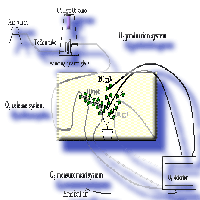
A new approach to ozone plant fumigation: the Web-O3-Fumigation. Isoprene response to a gradient of ozone stress in leaves of Quercus pubescens
iForest - Biogeosciences and Forestry, Volume 1, Issue 1, Pages 22-26 (2008)
doi: https://doi.org/10.3832/ifor0240-0010022
Published: Feb 28, 2008 - Copyright © 2008 SISEF
Research Articles
Abstract
The innovative Web-O3-Fumigation (WOF) is a suitable technique to study O3 effects in plants. Through WOF, a very high dose of O3 fumigation may be obtained at the level of selected leaves, while allowing a gradient of ozone stress in branches at different distance from branch directly fumigated with O3 diffusing from perforated Teflon tubes. We used WOF to study the impact of O3 on the emission of isoprene, a putatively powerful antioxidant, in leaves of Quercus pubescens. In our experiment we produced with WOF: i) approximately 300 ppb O3 on the leaves of the branch directly exposed to the pollutant; ii) approximately 190 ppb on the leaves of the branches distant less than 30 cm from the treated branch; iii) a summer ambient O3 concentration (100 ppb) on the leaves of the branches distant more than 30 cm from the branch directly fumigated. High O3 concentrations induced a long-lasting inhibition of photosynthesis and isoprene emission in leaves which were directly fumigated. However, isoprene emission was stimulated by intermediate O3 concentration 288 hours after the end of the treatment. Isoprene stimulation, and the activation of the related antioxidant mechanism, may therefore be dependent on the O3 concentration and may be different in plant canopies depending on their exposure to the pollutant.
Keywords
Ozone, Isoprene, Web-fumigation, Photosynthesis, Stomatal conductance
Introduction
Tropospheric ozone (O3) is an important phytotoxic air pollutant ([4], [10]). In summer, tropospheric ozone concentrations can reach peaks of 100 ppb. The rising O3 level in the atmosphere affects the growth rate of plants and induces visible foliar injury. The O3 negative effect is due to the reactive oxygen species (ROS) that is produced within the leaves ([3], [2]). The ROS can damage cellular membranes impairing cellular metabolic function ([13]). Plant responses to O3 are dependent on the uptake of O3 into the leaf and on the action of defensive mechanisms in plant tissues. A reduction of stomatal conductance may be regarded as a physical resistance mechanism to O3 ([7], [16]). However, plants also operate defensive mechanisms within plant tissue to detoxify O3 or repair injured tissue ([9]). Isoprene emission is one of the most important of such mechanisms in Mediterranean vegetation as demonstrated by Affek & Yakir ([1]) and Loreto et al. ([5]).
The simplest way to assess plant response to O3 is to grow plants inside enclosures into which O3 is then continually released in concentrations that enrich or simulate the daily variations of the pollutant. This method allows researchers to evaluate the effect of several concentrations of O3 (typically up to three times of the ambient concentration) as well as those of other gases or pollutants that can be added simultaneously ([11], [15]) The enclosure system in which plants must be maintained to allow O3 enrichment, may alter the atmospheric parameters and environment and usually it is difficult to determine which is the effect due to the O3 treatment and which is the contribution of the artefact growing conditions.
In a less disruptive approach, called the “zonal air pollution system” (ZAPS) ([17]), a series of pipes over the crop continuously releases O3 into the plant canopy at various rates in different plots. This method avoids some of the artificial conditions inside chambers but is more expensive. Another disadvantage is the low maximum enrichment achieved by this technique, because of the continuous mixing with ambient air.
In this work we used a new technique, hereby referred to as WEB-O3-fumigation, to fumigate the deciduous downy oak Q. pubescens with O3. This technique is an evolution of the ZAPS. It makes possible to steadily release the O3 target concentration at leaf level through perforations in Teflon tubes which are wrapped around one of the branches of a plant in correspondence to the target leaves. The technique also allowed to study the impact of an O3 exposure gradient in the same plant depending on the distance from the pollution source. Furthermore, this method allowed to operate the fumigation in completely field condition and therefore reducing or eliminating artefacts by enclosure systems.
This paper describes the Web-O3-fumigation system and presents data from a specific application of this technique to study the effect of different gradients of ozone stress on the isoprene emission of Q. pubescens leaves.
Materials and Methods
Plant material
Three year old Q. pubescens plants were grown in 10 L pots filled with commercial sandy soil. All plants were grown during spring and summer time outside under a typical Mediterranean ecosystem conditions characterized by dry and hot days. The plants were regularly irrigated and fertilized to avoid drought and nutritional stress.
Gas exchange measurements
The exchange of CO2 and H2O between leaf and air were measured with Li-6400 Portable Gas Exchange System (Li-COR, Lincoln, Nebraska, USA). The central part of a fully expanded, intact leaf was clamped in the Li 6400 gas-exchange cuvette holding a 6 cm2 area, and exposed to an artificial atmosphere reconstituting ambient air by mixing O2, N2 and CO2 (20%, 80% 0.037% respectively) but free of any contamination. A flow rate of 0.5 L min-1 was used. During all measurements, the cuvette was maintained at a temperature of 30 C and the leaf was illuminated with a light intensity of 1000 mmol m-2s-1. Simultaneously to CO2 and H2O gas exchange measurements, also isoprene emissions were detected by connecting on line the Li-6400 gas exchange cuvette to a portable gas chromatograph (Syntech GC 855) as described by Loreto & Velikova ([6]). Gas exchanges were measured before and after the ozone treatment in leaves of the branch directly exposed to the fumigation (high O3 concentration), in leaves of branches not directly hit by the O3 jet, but at distance < 30 cm from the fumigating tubes (intermediate O3 concentration), and in leaves far (distance lower than 30 cm) from the O3 treated branch (ambient O3 concentration). The parameters were analysed immediately after the end of the treatment (0h) and during the recovery (96, 144 and 288h). The experiment was carried out on five different plants and on each plant were exposed to O3 only three branches. The analyses were done on three different leaves for each treated branch. Each measurement was done at the same time of the day to minimize physiological and environmental changes. The analysis time-course (30 min) was sufficient for physiological parameter and the isoprene emission to reach a steady state.
Web-O3-Fumigation system
The Web-O3-Fumigation system was based on three different parts: the O3 production system, the O3 release system and the O3 measurement system (Fig. 1)
The O3 production system
Ozone was generated by flowing air, pushed by a diaphragm air pump through a winding quartz glass illuminated with an UV light source Helios Italquartz (Italquartz, Milan, Italy). The amount of ozone generated was regulated by changing the intensity of the UV light source and changing the air flow through the winding quartz glass allowing different levels of ozone enrichment of the ambient air (Fig. 1).
The O3 release system
Teflon tubes (internal diameter 4 mm), previously perforated with 0.2 mm holes in correspondence to the branch leaves, were wrapped around one of the major branches of the plant. This allowed leaves of that branch to be directly exposed to high ozone concentration (300 ppb), while other branches of the same plant were exposed to intermediate (190 ppb) or ambient (100 ppb) O3 concentrations depending on their distance from the fumigation holes (≤ 30 cm and > 30 cm, respectively). Plants were fumigated with ozone for three days, 8 h per day (from 9:00 to 17:00). To improve the control of O3 concentrations, a wind barrier was created. The rectangular open top wind barrier (3x4x3, 1.50 m high) was constructed at a distance of 2 m from the plant as a wooden frame covered with a low density fiber net allowing air circulation without significantly altering plant microclimate.
The O3 measurement system
The Photometric O3 analyzer (1008 Dasibi Environmental Corp., Glendale, California, USA) continuously monitored the O3 concentration in different parts of the plant and at different distance from the plant. Two sampling lines were installed on each plant branch (at the lower and at the upper part of the branches exposed to high, intermediate, and ambient O3) and two sampling lines were installed to control the ambient O3 concentration. All sampling air inlets were positioned at 5 cm from the O3 release point, corresponding to the average distance between leaves and O3 vents. Air from the sampling Teflon lines (internal diameter 4 mm, 2 m in length) was continuously drawn by the AC diaphragm pump contained in the Dasibi ozone detector at a flow rate of 1 litre per minute. The Dasibi ozone detector measured every 20 seconds the O3 concentration in the sample air, allowing sufficient time to purge the measurement system. Each of the 8 sampling lines were monitored for 15 min and four times during the 8h period of each day of the experiment. Switching between the different sampling lines was done by manually operating glass valves.
Weather station data
The climatic data were continuously monitored by a weather station installed 50 m away from the experimental site. The weather station measured air temperature, relative humidity and wind speed with an anemometer (SIAP, Bologna, Italy).
Statistics
The data presented are means from 5 different plants. Means were statistically separated by a Tukey’s test, and values significantly different at the 5% in respect to control level are identified by asterisks.
Results
The O3 fumigation was done from 15 to 17 July 2003 from 9:00 to 17:00. During fumigation, the weather was typically Mediterranean, characterized by absence of rain and clouds, and wind speed and air temperature reaching a maximum early afternoon while relative humidity was at its minimum (Fig. 2).
Fig. 2 - Diurnal course of wind speed (A), ambient temperature (B) and relative humidity (C) during the three days of the experiment (15-16-17 July 2003). The black line represents the mean S.E. (n=3).
As expected, the high solar irradiation coupled with anthropogenic and biogenic ozone precursors allowed an increase in photochemical reaction of O3 formation, typical of the summer period in the Mediterranean area. In fact, during the warmer hours the ambient ozone concentration reached peaks of 100 ppb (Fig. 3A).
Fig. 3 - Average of: ambient O3 concentration (A), O3 concentration sampled on parts of the plant branch distant ≤ 30 cm from the treated branch (B), O3 concentration sampled on parts of the plant branch distant less than 30 cm from the treated branch (C); the dashed line indicates the mean value of ozone during the fumigation (mean S.E; n=540: on each sampling line the Dasibi ozone detector has done 45 measurements in 15 min, for 4 times during 8h of treatment, and for 3 days of treatment).
As mentioned before, the wind speed varied during the day, ranging from 2 to 5 m/s (Fig. 2A). The moderate wind speed did not affected the O3 concentrations in our experimental system, also because of the windbreak system surrounding plants. Both, the high target O3 concentration (Fig. 3B) and the intermediate O3 concentration (Fig. 3C), monitored continuously in different parts of the O3 treated plant branches, were maintained constant. As expected, a more significant variation of the O3 concentration was observed in the branches exposed to intermediate ozone, mainly depending on wind speed and directions. Nevertheless, branches exposed to intermediate fumigation experienced an O3 dose higher than ambient through the entire period (Fig. 3C), as the average O3 concentration experienced during fumigation was 190 ppb.
Q. pubescens plants exposed to the three O3 concentrations did not show any visible injury few days after the treatment (data not shown). However, plants exposed to 300 ppb and 190 ppb of ozone concentration showed a reduction of photosynthesis and stomatal conductance after the treatment (Fig. 4A, B). Recovery of stomatal conductance was complete on leaves directly treated with ozone (300 ppb - Fig. 4A). On the contrary, there was no recovery of the photosynthetic rate. The intercellular CO2 concentration did not change following O3 exposure (Fig. 6), suggesting that photosynthesis reduction was caused by stomatal conductance reduction. The isoprene emission reduction followed the photosynthetic rate inhibition. There was no isoprene emission recovery after 288h (Fig. 5A).
Fig. 4 - Q. pubescens photosynthesis (A) and stomatal conductance (gs) before the ozone treatment (C= control), immediately (0h) and at 96, 144 and 288 hours after the end of the web-fumigation. (A) Leaves of the branch directly fumigated with ozone (300ppb), (B) leaves of the branch close (≤ 30 cm) to ozone source (190 ppb), (C) leaves of branch far (more than 30 cm) from ozone source (100ppb). Means S.E. (n see Material and Method) are shown. Two stars indicate for A and gs means significantly different (p < 0.05) from O3 control as assumed by Tukey’s test. One star indicates means for gs significantly different (p < 0.05) from O3 control as assumed by Tukey’s test.
Fig. 6 - Q. pubescens CO2 intercellular concentration (Ci) in leaves before ozone treatment (C= Control), immediately (0h) and at 96, 144 and 288 hours after the end of the web-fumigation. Leaves of the branch directly fumigated with ozone (300 ppb); leaves of the branch close (≤ 30 cm) to the ozone source (190 ppb), leaves of branch far (more than 30 cm) from ozone source (100 ppb). Means S.E. (n: see Material and Method) are shown.
Fig. 5 - Q. pubescens isoprene emission before the ozone treatment (C= Control), immediately (0h) and 96, 144 and 288 hours after the end of the web-fumigation. (A) Leaves of the branch directly fumigated with ozone (300ppb), (B) leaves of the branch closed (≤ 30 cm) to ozone source (190 ppb), (C) leaves of branch far (more than 30 cm) from ozone source (100ppb). Means S.E. (n see Material and Method) are shown. Two stars indicate means for isoprene emissions significantly different (p < 0.05) from O3 treatment by Tukey’s test.
Leaves exposed to the intermediate O3 concentration (average 190 ppb over the treatment, Fig. 4B, 5B) showed a total recovery of both photosynthetic rate and stomatal conductance 96h after the end of the ozone treatment. The isoprene emission showed a reduction of 30% immediately after the end of the ozone treatment, but the emission was stimulated by 30% after 288h (Fig. 4B). Leaves exposed to ambient ozone concentrations did not show significantly reduction of the observed parameters as shown for photosynthesis, stomatal conductance (Fig. 4C) and isoprene emission (Fig. 5C).
Discussion
Many studies focusing on ozone effects on plant physiology have been conducted using different techniques of ozone-fumigation. Field experiments where whole plants are fumigated on large structures, e.g., Open Top Chambers ([18]) have problems of consistency and homogeneity of the fumigation and do not allow insight on the effect of the pollutant on plant parts that are not directly exposed to the stress but may be somehow indirectly interested by the physiological responses of stressed plant structures. In addition, it is difficult to extrapolate the effect of ozone from the effect of the confined environment in which plants must be maintained to allow O3 enrichment. Laboratory experiments on whole plant ([12]) or single leaf ozone fumigation ([5]) are certainly more precise and have been efficient to understand physiological and biochemical changes induced by O3. However, laboratory measurements are even less transferable to field conditions and do not necessarily reflect the real response by outdoor plants. They also do not allow large scale measurements being limited temporally and spatially. For instance, it is not possible to carry out laboratory measurements with large plants such as forest plants.
The Web Fumigation is not a new technique but it was used instead of the FACE-system (Free Air CO2 Enrichment system) to allow CO2 fumigation on tall canopy trees ([14]). We have adapted this technique to make it suitable for the O3 fumigation. The Web-O3-Fumigation has several advantages that make this technique promising for ecological studies of gaseous pollutants. First, it allows field measurements on plants maintained in open air, therefore minimizing invasivity of enclosure systems that may alter atmospheric parameters and environmental conditions. This can be done also with the Free-Air-Enrichment technique (FACE for CO2, e.g., [8]), but we could demonstrate, with our experiment showing that O3 disappears at short distance from the fumigation source even in absence of wind, FACE techniques would be extremely difficult to use for highly reactive gases. Second, the Web-O3-fumigation allows to obtain gradients of O3 fumigation, in order to study the effect of ozone on plant structures of the same individual (thus genetically coherent) but subjected to different levels of stress, as it often occurs in nature for the exposure to acute sources of pollutants (e.g., in urban areas). We have shown in our experiment that branches of Q. pubescens not directly exposed to high O3, may suffer from O3-induced transient damage and may even stimulate isoprene synthesis, as previously observed for other terpenes ([5]). But we have also seen that in response to a heavy treatment (300 ppb), the physiological parameters of the same plant are more permanently reduced and the activation of terpenes synthesis is delayed or inhibited. Perhaps this means that additional mechanisms of response to stress are activated when the stress is acute and needs a more powerful antioxidant response for the plant to cope with it ([6]). Third, the Web-O3-technique makes it possible to study the effect of the same stress on different parts of large trees, such as young fully expanded leaves, or leaves of several years in the case of evergreen plants. Fourth, this technique allows to transfer the precision and replicability of O3 fumigation obtained in laboratory experiments to field conditions, thus expanding the possibility to efficiently study process-based mechanisms such as photosynthesis limitations or the activation of defence mechanisms in response to the stress.
Acknowledgements
We thank Violeta Velikova who helped with gas-exchange measurements. We thank Giuseppe Santarelli for providing weather data and Francesco Loreto for many helpful discussions and for the critical reading of the manuscript. This study has been supported by the European Commission (contract MC-RTN-CT-2003-504720, “ISONET”) and by the Ministero dell’Ambiente Project “Effetti degli stress ambientali con particolare riguardo all’ozono troposferico sulla vegetazione naturale ed in area Mediterranea” (OZONIT).
References
Gscholar
Gscholar
Gscholar
Gscholar
Gscholar
Gscholar
Authors’ Info
Authors’ Affiliation
Corresponding author
Paper Info
Citation
Pinelli P, Tricoli D (2008). A new approach to ozone plant fumigation: the Web-O3-Fumigation. Isoprene response to a gradient of ozone stress in leaves of Quercus pubescens. iForest 1: 22-26. - doi: 10.3832/ifor0240-0010022
Paper history
Received: Sep 10, 2004
Accepted: Dec 20, 2004
First online: Feb 28, 2008
Publication Date: Feb 28, 2008
Publication Time: 38.83 months
Copyright Information
© SISEF - The Italian Society of Silviculture and Forest Ecology 2008
Open Access
This article is distributed under the terms of the Creative Commons Attribution-Non Commercial 4.0 International (https://creativecommons.org/licenses/by-nc/4.0/), which permits unrestricted use, distribution, and reproduction in any medium, provided you give appropriate credit to the original author(s) and the source, provide a link to the Creative Commons license, and indicate if changes were made.
Web Metrics
Breakdown by View Type
Article Usage
Total Article Views: 52848
(from publication date up to now)
Breakdown by View Type
HTML Page Views: 44491
Abstract Page Views: 2390
PDF Downloads: 5081
Citation/Reference Downloads: 64
XML Downloads: 822
Web Metrics
Days since publication: 5994
Overall contacts: 52848
Avg. contacts per week: 61.72
Article Citations
Article citations are based on data periodically collected from the Clarivate Web of Science web site
(last update: Feb 2023)
Total number of cites (since 2008): 6
Average cites per year: 0.38
Publication Metrics
by Dimensions ©
Articles citing this article
List of the papers citing this article based on CrossRef Cited-by.
Related Contents
iForest Similar Articles
Research Articles
The use of branch enclosures to assess direct and indirect effects of elevated CO2 on photosynthesis, respiration and isoprene emission of Populus alba leaves
vol. 1, pp. 49-54 (online: 28 February 2008)
Research Articles
A comparison between stomatal ozone uptake and AOT40 of deciduous trees in Japan
vol. 4, pp. 128-135 (online: 01 June 2011)
Research Articles
Ozone fumigation effects on the morphology and biomass of Norway spruce (Picea abies L.) saplings
vol. 2, pp. 15-18 (online: 21 January 2009)
Research Articles
Photosynthesis of three evergreen broad-leaved tree species, Castanopsis sieboldii, Quercus glauca, and Q. myrsinaefolia, under elevated ozone
vol. 11, pp. 360-366 (online: 04 May 2018)
Research Articles
Drought tolerance in cork oak is associated with low leaf stomatal and hydraulic conductances
vol. 11, pp. 728-733 (online: 06 November 2018)
Research Articles
Prediction of ozone effects on net ecosystem production of Norway spruce forest
vol. 11, pp. 743-750 (online: 15 November 2018)
Research Articles
Light acclimation of leaf gas exchange in two Tunisian cork oak populations from contrasting environmental conditions
vol. 8, pp. 700-706 (online: 08 January 2015)
Research Articles
Developing stand transpiration model relating canopy conductance to stand sapwood area in a Korean pine plantation
vol. 14, pp. 186-194 (online: 14 April 2021)
Research Articles
Gas exchange characteristics of the hybrid Azadirachta indica × Melia azedarach
vol. 8, pp. 431-437 (online: 17 December 2014)
Research Articles
Role of photosynthesis and stomatal conductance on the long-term rising of intrinsic water use efficiency in dominant trees in three old-growth forests in Bosnia-Herzegovina and Montenegro
vol. 14, pp. 53-60 (online: 28 January 2021)
iForest Database Search
Search By Author
Search By Keyword
Google Scholar Search
Citing Articles
Search By Author
Search By Keywords
PubMed Search
Search By Author
Search By Keyword