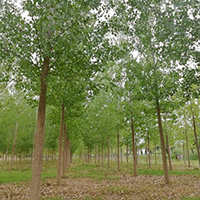
A review of the performance of woody and herbaceous ornamental plants for phytoremediation in urban areas
iForest - Biogeosciences and Forestry, Volume 13, Issue 2, Pages 139-151 (2020)
doi: https://doi.org/10.3832/ifor3242-013
Published: Apr 14, 2020 - Copyright © 2020 SISEF
Review Papers
Abstract
Urban and periurban areas are often contaminated by several pollutants. Phytoremediation is considered to be an effective and eco-friendly strategy for the restoration of these contaminated lands. For this purpose, the exploitation of ornamental plants could be an additional option, due to their positive impact on the landscape. In this paper, we reviewed a selection of species which have been proposed for utilization in phytoremediation. Several tree species have been introduced in the past into urban environments for parks, gardens and avenues, with a selection studied for their capacity to absorb, tolerate, and translocate contaminants. Shrubby and herbaceous species are also commonly exploited for their ornamental features and are now studied for phytoremediation purposes. The responses of several effective species to the presence of heavy metals or dangerous organic compounds in the growth substrate are examined in this paper.
Keywords
Introduction
Environmental pollution is an increasing global problem. Contaminants vary depending upon their source and the type of ecosystem involved. Urban areas can be affected by several organic and inorganic contaminants, which negatively impact soils, water and the atmosphere ([10]). The study of the effect of contamination by pollutants on these urban environments and potential solutions to the problems associated with soil contamination and rehabilitation dates to the last few decades ([126]).
Urban and periurban soils are often polluted as consequence of human activities. Brownfield sites, mainly located in periurban sites, reflect industrial heritage and are probably the most common scenarios where urban and periurban contaminated soils are found ([117]). In general, heavy metals are the major contaminants: lead is commonly found near roads and associated with zinc and cadmium ([47]); excessive copper is frequently detected in soils used for a long period as agricultural land, especially vineyards ([16]), and chromium is a residual of some industrial activities ([140]). Organic pollutants can also injure urban soils by direct contamination, or after initial emission into the atmosphere followed by transport in both gaseous and particulate forms, and subsequent accumulation in soils after dry and wet atmospheric deposition ([19]). Urban soils may also be very different to more natural soils, due to lower organic fraction, water drainage and aeration, different pH value, microorganism content and the presence of anthropic material. These features can change the performance of these soils with respect to pollutants ([126], [112]). As stated by Cunningham & Berti ([34]) “new technologies are needed to address numerous contaminants, especially those that are neither volatile nor mobile in soil solution”. Plants have been proposed to mitigate the dangerous effects of pollutants, with phytoremediation recognized as a promising technology for the recovery of contaminated environments ([109]). Phytoremediation could be successfully exploited in urban territories; in these contexts, many herbaceous and some woody species (including forest species) are suitable for planting because of their ornamental features and adaptability to inhabited areas. Furthermore, plants are useful sensors to identify environmental contamination and potential exposures to pollutants ([59]). In fact, some of these species show the capacity to absorb, hold or translocate specific contaminants; moreover, these ornamentals pose little threat to food chain contamination, and can be appreciated by the resident populations for their positive impact on landscape.
In the more circumscribed field of flowerbeds and urban green plantations, special mixtures of topsoil are commonly used and rules have to be followed with regards to the presence of contaminants ([62]). Risks are higher in allotments, since contaminants could be transferred to the food chain ([111], [66]). Attention has to be paid to parks, playgrounds, kindergartens and urban areas where people come into close contact with soil ([2], [30], [37], [71], [83], [84]). In these areas, selected ornamental plants may play an important role in reducing the presence of pollutants, while at the same time giving a pleasant temporary decoration. Botanists have elaborated several definitions of ornamental plants; these can be defined as plants that have highly ornamental features such as ornamental flowers, fruits or foliage ([74]). Nevertheless, we must also consider that the ornamental value of a plant may vary according to the different tastes and traditions of each country.
The focus of our attention is the “original” soils, and the present article is aimed primarily at suburban areas, which can be even heavily contaminated, due to their past uses. If the plants chosen to be used in remediation are tree species, several selection criteria have to be taken into account ([33]), including problems linked to climate change ([107], [69]) and the preferences expressed by the resident populations (these inclinations are generally for plantations with high level of biodiversity - [22]). Also, it is always advisable to consider all of the actions related to the sustainability of plantations ([44]) and the analysis of costs for their periodic removal and disposal ([8], [73], [32], [110], [133]).
In this context, we might also consider the possible use of tree planting for short-rotation coppicing, by which a profitable recovery of the used plants can be performed. For instance, willows may be exploited for this purpose, since these species display high ornamental value and are suitable for bioenergy production combined with potential for phytoremediation ([53], [55]). Therefore, the choice of species to be used for phytoremediation includes consideration of criteria such as respect for biodiversity, easy harvesting management, by-product utilisation and possible economic returns.
After their use for soil remediation, all plants (woody and herbaceous) must be harvested and treated, since the content of pollutants is an important concern. Several solutions are available for this purpose, including compacting, composting, biogas production and pyrolysis ([11], [97], [46]). The easiest procedure is incineration of biomass, with recovery of residual heavy metals. When ornamental flowers are used, cut flowers with limited contaminants content can be eventually sold.
An overview is provided of the most effective woody and herbaceous plants (tree species, shrubs and herbaceous flowers) for the remediation of urban and suburban areas, through analysis of recent literature illustrating how these species react when facing either inorganic (heavy metals) or organic contaminants. This review is specifically addressed to a selection of species suitable for exploitation in urban environments, due to their adaptability, ornamental characteristics and appreciation by resident populations; the proposed selection, albeit large, is mainly focused on the species best suited to European and American environments.
Several tree species have been successfully used in urban parks, gardens and avenues; for instance, in Europe pines, cypresses, poplars, willows, birches, sycamores and lindens are widely planted ([92]). Numerous recent studies demonstrated that some tree species have a good attitude to tolerate, absorb and remove specific contaminants from the soil (reviewed by [85], [99]). Trees can enhance the aesthetic quality of urban landscape ([26]), simultaneously providing other functions, such as improved air quality ([96]), reduction of noise pollution ([100]), mitigation of waterlogging ([82]) and reducing heat island effects ([113] - Fig. 1, Fig. 2).
Fig. 1 - In vitro culture for the rapid mass propagation of plants to be exploited in phytoremediation: (a) multiclonal culture of Populus alba; (b) in vitro-rooted plantlet of Salix alba.
Fig. 2 - Woody species for phytoremediation: (a) a poplar plantation in an urban site (outskirts of Florence); (b) Betula pendula.
A great variety of herbaceous and shrubby species have been analysed for phytoremediation purposes (reviewed by [81] - Fig. 3), but many still remain to be studied. Furthermore, plants’ association, with its huge possibilities of combinations, offers interesting perspectives and is therefore discussed.
Fig. 3 - Two widespread ornamental species studied and proposed for phytoremediation purposes: (a) Chrysanthemum; (b) Nerium oleander.
The choice of ornamental woody and herbaceous plants for urban (and periurban) environments should also take into account some factors. In these sites (often smaller areas compared to the countryside), the problem of the scattering of leaves could be more relevant; especially when using trees, and for species that translocate pollutants to the leaves, leaves should be periodically collected and treated. Moreover, due to the greater anthropic presence in urban site and transport constraints, it is probably opportune to choose species that have less maintenance needs and leaves which are easier to collect at the end of the leaf life-cycle.
Heavy metals
Pollution from industrial emissions, effluents and solid discharges are the main source of an abnormal high presence of heavy metals in soils. In general, numerous human activities result in the emission of these harmful pollutants that enter into the biosphere through wastes (emission, waste-water and waste solid), including municipal wastes in agriculture and excessive use of fertilizers. Several plant species have the capacity to absorb and translocate specific metals; a selection of woody and herbaceous species, which could be identified as “multipurpose species”, are reviewed below, for their possible utilisation in metal remediation coupled to considerable ornamental features (Tab. 1, Tab. 2).
Tab. 1 - Ornamental plants for the phytoremediation of heavy metal: trees and shrubs. (A): accumulation; (T): translocation.
Group | Species | Pollutants | A / T | References |
---|---|---|---|---|
Trees | Acacia mangium | Pb | A | [138] |
Acer cappadocicum | Pb | A | [1] | |
Azadirachta indica | Pb | A | [138] | |
Betula pendula | Zn | A,T (partial) | [108] | |
Cinnamomum camphora | Zn | A,T | [141] | |
Eucalyptus camaldulensis | Pb | A | [138], [95] | |
Cd | T | |||
Fraxinus excelsior | Pb | A | [1] | |
Mimosa cesalpiniaefolia | Pb | A | [1] | |
Platycladus orientalis | Pb | T | [1] | |
Populus alba | Cd,Pb | A | [60], [139] | |
Populus deltoides | As | A | [64] | |
Cd | A | [139] | ||
Populus nigra | Cd | A | [139] | |
Populus trichocarpa | Cd | A | [139] | |
Populus × canadensis | Cd | A | [139] | |
Populus × euramericana | Cu | A | [13] | |
Zn | T (partial) | [38] | ||
Cr, Fe | T (partial) | [48] | ||
Populus × generosa | Cd | A | [139] | |
Salix dasyclados | Cd | A | [70] | |
Zn | T | [130] | ||
Salix fragilis | Cd, Zn | T | [89] | |
Salix miyabeana | Zn | T | [36] | |
Salix nigra | Ag | A | [55] | |
Salix schwerinii | Cd, Zn | T | [89] | |
Salix viminalis | Cd | A | [70] | |
Zn | T | [130] | ||
Senna siamea | Pb | A | [138] | |
Shrubs | Buddleja asiatica | Pb | T | [131] |
Buddleja paniculata | Pb | T | [131] | |
Catharanthus roseus | Ni, Pb | A | [120] | |
Euonimus japonicus | Cd | A | [141] | |
Euphorbia milii | Cr | T | [104] | |
Ligustrum vicaryi | Cd | A | [141] | |
Lonicera japonica | Cd | T | [78] | |
Loropetalum chinense | Cd | A | [141] | |
Osmanthus fragrans | Cd | A, T | [141], [137] | |
Pb | T | [137] | ||
Rhapis excelsa | Cd | A | [143] | |
Ricinus communis | Ni | A | [3] |
Tab. 2 - Ornamental plants for the phytoremediation of heavy metals: herbaceous species. (A): accumulation; (T): translocation.
Group | Species | Pollutants | A / T | References |
---|---|---|---|---|
Herbaceous | Althaea rosea | Cd | T | [77] |
Pb | A | [77] | ||
Alternanthera bettzickiana | Cd, Pb | T | [124] | |
Alyssum maritima | Cr | T | [17] | |
Amaranthus caudatus | Ni | T | [15] | |
Cd | T | [23] | ||
Aptenia cordifolia | Cr | T (partial) | [17] | |
Calendula officinalis | Cd | A | [77] | |
Cr | T (partial) | [103] | ||
Cu | T | [49] | ||
Canna indica | Pb, Zn Cr, Ni, Cd |
T | [121] | |
A | [121] | |||
Chlorophytum comosum | Cd | T | [135] | |
Zn | T | [123] | ||
Gomphrena globosa | As | A | [116] | |
Helianthus annuus | Cu | T | [45] | |
Cd | T | [14] | ||
Ni | T | [94] | ||
As | T | [105] | ||
Hydrangea paniculata | Cu | T | [45] | |
Impatiens balsamina | Cr | T (partial) | [91] | |
Iris lactea | Cd | A | [57] | |
Iris pseudacorus | Cr, Zn | A | [21] | |
Mesembryanthemum crystallinum | Ni | T (partial) | [5] | |
Mirabilis jalapa | Cr | T | [91] | |
Salvia splendens | Cd | T | [14] | |
Polianthe tuberosa | Cd | T | [102] | |
Pteris vittata | As | T | [142] | |
Sedum alfredii | Zn | A | [28] | |
Syngonium sp. | As | A | [63] | |
Tagetes erecta | Cd | A | [14] | |
Ni | T | [15] | ||
As | T | [105] | ||
Tagetes patula | As | A | [63] | |
Tagetes erecta × patula | As | T | [31] | |
Vinca rosea | Cr | T | [40] | |
Zinnia elegans | Pb, Cr | T | [41] | |
As | A | [116] |
Woody species
Among tree species traditionally used in urban and periurban plantations, a limited number showed suitability for phytoremediation purposes. Analysis of the recent literature indicates that the most promising are some species of the Salicaceae family (Salix spp., Populus spp.), but some others also show significant pollution resistance traits, such as Ailanthus altissima, Robinia pseudoacacia, Betula pendula, Carpinus betulus, Ginkgo biloba and Platanus hispanica ([35]). Several tree species (mainly willows and poplars) are not hyperaccumulators, but do exhibit traits of high interest, such as fast growth and high production of biomass, easy propagation, a deep root system and the capacity to uptake and translocate a significant amount of metal contaminants in the soil to the shoots ([129], [51]). Poplars in particular, display a number of different characteristics useful towards environmental protection, which include phytoremediation, especially in combination with short rotation forestry and landscape restoration ([43]). Woody species may also be utilised for the plantation of green belts around contaminated lands. Eucalypts, willows and poplars are all fast-growing trees with short rotation coppice systems that could be successfully utilised for this purpose ([101]). This phytoremediation would have to be undertaken in consideration of the need to harmonize these choices with the landscape character, as generally requested by the resident populations ([12]).
The effect of lead (Pb) has been assessed in one-year-old potted seedlings of Cappadocian maple (Acer cappadocicum), European ash (Fraxinus excelsior) and Oriental aborvitae (Platycladus orientalis). Increasing Pb application in the soil (from 100 to 500 mg kg-1) did not affect the dry weight of roots of all species, while a gradual decrease was detected in leaves and shoots, with the highest inhibition in P. orientalis. This species, however, showed the highest translocation factor values, tolerance index and bioconcentration factor, indicating a possible use of this conifer species for remediation of Pb-polluted soils ([1]). The bioconcentration factor (BF) is defined as the ratio of metal(loid) concentration in aerial biomass to that in soil, and the translocation factor (TF) the ratio of metal(loid) concentration in shoots to that in roots, both factors taking values >1 in accumulators and <1 in excluders; the tolerance index (TI) is the percent of the organ’s growth of the treated plant compared to the growth of the control plant ([88], [128]). Three leguminous woody species, Mimosa caesalpiniaefolia, Erythrina speciosa and Schizolobium parahyba, were tested in a lead-contaminated area. While M. caesalpiniaefolia did not show symptoms of Pb toxicity, the other two species exhibited reduced shoot biomass yield, leaf area and height. The increase of Pb concentrations in soil led to augmented Pb concentration in shoots and roots, but most of the Pb accumulated in the roots, and only a small fraction was translocated to the aboveground parts of the plant. Mimosa showed the highest Pb tolerance and phytostabilisation potential in lead-contaminated soils ([106]).
In a study to identify candidate species among fast-growing trees for remediating Pb-contaminated soils ([138]), hydroponic cultures of cuttings from Acacia mangium, Azadirachta indica, Eucalyptus camaldulensis, and Senna siamea, were tested in increasing Pb concentrations. All species showed high Pb tolerance (over 78%) but low TF (<1) for all treatments (10, 30, and 50 mg L-1). Based on these indices, A. mangium and E. camaldulensis were found to be good candidate species for Pb remediation ([138]).
Willow (Salix nigra) showed a moderate tolerance to silver (Ag) in a hydroponic experiment with increasing AgNO3 concentrations, observing a significant reduction of biomass production with AgNO3 0.027 μM, but also adaptation signals over a longer timeline ([54]).
Eastern cottonwood (Populus deltoides) was evaluated for arsenic (As) tolerance and phytostabilization potential, by exposure to various As levels in soil (control, 5, 10, 15, and 20 mg kg-1) in a 9-month pot experiment. Plant height stress tolerance index (TI) significantly decreased with increasing As levels, while indices related to root length and dry matter were not affected. TF and BF were less than 1.0, but root and shoot As content significantly increased with increasing As concentrations ([64]). The effect of high copper (Cu) concentrations was investigated on poplar woody cuttings (Populus × euramericana, clone “Adda”), finding that increasing levels of Cu up to 100 μM resulted in a general reduction of plant growth and that the metal was mainly accumulated in the root system at all Cu levels ([13]). Several poplar species (Populus alba, P. deltoides, P. nigra, P. trichocarpa, P. × generosa, P × canadensis) showed the capacity to accumulate cadmium (Cd), albeit with different effectiveness ([139]). Poplars also demonstrated phytoextraction capacity for zinc (Zn - [38]).
Cadmium accumulation was also demonstrated in Cinnamomum camphora, that showed the maximum Cd content in stems and leaves ([141]).
In a greenhouse experiment on ornamental plants, four shrubs (Osmanthus fragrans, Ligustrum vicaryi, Loropetalum chinense var. rubrum, and Euonymus japonicus cv. Aureo-mar) were tested in the presence of Cd. The results showed that these species can grow normally at Cd soil concentrations lower than 24.6 mg kg-1. The metal accumulated principally in the roots, with the highest amount detected in Euomymus ([141]).
Rhapis excelsa, Camellia polyodonta and C. gigantocarpa were tested for soil Cd absorption in a pot experiment with different Cd treatments (10, 25 and 50 mg kg-1). The three species never showed any toxic symptom and grew well at all Cd concentrations. Cadmium contents was higher in the roots than in the stems and leaves. At 50 mg kg-1 Cd concentration, the Cd content in the roots of Rhapis excelsa was the highest amongst all the tested species and 7.05 times higher than that at 10 mg kg-1 Cd concentration ([143]). Lonicera japonica plants exposed to Cd concentrations up to 50 mg L-1 did not show significant differences (compared to control) in height and dry biomass of leaves and roots. TIs were all above 0.8 and the high BF and TF justified the proposal to include the species in the list of potential Cd accumulators ([78]).
The ornamental shrub Euphorbia milii tolerated up to 75 mg of applied Cr per Kg soil, and was efficient in translocating Cr from roots to shoots. Plant death occurred when higher metal concentrations were used ([104]).
Buddleja asiatica is known to display a high accumulation capacity and tolerance for lead. This species, and the related ornamental B. paniculata, were therefore investigated in a hydroponic culture, in the presence of 10 or 20 mg L-1 Pb. Both species showed increased biomass and Pb concentrations in the roots of 12.1 and 21.7 mg kg-1, respectively. In a 3-month pot experiment, using three different soils with various Pb levels (10.6, 31.3, and 89.1 mg kg-1) the two species of Buddleja had a slight decrease in survival rates at the highest Pb concentration, but a general regular growth. In a 6-month field trial experiment conducted in Pb-contaminated sites (Pb content: 95-101 mg kg-1), both Buddleja species showed 100% survival, increased biomass production and phytoextraction capacity (TF) from 1.1 to 2.3 ([131]). Ricinus communis, as well, showed the capacity to uptake nichel (Ni) from contaminated soils, and was therefore classified as an accumulator ([3]); this species also demonstrated a great potential for Cd removal, due to its features of fast growth, high biomass and considerable absorption and accumulation ([61]).
It is more common for a soil to be affected by the pollution of a mix of heavy metals; some case studies are therefore reported below concerning woody plants.
In a pot experiment with seven willow clones, significant differences between clones were found in cadmium and zinc accumulation. Cd and Zn were transferred from roots to aboveground tissues (mainly leaves), leading to the conclusion that willows are suitable phytoextractors of moderately contaminated soils ([130]). Another study tested the ability of five woody species to extract heavy metals (copper, zinc or cadmium) from a polluted soil. Salix viminalis and Betula pendula had already demonstrated phytoextraction ability for Zn and Cd, while the phytoextraction capabilities of Alnus incana, Fraxinus excelsior and Sorbus mougeotii were unknown. The results suggest that none of these species transferred Cu to the shoots. Salix and Betula were able to transfer Zn and Cd to leaves and twigs, while Alnus, Fraxinus and Sorbus excluded them from their above-ground tissues ([108]). A pot experiment involving Salix miyabeana grown in brownfield soils differentially contaminated with Ag, Cu and Zn (up to 113.60, 47.50, and 117.00 mg kg-1 respectively), demonstrated a potential capability for phytoremediation, since a high concentration of Zn (119.96 ± 20.04 mg kg-1) was detected in above-ground plant tissues at the end of the treatment ([36]).
Five species of Salix were tested in a pot experiment to compare their capacity to extract and accumulate Cd, Zn, Cu, Ni, Pb, and chromium (Cr). Salix schwerinii “Christina”, S. dasyclados “Loden” and S. fragilis “Belgisch Rood” showed the highest Cd and Zn accumulation and were therefore considered good candidates for remediation ([89]). In an experiment on a strongly polluted soil (up to 18 mg Cd kg-1, 1400 mg Cu kg-1, 500 mg Pb kg-1 and 3300 mg Zn kg-1), Salix viminalis demonstrated a high translocation of Cd (≥80 mg kg-1) and zinc (≥3000 mg kg-1) to the leaves alongside reduced growth. In contrast, when grown in a moderately polluted soil (2.5 mg Cd kg-1 and 400 mg Zn kg-1), S. viminalis extracted 0.13% of total Cd and 0.29% of total Zn per year and exhibited vigorous growth ([65]). In another study on eight Salix viminalis clones and one S. alba clone, differences emerged between clones in biomass production and accumulation efficiency, with two S. viminalis clones demonstrating a superior capacity to accumulate five heavy metals (Cd, Cu, Hg, Pb, Zn - [93]).
Poplars also showed some potential for phytoextraction of chromium and iron ([48]), cadmium and lead ([60]).
The accumulation of heavy metals has been assessed in leaves of some ornamental trees and shrubs used in districts of Turkey affected by high heavy metal pollution, finding significant differences among the tested species. The highest concentrations (mg kg-1) of Zn, Cu, Cd and iron (Fe) were observed in Cedrus libani (618.0), Betula alba (106.3), Salix alba (24.5) and Eleagnus angustifolia (0.3), while the highest Ni (6.4) and Pb (3.8) contents were found in Pyracantha coccinea ([56]).
A pot experiment was carried out on osmanthus (Osmanthus fragrans var. thunbergii), cultured in substrate supplemented with different concentrations of Cd, Pb, Zn, and Cu. The species showed high Cd and Pb transfer efficiencies and a limited transfer of Zn and Cu in the presence of Cd, suggesting the possible utilization of osmanthus in phytoremediation applications ([137]).
Herbaceous species
Cadmium is one of the most widespread contaminating metals in soils. Its action was investigated on three ornamental plants, Tagetes erecta, Salvia splendens, and Abelmoschus manihot, finding a little effect on seed germination of the three species and on shoot elongation of S. splendens, but a significant inhibitory effect on root elongation of all the tested plants and on shoot elongation of T. erecta. The calculated Cd-tolerance indices led to the conclusion that A. manihot was the most tolerant plant to Cd while S. splendens the most sensitive ([134]). Conversely, Bosiacki ([14]) found high Cd accumulation in leaves and shoots of Salvia splendens, as well as in inflorescences of Helianthus annuus, which is one of the most studied ornamental species for remediation purposes, while Tagetes erecta proved a moderate capacity to extract and accumulate Cd, with the greatest amount found in roots, then in leaves and shoots, and the lowest in inflorescences ([14]). Five concentrations of Cd (0, 25, 50, 75 and 100 mg kg-1 soil) were tested with three varieties of tuberose, finding that this metal did not produce any toxic macroscopic symptoms in all the three varieties. Having shown Cd accumulation in the shoots higher than 100 μg g-1 dry weight and a ratio of Cd >1 in the shoots to bulbs, this species has to be considered as a potential effective Cd accumulator ([102]). Chlorophytum comosum is a potential Cd accumulator; in a pot experiment it showed a TI above 100 in soil Cd concentration of 100 mg kg-1, and at Cd concentration up to 200 mg kg-1, the Cd content in roots and aboveground tissues reached 1522 and 865 mg kg-1, respectively ([135]). A detailed work on the relation between Canna indica and Cd, demonstrated its considerable potential in cadmium accumulation, but the root concentration factor was higher than the BF, indicating a limited translocation ([118]). Calendula officinalis was found to grow normally in soils containing 100 mg kg-1 Cd, with high metal accumulation in roots and shoots. In a hydroponic culture, for Althaea rosea the highest Cd accumulation was detected in shoots, and for both Calendula officinalis and Althaea rosea, a good accumulation capacity and tolerance to Pb were also observed ([77]). Iris lactea var. chinensis was found to accumulate Cd in leaves and roots after treatment with a hydroponic culture with 0 to 160 mg L-1 Cd treatment, showing a TI higher than the value detected in the other species tested, I. tectorum ([57]).
The effects of increasing doses of Ni (up to 300 mg dm-3 substrate) were investigated in three selected ornamental plants: Tagetes erecta, Helianthus annuus, and Amaranthus caudatus, finding that the highest amounts of Ni were accumulated in leaves of tagetes and amaranth, and in inflorescences of sunflower. Globally, tagetes showed the highest Ni uptake at concentrations of 25 and 50 mg dm-3, while for the substrates with an addition of 75, 150 or 300 mg Ni dm-3, the greatest accumulation was recorded in amaranth ([15]). An interesting ornamental halophyte, Mesembryanthemum crystallinum, was compared to the model species Brassica juncea, growing the plants for 3 months on a soil containing 0, 25, 50, and 100 mg kg-1 NiCl2. Ni reduced the growth activity of both species, but to a lower extent in M. crystallinum. Ni accumulated mainly in roots and the fraction translocated to shoots was higher in M. crystallinum than in B. juncea ([5]). Catharanthus roseus irrigated for 60 days with aqueous solutions of nickel and lead showed high accumulation of the two metals by roots, and to a lesser extent in stems and leaves ([120]).
Chlorophytum comosum seedlings treated with Zn showed inhibition of root length and fresh and dried plant weight at all the tested Zn concentrations (from 200 to 2000 mg kg-1), while the length of aboveground tissues and the volume of roots declined with the Zn dose. TI was above 50 at Zn concentrations lower than 600 mg kg-1 ([123]).
Aptenia cordifolia, Brassica juncea, Brassica oleracea, and Alyssum maritima were studied for their capacity to uptake and translocate hexavalent chromium (VI) supplied by irrigation. Increases in the Cr concentration significantly enhanced both accumulation and translocation of the metal in the roots and shoots of the tested species, with highest values recorded in the shoots of Alyssum maritima and in the roots of Brassica juncea ([17]). In a study on exposure to Cr of four ornamental plants, calendula, chrysanthemum, aster and dahlia, the metal caused a drastic reduction of plant growth at 10 mg kg-1, and at 25 mg kg-1 was responsible of a diffuse mortality in chrysanthemum. Overall, only calendula could be considered a possible candidate for phytoremediation of soils contaminated with low level of Cr ([103]). In another experiment on three ornamental species cultured in pots containing substrate with four Cr concentrations, Impatiens balsamina showed a decline in the biomass as the dose of Cr increased, while in Mirabilis jalapa and Tagetes erecta the four treatments did not impact growth; TF and BF of M. jalapa were greater than 1, indicating this species is a good candidate for the remediation of Cr-polluted soils ([91]). In Vinca rosea grown in pots containing soil with levels of chromium from 10 to 60 mg kg-1, plant height, fresh and dry weight decreased with high contamination levels of chromium. TFs were found to be lower than 1 for low metal concentrations and higher than 1 with Cr concentrations from 30 to 60 mg kg-1 ([40]).
In the presence of Pb, the remediation potential of Vinca rosea was higher than 1 at Pb concentrations from 20 to 40 mg kg-1 and lower with 50 to 90 mg kg-1 ([41]). Similar results were obtained with zinnia (Zinnia elegans) grown in pots containing soils with different levels of lead and chromium. Plants grown in the presence of lead were healthier compared to plants grown in Cr-contaminated soils. TF was also higher in Pb-contaminated soils ([42]).
For copper remediation, Calendula officinalis showed a high tolerance (up to 400 mg kg-1) to copper contamination, with the maximum Cu accumulation (4.67 and 3.99 mg g-1 in leaves and roots, respectively) in soil treated with 300 mg Kg-1, a level considerably higher than the amount of 1 mg g-1 which defines Cu hyperaccumulators (even the TF was >1 at all Cu doses - [49]).
For arsenic remediation, Tagetes patula and Syngonium sp. were tested in pots with soil containing As up to 10 mg kg-1. The plants showed significant As accumulation, particularly in roots, with an average TF of 0.91 for marigold and 0.75 for arum ([63]). A further experiment on a triploid hybrid Tagetes erecta × patula showed that arsenic was found mostly in leaves (46.2%) with the lowest As content (5.8%) in flowers. The hybrid plants continued to grow vigorously in the As-contaminated substrate ([31]). Several ornamental plant species were tested for their potential for As remediation in a hydroponic system: iris (Iris savannarum), switchgrass (Panicum virgatum), Tithonia rotundiflora, Coreopsis lanceolata, sunflower (Helianthus annuus), and marigold (Tagetes erecta). Tithonia and Coreopsis showed respectively 85% and 65% reductions in dry weight at 0.75 mg L-1 As concentration. At the highest As rate, marigold and sunflower had uptake ratios of 7.4 and 16.6, respectively, and TF near one, allowing consideration of these species as interesting candidates for As phytoremediation ([105]).
The effects of As were tested under hydroponic conditions on two other flowering species, Gomphrena globosa and Zinnia elegans. Arsenic principally accumulated in the roots, followed by leaves, stems and flowers, indicating that these species were arsenic tolerant plants but not potentially As-remediating ([116]).
For the phytoremediation of mixed heavy metals, a study on Alternanthera bettzickiana, a species commonly used as an ornamental edging plant, showed a good accumulation of Cd and Pb at concentrations up to 1.0 mM, with total uptake of both metals higher in shoots than roots ([124]). Based on BF and TF, also Canna indica was indicated to be a good accumulator of Cd, Pb, Ni, Zn, and Cr, with high TF for Ni and Cr ([121]). The macrophyte Iris pseudacorus is considered to be a candidate for Cr rhizofiltration and Zn phytoextraction, having shown a good tolerance and accumulation capacity towards these two metals. Plants grown in a nutrient solution containing ZnCl2 or CrCl3 from 0 to 200 μg ml-1 survived and accumulated Cr and Zn in all tissues ([21]). In a greenhouse experiment, Hydrangea paniculata and Helianthus annuus accumulated significant amounts of Cu and Pb. Helianthus showed high accumulation of heavy metals in the shoots and efficacious translocation to the leaves, while Pb was not as easily taken up and translocated as Cu. Hydrangea stored more metals in stems than in leaves, showing a lower translocation ability than Helianthus ([45]). Tanacetum vulgare showed environmental adaptability on high industrial pollution and an interesting capacity of mercure and lead uptake ([119]).
Organics
Phytoremediation of organic contaminants generally involves few classes of compounds, which are principally chlorinated solvents, petroleum hydrocarbons (PHCs), polycyclic aromatic hydrocarbons (PAHs), polychlorinated biphenyls (PCBs) and explosives. Contamination of soils with such products can have several causes, the main ones being uncontrolled industrial activity, intensive farmland exploitation and percolation of polluted waters of various origins. The most common contaminated soils are probably former industrial sites, which may display residual pollutants at different soil depths.
Over recent years, positive results have emerged regarding the capacities of several plant species to degrade specific organic compounds. According to Shimp et al. ([115]) it is fundamental to understand the physical, biological, and chemical relationships that determine the fate of each organic contaminant in the rhizosphere.
In this section some case examples concerning ornamental woody and herbaceous species are illustrated (Tab. 3).
Tab. 3 - Ornamental plants for the phytoremediation of organic compounds. (B[a]P): benzo[a]pyrene; (DDT): dichlorodiphenyltrichloroethane; (HCH): hexachlorocyclohexane; (PCB): polychlorinated biphenyls; (PAH): polycyclic aromatic hydrocarbons; (TCE): trichloroethylene; (TPH): total petroleum hydrocarbons.
Group | Species | Pollutants | References |
---|---|---|---|
Trees | Itea virginica | Oryzalin | [6] |
Populus deltoides × nigra | TCE | [39] | |
Dioxane | [4] | ||
PCB | [76] | ||
Atrazine | [18] | ||
Populus hybrids | HCH | [9] | |
Salix alba | Oryzalin | [6] | |
Salix miyabeana | PAH, PCB | [52] | |
Salix sachalinensis | PAH, PCB | [52] | |
Shrubs | Cytisus striatus | HCH | [7] |
Nerium oleander | Fluoride | [67] | |
Ricinus communis | DDT | [61] | |
Herbaceous | Aloe vera | Formaldehyde | [75] |
Aster amellus | Dyes | [68] | |
Canna indica | Triazophos | [29] | |
Chrisanthemum morifolium | Benzene, Formaldehyde |
[75] | |
Crassula portulacea | Benzene | [75] | |
Dianthus chinensis | Sulfur dioxide | [75] | |
Echinacea purpurea | TPH | [80] | |
Festuca arundinacea | TPH | [80] | |
Gaillardia aristata | TPH | [80] | |
Gaillardia grandiflora | Dyes | [25] | |
Impatiens balsamina | TPH | [20] | |
Iris lactea | TPH | [27] | |
Medicago sativa | TPH | [80] | |
Mirabilis jalapa | B[a]P | [122] | |
Portulaca grandiflora | Dyes | [25] | |
Portulaca oleracea | Fluoride | [67] | |
Tagetes patula | Dyes | [25] | |
B[a]P | [122] |
Woody species
In a soil contaminated with a mix of PAHs, PCBs and heavy metals, two clones of different willow species (Salix sachalinensis SX61 and S. miyabeana SX64) gave encouraging growth and survival results after a single growing season planted in soils with high concentrations of both organic and heavy metal contaminants ([52]). Salix alba, S. gracilistyla var. melanostachys and Itea virginica were treated for 9 days with a 4 mg L-1 suspension of two herbicides (isoxaben and oryzalin). Isoxaben reduced the growth rate of white willow and I. virginica, while both herbicides reduced the growth index for S. gracilistyla. The final dry weight was lower for all taxa when exposed to both herbicides, but the set of data suggest that S. alba and I. virginica display some attitude in the remediation of oryzalin ([6]).
In hydroponic studies, hybrid poplar cuttings (Populus deltoides × nigra) removed 54.0% of dioxane (1.4-Dioxane), a persistent environmental pollutant, indicating the potential of this species in the phytoremediation of sites contaminated by dioxane and other hydrophilic pollutants ([4]). P. deltoides × nigra showed also the capacity to accumulate PCBs, observing that mono- and di-chlorinated congeners were primarily translocated from the roots to the secondary stems, tri-chlorinated to the main stem but not farther, and tetra-chlorinated were bound strongly to root tissues ([76]). With the same hybrid, the capacity to uptake, hydrolyze and dealkylate atrazine to less toxic metabolites was detected by Burken & Schnoor ([18]).
Nerium oleander (Fig. 3b) resulted able to remove 92% of fluoride from a 10 mg L-1 NaF solution, within 15 days ([67]). Ricinus communis showed a great potential for removing dichlorodiphenyltrichloroethane (DDT) from contaminated soils, with different effectiveness depending on the genotype ([61]).
Herbaceous species
In a study on Aster amellus, the capacity was observed to decolorize the sulfonated azo dye Remazol Red. After the cultivation period, four non-toxic metabolites were identified; this indicated that the plant can be used for cleaning textile effluents ([68]). In another study on phytoremediation of dyes from textile wastewater, Tagetes patula, Aster amellus, Portulaca grandiflora and Gaillardia grandiflora were tested separately, finding that within 30 days they reduced the color value by 59, 50, 46 and 73%, respectively. Only a minor decrease in plant growth was observed, suggesting that these ornamental species could be an interesting solution for use on the ridges of constructed wetland for the treatment of dyes ([25]).
Tagetes patula and Mirabilis jalapa were tested in a pot experiments to evaluate their remediation capacity towards benzo[a]pyrene (B[a]P). The dry biomass of the two species increased at low B[a]P doses and then reduced with increasing concentrations. It also emerged that the tolerance to this pollutant was greater at the plant’s flowering and mature stages compared with the seedling stage. Significantly positive correlations were found between the B[a]P content of roots, stems, leaves and shoots to soil B[a]P concentrations ([122]).
For the treatment of PHCs-contaminated soil, Iris dichotoma and I. lactea were investigated in a pot culture experiment. These species were found to promote degradation of fractions of PHCs. I. lactea tolerated high concentration of PHCs (40.000 mg kg-1) and showed a good degradation rate of petroleum hydrocarbons. In contrast, I. dichotoma tolerated lower PHC concentrations, with a lower rate of total petroleum hydrocarbons (TPHs) degradation ([27]). Impatiens balsamina was also tested for petroleum remediation, finding that after a 4-month culture period in pot, the average TPHs degradation rate was up to 18.13-65.03%, greater than that (10.20-35.61%) of natural degradation in the control treatment ([20]).
In a pot-culture experiment to assess the TPHs-phytoremediation potential of 14 ornamental plants in petroleum-contaminated soil, it emerged that Gaillardia aristata, Echinacea purpurea, Festuca arundinacea and Medicago sativa were effective in reducing TPHs (and related compounds) in 10 mg kg-1 TPH-contaminated soil. Removal rates after 30 days were between 37.2 and 49.4%, (control only 12.9%). Removal rates of TPH composition were also significantly higher than controls, and Fourier transform infrared spectroscopy confirmed the presence of oil in the plant tissues ([80]).
Plants of Portulaca oleracea were able to remove fluoride from a 10 mg L-1 NaF solution, within 15 days by 73%; the higher fluoride concentrations showed lower removal rates ([67]). Canna indica was studied in a hydroponic system for testing its ability to remediate triazophos contamination, a harmful pesticide. After 21 days of exposure, a significant percentage of the substance was removed from the substrate ([29]). Among numerous other cases, Chrysanthemum morifolium can simultaneously absorb and purify benzene and formaldehyde to a large extent, while Aloe vera var. chinensis can absorb formaldehyde; Crassula portulacea is active towards benzene, and Dianthus chinensis towards sulfur dioxide ([75]).
Wild ornamentals with high ornamental value, fast growth and extensive root systems are, in general, a suitable solution, when deemed capable of degrading contaminants, due to their broad adaptability, widespread distribution and ease of cultivation ([28]).
Consociations
Soils are often polluted by different metals or organic compounds, so phytoremediation may require multiple plant species and ecotypes since most of the plants suited to this purpose show an aptitude to accumulate only one or a few pollutants.
A pot experiment was carried out with the aim of determining the phytoextraction potential of the hyperaccumulator Pteris vittata when co-planted with a woody tree (Morus alba or Broussonetia papyrifera) in soil contaminated with Cd, Pb, Zn, or As. The uptake of As was significantly increased when co-planted with Morus or Broussonetia (by 80.0% and 64.2% respectively). However, co-plantation did not have a promoting effect on the metal accumulation of both M. alba L. and B. papyrifera ([142]).
In the case a huge expanse of land, a consociation of grasses could be a good solution. Work by Maila et al. ([86]) demonstrated the potential of the grass species Brachiaria serrata and Eleusine corocana in decontaminating PAHs-contaminated soil. It was found that after a ten-week treatment the naphthalene concentration was undetectable in the “multispecies” vegetated soil compared to 96% removal efficiency in the monoplanted treatment and 63% in the control. For the same contaminants, ryegrass (Lolium perenne), white clover (Trifolium repens) and celery (Apium graveolens) were tested, finding that the remaining percentage of PAHs in mixtures was significantly lower than those in monocultures and non-planted soils ([90]). Another work proved that Brassica campestris showed low removal of PAHs, while Medicago sativa had the highest potential for remediation of phenanthrene and Trifolium repens for pyrene; but mixed cropping (rape with white clover or alfalfa, Medicago sativa) showed far better results than single cropping for the remediation of PAHs ([136]).
Concerning polychlorinated biphenyls (PCB), Terzaghi et al. ([125]) demonstrated that Festuca arundinacea cultivated by adding compost or in consociation with Cucurbita pepo ssp. pepo and Medicago sativa cultivated with Rhizobium spp. and mycorrhizal fungi reduced total PCB concentrations by about 20%, with a significant depletion in a high number of PCB congeners. In an in vitro experiment, Petunia grandiflora and Gaillardia grandiflora, when cultured together, showed a great effectiveness in degrading and removing a dye mixture from the substrate in 36 h, with results significantly higher than those detected from the cultivars in isolation ([132]).
Enhanced phytoremediation
It is worth noting that, for several tree species, the plant-fungi-bacterium system represents an important interactive balance for the implementation of the phytoremediation activity, as recently observed in hybrid poplar (Populus deltoides × P. nigra) and willow (Salix purpurea subsp. lambertiana - [50]). Eucalyptus camaldulensis also demonstrated increased effectiveness in extraction, uptake, and translocation of Cd when inoculated with arbuscular mycorrhiza fungi or plant growth promoting rhizobacteria ([95]). As an example for herbaceous species, Helianthus annuus inoculated with Bacillus safensis and/or Kocuria rosea was tested in soil with four levels of Ni concentrations (0, 150, 300, and 450 mg kg-1), finding that the highest Ni uptake was observed at Ni 300, when the sunflower seed was co-inoculated by B. safensis + K. rosea ([94]).
With regard to organic pollutants, endophyte-assisted phytoremediation of a site contaminated with Trichloroethylene (TCE) was studied using Populus deltoides × nigra inoculated with a strain of Enterobacter. The inoculated trees showed an increased growth and a reduced toxic effect compared to control, excreting 50% more chloride ions into the rhizosphere, a good signal of an increased TCE metabolism in planta. A significant decrease in the concentration of TCE and its derivatives from the tree-associated groundwater plume was also detected ([39]). With hybrid poplar clones associated to Arthrobacter strains, the possibility to rhizoremediate soils contaminated with the insecticide exachlorocyclohexane (HCH) isomers was demonstrated, stressing the importance of in situ pre-selection of the best candidate plants and bacteria strains ([9]). The shrub Cytisus striatus, also in association with microbial inoculants (Rhodococcus erythropolis and Sphingomonas sp.) showed an interesting activity on the dissipation of the HCH. HCH concentration in soil was reduced after plant growth and, more significantly, with inoculated plants ([7]).
Many studies assess the remediation of metal-polluted soil with the help of several agents, mainly synthetic organic chelates, but also natural organic compounds and inorganic products, that overcome limitations to phytoremediation due to low metal solubility and availability ([72]). Nevertheless, the high cost of these products and the possible toxic outflow into the environment have to be taken into account. Below, a few cases are mentioned as examples.
The application to soil of sodium dodecyl sulfate (SDS), ethylenediaminetriacetic acid (EDTA) and ethylenegluatarotriacetic acid (EGTA) to enhance Cd remediation was studied with Calendula officinalis. EDTA was observed to be toxic to the plants, while the addition of SDS and/or EGTA resulted in significantly increased plant biomass (p < 0.05). Almost all of the treatments containing SDS or/and EGTA led to an increase in the total Cd content in the plants ([79]). For enhancing the uptake and translocation of Cd, Cr, and Ni, two cultivars of Helianthus annuus were used in conjunction with EDTA and citric acid (CA) as chelators. EDTA at a concentration of 0.1 g kg-1 produced the best results for both cultivars, while the highest CA concentrations had a phytotoxic effect ([127]). In Althaea rosea, EDTA and tannic acid led to higher heavy metal removal of Cd, Ni, Pb and Cu from an artificially contaminated soil, with significant heavy metal accumulation in stems and leaves ([24]).
In view of a more environmentally friendly choice, less harmful products can be used. Amaranthus caudatus showed an increased capacity to uptake cadmium when solutions of tea saponin (extracted from camellia seeds) or EDTA were supplied to the soil, detecting TF >1, with better values for saponin ([23]). In a pot experiment with Helianthus annuus, the effects of culture in a soil contaminated with Cd and Zn and amended with swine manure, salicylic acid (SA), or potassium chloride (KCl) were assessed. The three amendments increased sunflower biomass, height, and flower diameter. Manure significantly decreased the bioaccumulation coefficient (BCF) of Cd and Zn, while KCl increased the BCF of Cd. Either swine manure and KCl increased Cd and Zn translocation from roots to aboveground parts, while swine manure and SA reduced the Cd/Zn ratios in flowers ([58]).
Within this wide frame, the development of transgenic plants with enhanced phytoremediation capacity is also a possible approach ([114]), but the general opposition of public opinion to the introduction of genetically modified plant species has to be carefully considered.
Conclusions
The use of ornamental (woody and flowering) plants for the phytoremediation of urban and periurban environments shows many positive aspects that have been highlighted in this work. The “multipurpose” function of these plants plays an important role in the environmental restoration and aesthetic enhancement, but the success of the phytoremediation strategy lies in the careful choice of species and/or genotypes matching the specific environments and pollutants.
From the review of the available literature it emerged that, trees in general, even if not classifiable as hyperaccumulators, display a greater potential for exploitation in phytoremediation compared to herbaceous species. This is simply due to the greater biomass growth potential and rooting system depth of woody species. On the other hand, herbaceous species are characterised by higher variability and plasticity, and offer the possibility of frequent replacements.
Among trees, Salicaceae are probably the most investigated species for phytoremediation purposes ([87]). Great interest is addressed to Salix spp., while poplars are now considered to be model species, comparable to Arabidopsis among herbaceous plants. Due to their adaptability to different environments, fast growth, ease of propagation and good performances when exposed to some pollutants, these species might possess some useful practical applications in phytoremediation, particularly in peri-urban areas. For urban environments, several other woody species are probably more suitable, being characterised by a higher ornamental value. Among flowering herbaceous plants, the possibility of choice is significantly wider; for instance, the Asteraceae family shows a wide range of interesting species ([98]) with sunflowers having been studied in depth and demonstrating a high capacity to remediate specific pollutants.
Several interesting species are yet to be explored, and special attention should be paid to the huge possibilities offered by plant consociation, including aspects related to modifications in the structure of the rhizosphera. Within this topic, possible associations between herbaceous plants, trees or between herbaceous and woody plants are practically infinite, allowing a perfect adhesion to the needs of each specific environment, and making phytoremediation an “aesthetic experience”, as proposed by Sleegers ([117]).
Finally, efforts are required to overcome problems related to the disposal of contaminated materials and how to limit the costs related to the exploitation of this technique.
In synthesis, phytoremediation could now be seen as part of a multifunctional process that creates a green infrastructure network defining evolving landscapes, not only in the countryside but also in urban environments.
Acknowledgements
I gratefully acknowledge Mrs. Catia Boggi and Mr. Gabriele Cencetti for their cooperation in the information retrieval and for the images provided. The manuscript was greatly improved by the comments provided by two anonymous reviewers.
References
CrossRef | Gscholar
Gscholar
Gscholar
Gscholar
CrossRef | Gscholar
Gscholar
Gscholar
Gscholar
Gscholar
CrossRef | Gscholar
Gscholar
Online | Gscholar
Gscholar
CrossRef | Gscholar
Gscholar
CrossRef | Gscholar
Authors’ Info
Authors’ Affiliation
Institute of Biosciences and Bioresources, National Research Council, v. Madonna del Piano 10, I-50019 Sesto Fiorentino, FI (Italy)
Corresponding author
Paper Info
Citation
Capuana M (2020). A review of the performance of woody and herbaceous ornamental plants for phytoremediation in urban areas. iForest 13: 139-151. - doi: 10.3832/ifor3242-013
Academic Editor
Werther Guidi Nissim
Paper history
Received: Sep 18, 2019
Accepted: Feb 07, 2020
First online: Apr 14, 2020
Publication Date: Apr 30, 2020
Publication Time: 2.23 months
Copyright Information
© SISEF - The Italian Society of Silviculture and Forest Ecology 2020
Open Access
This article is distributed under the terms of the Creative Commons Attribution-Non Commercial 4.0 International (https://creativecommons.org/licenses/by-nc/4.0/), which permits unrestricted use, distribution, and reproduction in any medium, provided you give appropriate credit to the original author(s) and the source, provide a link to the Creative Commons license, and indicate if changes were made.
Web Metrics
Breakdown by View Type
Article Usage
Total Article Views: 40898
(from publication date up to now)
Breakdown by View Type
HTML Page Views: 32656
Abstract Page Views: 3397
PDF Downloads: 4247
Citation/Reference Downloads: 21
XML Downloads: 577
Web Metrics
Days since publication: 1682
Overall contacts: 40898
Avg. contacts per week: 170.21
Article Citations
Article citations are based on data periodically collected from the Clarivate Web of Science web site
(last update: Feb 2023)
Total number of cites (since 2020): 13
Average cites per year: 3.25
Publication Metrics
by Dimensions ©
Articles citing this article
List of the papers citing this article based on CrossRef Cited-by.
Related Contents
iForest Similar Articles
Research Articles
Heavy metal (Zn, Pb, Cd) concentration in soil and moss (Pleurozium schreberii) in the Brynica district, southern Poland
vol. 4, pp. 176-180 (online: 11 August 2011)
Review Papers
Heavy metals and woody plants - biotechnologies for phytoremediation
vol. 4, pp. 7-15 (online: 27 January 2011)
Research Articles
Variation of major elements and heavy metals occurrence in hybrid aspen (Populus tremuloides Michx. × P. tremula L.) tree rings in marginal land
vol. 13, pp. 24-32 (online: 15 January 2020)
Review Papers
Monitoring the effects of air pollution on forest condition in Europe: is crown defoliation an adequate indicator?
vol. 3, pp. 86-88 (online: 15 July 2010)
Technical Reports
Air pollution regulations in Turkey and harmonization with the EU legislation
vol. 4, pp. 181-185 (online: 11 August 2011)
Editorials
COST Action FP0903: “Research, monitoring and modelling in the study of climate change and air pollution impacts on forest ecosystems”
vol. 4, pp. 160-161 (online: 11 August 2011)
Research Articles
Distribution and concentration of cadmium in root tissue of Populus alba determined by scanning electron microscopy and energy-dispersive x-ray microanalysis
vol. 1, pp. 96-103 (online: 20 May 2008)
Research Articles
Can species Cedrela fissilis Vell. be used in sites contaminated with toxic aluminum and cadmium metals?
vol. 14, pp. 508-516 (online: 11 November 2021)
Research Articles
Dust collection potential and air pollution tolerance indices in some young plant species in arid regions of Iran
vol. 12, pp. 558-564 (online: 17 December 2019)
Commentaries & Perspectives
Clean air policy under the UNECE Convention on long-range transboundary air pollution: how are monitoring results “translated” to policy action
vol. 2, pp. 49-50 (online: 21 January 2009)
iForest Database Search
Search By Author
Search By Keyword
Google Scholar Search
Citing Articles
Search By Author
Search By Keywords