|---|---|---|---|
Extractable Zn | 0.764* | 0.940* | 0.940** | -0.58 | -0.577 |
Extractable Pb | 0.920** | 0.850* | 0.990** | -0.723* | -0.531 |
Extractable Cd | 0.997** | -0.364 | 0.983** | -0.772* | -0.685* |
Accumulation and distribution of HMs in A. nepalensis
The distribution of the metals within A. nepalensis is presented in Fig. 1. In general, HMs were accumulated mainly in roots, and concentrations of HMs in the roots decreased as extractable HMs in the soil decreased (Fig. 1 and Tab. 1). The highest concentration of HMs in roots occurred in sampling plot P1, where the concentrations of Zn, Pb and Cd were 573.9, 3550.1 and 94.7 mg kg-1, respectively. The accumulation and distribution of Zn and Pb in shoots were similar to those in roots. The highest concentrations of Zn (262.8 mg kg-1) and Pb (219.2 mg kg-1) also occurred in severely polluted plot P1 and decreased as soil extractable HMs decreased. However, the accumulation of Cd in the shoots differed from that of Zn and Pb. The highest accumulation of Cd occurred in P3, which had a moderate extractable Cd concentration in soil compared to the other plots, and the accumulation of this metal was lower in plots with higher (P1 and P2) and lower (P4 and P5) soil extractable Cd (Fig. 1). Furthermore, significantly positive correlation between plant metal accumulation and soil extractable HM was observed, except for Cd in the shoot (Tab. 2). The BFs and TFs of A. nepalensis for Zn, Pb and Cd are given in Tab. 3. The A. nepalensis BFs for Zn and Pb were always >1 in P4 and P5 and >1 for Cd in P3, P4 and P5, which suggested that A. nepalensis acts as an accumulator of Zn and Pb in soils with lower levels of extractable HMs but not in severely polluted soils, and as a Cd accumulator in even moderately Cd-polluted soils. Moreover, there is a significantly negative correlation between BF and soil extractable HMs. The TF of A. nepalensis for Zn was <1 in all sample plots, but >1 for Pb in P4 and for Cd in P3 and P4. A negative correlation between TF and soil extractable HMs was also observed (Tab. 2).
Fig. 1 - Zn (A), Pb (B) and Cd (C) accumulation in shoots and roots of A.nepalensis from the tailing of Munai mine in Lancang county, Yunnan province, south-western China. P1 to P5 are five sampling plots with different distances (200 m, 500 m, 1700 m, 2800 m and 3800 m) to the lead-zinc smelting facility. Vertical bars represent the mean ± SE (n = 6). Different letters above or below bars indicate significant differences among the five sampling plots analyzed at p<0.05 level, according to the LSD test.
Tab. 3 - Bioaccumulation factors (BFs) and translocation factors (TFs) of Zn, Pb and Cd in A.nepalensis from the tailing of Munai mine in Lancang county, Yunnan province, south-western China (mean ± SE, n = 6). P1 to P5 represent five sampling plots with different distances (200 m, 500 m, 1700 m, 2800 m and 3800 m) to the lead-zinc smelting facility. Different letters in the same column indicate significant differences at p<0.05 level, according to the LSD test.
Plot | BF | TF | ||||
---|---|---|---|---|---|---|
Zn | Pb | Cd | Zn | Pb | Cd | |
P1 | 0.20 ± 0.05 a | 0.01 ± 0.01 a | 0.07 ± 0.07 a | 0.44 ± 0.07 a | 0.05 ± 0.02 a | 0.02 ± 0.02 a |
P2 | 0.19 ± 0.06 a | 0.04 ± 0.02 a | 0.58 ± 0.55 a | 0.37 ± 0.12 a | 0.08 ± 0.04 a | 0.45 ± 0.43 ab |
P3 | 0.62 ± 0.20 a | 0.05 ± 0.03 a | 8.63 ± 9.00 b | 0.46 ± 0.07 a | 0.11 ± 0.11 a | 1.51 ± 0.77 b |
P4 | 3.53 ± 0.87 a | 6.50 ± 3.32 b | 8.39 ± 4.88 b | 0.47 ± 0.20 a | 2.33 ± 1.66 b | 1.27 ± 1.05 b |
P5 | 25.87 ± 17.96 b | 8.85 ± 7.58 b | 20.56 ± 5.82 c | 0.90 ± 0.29 b | 0.35 ± 0.19 c | 0.54 ± 0.15 ab |
Discussion
A considerable variation in soil and plant metal concentrations both within and between the sampling plots was observed, reflecting the heterogeneous nature of the polluted area and high individual variability within plants ([24], [23]). Our results indicated that metals were mainly accumulated in the roots of A. nepalensis, particularly in those plots with high metal concentrations (Fig. 1), which suggested strong metal retention during HM transportation from roots to shoots that might be interpreted as a mechanism that assists the plant with metal stress tolerance. Lee et al. ([10]) reported that Alnus hirsuta and Alnus firma absorbed Zn, Pb, Cd, Cu and Cr, accumulating the metals mainly in roots, and Rosselli et al. ([15]) found that Alnus incana showed a promising ability of excluding Zn, Cd and Cu from its above-ground tissues. Similar results were also reported in other woody genera, such as Salix for Pb, Zn, Cd and Cu ([9], [14]), Fraxinus for Zn, Cd and Cu ([15]), and Eucalyptus for Pb, Zn and Cu ([25]).
Accumulation and exclusion are two basic strategies by which plants respond to elevated concentrations of HMs ([3]). A TF (Cshoot/Croot) greater than 1 is common in metal accumulator species, whereas the TF is lower than 1 in metal excluder species ([3], [24]). The TFs (Cshoot/ Croot) and BFs (Cshoot/Csoil) of A. nepalensis for Zn and Pb were lower than 1 in soils severely polluted with Zn and Pb (P1, P2 and P3), indicating that exclusion might be its tolerance strategy. Stoltz & Greger ([20]) showed that most of the plant species growing on mine tailings exhibited restricted translocation of metals to the shoot. Rosselli et al. ([15]) also reported that Alnus incana could be considered a heavy metal excluder and may have a mechanism for avoiding metal uptake by stabilizing HMs in the rhizosphere or keeping them in their roots. Accumulation and translocation of Cd in A. nepalensis were somewhat complex; the TF and BF were greater than 1 in P3 and P4, which had moderate Cd concentrations compared to the other sampling plots, but were lower than 1 under more intensively Cd stressed soils (P1 and P2), which indicated that A. nepalensis may use different tolerance strategies under different levels of Cd stress. The relatively high BF and TF of A. nepalensis for Cd make it a suitable woody candidate for phytoextraction in Cd-contaminated soils. In addition, A. nepalensis accumulated relatively high concentrations of Zn, Pb and Cd in its roots (Fig. 1), which suggested that it might be a good candidate for phytostabilization of these metals.
Phytoremediation, mainly via the mechanisms of phytoextraction and phytostabilization, is a promising and practical technology to remove HMs from polluted environments ([8]). HM hyperaccumulators, which have the ability to absorb and translocate HMs from soil to shoot, have been considered the most promising candidates in phytoextraction. Unfortunately, the majority of plants in nature are sensitive to excessive HMs in the soils. Moreover, the slow growth and small biomass of many HM hyperaccumulators have been reported to limit their application in remediating HM-polluted soils ([7]). In phytostabilization, HM ions are absorbed and confined to roots and to rhizospheric soil, thus inhibiting the mobility of metal ions, restricting their leaching into groundwater and reducing metal bioavailability for entry into the food chain ([22], [6], [26]). Woody plants, especially fast-growing species with large biomasses, have shown advantages and potential for application in phytostabilization ([13], [26]). In the present study, A. nepalensis showed a relatively high capacity to accumulate Zn, Pb and Cd in roots when growing in 5 plots with intensively polluted soils and significant Cd accumulation in shoots when growing in moderately contaminated soils, which suggested its potential for application in the phytoremediation of mining areas.
Conclusions
Adding to a series of desirable properties of A. nepalensis, the promising capacity of this alder species to tolerate and accumulate heavy metals was revealed for the first time in this study. A. nepalensis can accumulate relatively high concentrations of Zn, Pb and Cd in its roots. The BFs for Zn and Pb that were greater than 1 indicated this plant’s phytostabilization strategy and capacity in slightly contaminated soils. Remarkably, its ability to phytostabilize and phytoextract Cd was verified by BFs and TFs both greater than 1, even in moderately Cd-contaminated soils. We therefore suggest that A. nepalensis is a potential candidate for use in the phytoremediation of Zn-, Pb- and Cd-polluted soils in its indigenous range.
Acknowledgments
We thank Zhang XT, Wei YF, Zhang Y, Wang YE, Diao YH, Li XB, Wang L and Qin LP (Laboratory of Conservation and Utilization for Bioresources, Yunnan University, China) for their helps in sample collection and soil, plant samples preparation. We also thank Zhan FD (College of Resources and Environment, Yunnan Agricultural University, China) for his help and guidance in heavy metal analysis. This research was financially supported by the National Natural Science Foundation of China (31160009; 41161083), Key Project of Applied Basic Research of Yunnan (2013FA001), Young Academic and Technical Leader Raising Foundation of Yunnan Province (2012HB006) and Technological Innovation Talents Raising Foundation of Yunnan Province (2012HB053).
Author’s contribution: Yuebo Jing and Hongliang Cui contributed equally to this work.
References
Gscholar
Gscholar
Gscholar
Gscholar
Authors’ Info
Authors’ Affiliation
Hongliang Cui
Tao Li
Zhiwei Zhao
Laboratory of Conservation and Utilization for Bioresources and Key Laboratory of Microbial Diversity in Southwest China, Ministry of Education, Yunnan University, Kunming, 650091 Yunnan (China)
Yunnan Academy of Forestry, Kunming, 650201 Yunnan (China)
Corresponding author
Paper Info
Citation
Jing Y, Cui H, Li T, Zhao Z (2014). Heavy metal accumulation characteristics of Nepalese alder (Alnus nepalensis) growing in a lead-zinc spoil heap, Yunnan, south-western China. iForest 7: 204-208. - doi: 10.3832/ifor1082-007
Academic Editor
Elena Paoletti
Paper history
Received: Jul 20, 2013
Accepted: Nov 20, 2013
First online: Feb 27, 2014
Publication Date: Aug 01, 2014
Publication Time: 3.30 months
Copyright Information
© SISEF - The Italian Society of Silviculture and Forest Ecology 2014
Open Access
This article is distributed under the terms of the Creative Commons Attribution-Non Commercial 4.0 International (https://creativecommons.org/licenses/by-nc/4.0/), which permits unrestricted use, distribution, and reproduction in any medium, provided you give appropriate credit to the original author(s) and the source, provide a link to the Creative Commons license, and indicate if changes were made.
Web Metrics
Breakdown by View Type
Article Usage
Total Article Views: 55820
(from publication date up to now)
Breakdown by View Type
HTML Page Views: 47326
Abstract Page Views: 2699
PDF Downloads: 4604
Citation/Reference Downloads: 32
XML Downloads: 1159
Web Metrics
Days since publication: 3920
Overall contacts: 55820
Avg. contacts per week: 99.68
Article Citations
Article citations are based on data periodically collected from the Clarivate Web of Science web site
(last update: Feb 2023)
Total number of cites (since 2014): 7
Average cites per year: 0.70
Publication Metrics
by Dimensions ©
Articles citing this article
List of the papers citing this article based on CrossRef Cited-by.
Related Contents
iForest Similar Articles
Research Articles
Effect of soil-applied lead on mineral contents and biomass in Acer cappadocicum, Fraxinus excelsior and Platycladus orientalis seedlings
vol. 10, pp. 722-728 (online: 27 July 2017)
Research Articles
Analysis of biometric, physiological, and biochemical traits to evaluate the cadmium phytoremediation ability of eucalypt plants under hydroponics
vol. 10, pp. 416-421 (online: 24 March 2017)
Research Articles
Effect of cadmium (Cd) and lead (Pb) soil contamination on the development of Hymenoscyphus fraxineus on Fraxinus excelsior and F. angustifolia seedlings
vol. 16, pp. 307-313 (online: 09 November 2023)
Review Papers
Heavy metals and woody plants - biotechnologies for phytoremediation
vol. 4, pp. 7-15 (online: 27 January 2011)
Review Papers
A review of the performance of woody and herbaceous ornamental plants for phytoremediation in urban areas
vol. 13, pp. 139-151 (online: 14 April 2020)
Research Articles
Using nano-scale Fe0 particles and organic waste to improve the nutritional status of tree seedlings growing in heavy metal-contaminated soil
vol. 14, pp. 447-455 (online: 28 September 2021)
Research Articles
Heavy metal (Zn, Pb, Cd) concentration in soil and moss (Pleurozium schreberii) in the Brynica district, southern Poland
vol. 4, pp. 176-180 (online: 11 August 2011)
Review Papers
Monitoring the effects of air pollution on forest condition in Europe: is crown defoliation an adequate indicator?
vol. 3, pp. 86-88 (online: 15 July 2010)
Research Articles
Mapping Cadmium distribution in roots of Salicaceae through scanning electron microscopy with x-ray microanalysis
vol. 4, pp. 113-120 (online: 01 June 2011)
Research Articles
Preliminary results of the tolerance to inorganic contaminants and phytoextraction potential of twelve ornamental shrub species tested on an experimental contaminated site
vol. 11, pp. 442-448 (online: 18 June 2018)
iForest Database Search
Search By Author
Search By Keyword
Google Scholar Search
Citing Articles
Search By Author
Search By Keywords
PubMed Search
Search By Author
Search By Keyword