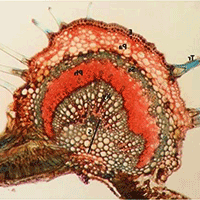
Evaluating anatomical and biochemical responses to cement dust pollution in Quercus castaneifolia C.A. Mey. and Zelkova carpinifolia (Pall.)
iForest - Biogeosciences and Forestry, Volume 18, Issue 3, Pages 102-108 (2025)
doi: https://doi.org/10.3832/ifor4574-018
Published: May 10, 2025 - Copyright © 2025 SISEF
Research Articles
Abstract
Understanding how plants respond to industrial pollutants is crucial for assessing environmental impacts and developing effective management strategies. This study focused on the structural and biochemical adaptations of Quercus castaneifolia and Zelkova carpinifolia in response to cement dust pollution, which poses a significant environmental risk and adversely affects plant growth. We investigated structural changes in leaves and analyzed key biochemical markers in trees growing near a cement factory in Neka city, Mazandaran province, Iran, to examine the defense mechanisms these species use against dust pollution. Our results demonstrated notable anatomical adaptations in both species, including increased druse crystals and sclerified parenchyma cells. Additionally, Q. castaneifolia leaves showed wall collapse of xylary vessel elements under dust exposure. Biochemically, we observed elevated phenylalanine ammonia-lyase (PAL) activity and higher concentrations of phenolic compounds and lignin in leaves from polluted sites. While Q. castaneifolia exhibited higher PAL activity, Z. carpinifolia showed greater levels of phenolics and lignin, alongside more pronounced anatomical changes. These findings highlight species-specific defense strategies and resilience to cement dust pollution. The study underscores the importance of integrating both anatomical and biochemical parameters in ecological assessments and suggests that environmental management strategies should account for species-specific responses to pollution.
Keywords
Cement Dust Pollution, Leaf Anatomy, Phenylalanine Ammonia-Lyase, Lignin, Quercus castaniefolia, Zelkova carpinifolia
Introduction
Cement factories have long been recognized as significant contributors to environmental pollution, releasing hazardous substances that threaten human health and the ecosystem ([10], [38]). The dust emitted by these industries contains elevated levels of heavy metals, including arsenic (As), cadmium (Cd), lead (Pb), mercury (Hg), thallium (Tl), aluminum (Al), beryllium (Be), chromium (Cr), copper (Cu), manganese (Mn), nickel (Ni), and zinc (Zn), all of which have been established as toxic by various environmental studies ([5], [7]). Beyond environmental contamination, the impact of cement dust extends to plant morphology and physiology, causing alterations in crucial parameters such as plant height, leaf number, area, and the fresh and dry weight of shoots and roots. Moreover, reductions in chlorophyll and carotenoid content and overall levels of plant sugars, proteins, and fats have been observed ([39]).
Despite the detrimental effects, particular plant species exhibit remarkable tolerance to cement dust contamination, attributed to genetic predisposition and concurrent biochemical and structural adaptations ([44], [12]). In response to pollutant toxicity, plants activate various enzymatic systems to mitigate or eliminate the damaging effects of pollutants ([11]). Phenylpropanoid metabolism is implicated in this response, particularly the role of the primary enzyme phenylalanine ammonia lyase (PAL) in the plant phenylpropanoid pathway. Elevated PAL enzyme activity and the production of phenolic compounds, such as lignin, are typical plant reactions to adverse environmental conditions, with phenol content as an indicator of plant stress severity ([6]).
With their expanded surface area and stomata, leaves are particularly susceptible to pollution. Stomata serve as key pathways for the penetration of pollutants into the intercellular and cellular spaces of leaves ([15]). Consequently, leaf anatomy is critical in discerning the effects of different contamination levels. Recent studies have emphasized the importance of anatomical modifications, mesophyll changes, and phenolic compound deposits in response to air pollution in various plant species ([19], [10]). The biochemical features of leaves, especially phenolic content, change in response to the level of air pollutants. Plants exposed to high pollution exhibit resistance and are effective bioindicators of air pollution ([9], [43]).
In light of previous investigations, which revealed elevated concentrations of heavy metals, such as Co, Cr, Cu, Cd, Mn, Ni, and Zn, in the vicinity of the Neka cement factory surpassing standard levels ([7]), the present study aims to unveil the structural and biochemical responses of two tree species, Zelkova carpinifolia (Pall.) and Quercus castaneifolia C.A. Mey., situated in proximity to the factory. This investigation was conducted in contrast to trees from a control site unaffected by the factory’s influence. The ecological and economic significance of Q. castaneifolia, a pivotal species within the Hyrcanian forests, and Z. carpinifolia, known for its high-quality wood and captivating canopy, underscores the critical need to comprehend their adaptive responses to cement dust pollution. These insights are not only vital for the preservation of these species but also have broader implications for biodiversity and ecological stability in Iranian forests ([41], [2]).
Materials and methods
Study area and sampling
The study focused on two distinct areas, classified based on pollution levels, as per the data provided by the Environmental Protection Organization of Mazandaran province. The polluted site, situated 2.5 km south of Neka, Iran (36° 39′ 5″ N, 53° 17′ 49″ E), was close to the Neka Cement Factory, while the control area was the Zarrin Abad Forest, located 11 km southeast of Sari, and 25 km away from the cement factory. The polluted site was approximately 500 m from the factory. Sampling was done in October 2021 from the upper half of the canopy in a southern direction. Three individual trees of each species were selected from the control and the polluted sites, and mature and uniform leaves were collected from each tree.
Soil physicochemical properties and heavy metal analysis
Soil samples from control and polluted sites were collected at 0-20 cm depth with four replicates each. The samples were dried, mixed thoroughly, and sieved before examination. Soil composition was assessed using the hydrometer technique. EC and pH were measured in soil-water mixtures. Samples were digested with a 2:1 mixture of nitric and perchloric acid. Heavy metalswere analyzed using ICP-MS, with calibration standards for accuracy. Results were reported in µg g-1 of dry soil weight.
Anatomical study
Transverse sections were prepared from the central portion of the mature leaves by free-hand sectioning (the section thickness typically ranged from 10 to 50 micrometers). Light microscopy was employed, and the photographs were taken using a Canon digital camera. Anatomical traits were measured by Digimizer software version 5.3.5 (MedCalc Software Ltd, Ostend, Belgium).
PAL activity assay
Leaf samples (300 mg) were homogenized in 6.5 mL Tris-HCl buffer at pH 8.8, supplemented with 15 mM of β-mercaptoethanol. The resulting extract underwent centrifugation at 12.000 rpm at 4 °C for 30 min, and the obtained supernatant was utilized for the enzymatic assay. A mixture containing 1 ml of the extraction buffer, 0.5 ml of 10 mM L-phenylalanine, 0.15 ml of supernatant, and 0.35 ml of double-distilled water was placed at 37 °C for 1 h. The reaction was terminated by adding 0.5 ml of 6 M HCl. The amount of trans-cinnamic acid formed was measured spectrophotometrically (Biochrom WPA Biowave II) at 290 nm. The enzyme activity was expressed in µmoles of trans-cinnamic acid per mg protein per hour ([45]).
Total phenolic content
The total phenolic content was determined using the Folin assay described by Singleton et al. ([42]). A reaction mixture comprising 1 ml of plant extracts, 0.5 ml of 50% FCR dissolved in 3 ml of deionized water, and 1 ml of 5% Na2CO3 solution was stored in the dark at room temperature for 2 h. The absorbance at 765 nm was measured spectrophotometrically (Biochrom WPA Biowave II), and the total phenolic content was expressed as mg of gallic acid equivalents per g fresh weight.
Total lignin content
The isolated cell wall material (5 mg) was subjected to a treatment involving a combination of 2.5 ml of 25% (w/w) acetyl bromide in HOAc and 0.1 ml of 70% HClO4 at 70 °C for one hour with gentle swirling every 10 min. After ice cooling, the mixture was transferred to a 25 ml volumetric flask containing 5 ml of 2M NaOH and 6 ml HOAc and filled up to 25 ml with acetic acid. Acetyl bromide soluble lignin was quantified using a known absorption coefficient (20.0 g-1 L cm-1) and absorbance at 280 nm ([16]).
Statistical analysis
Biochemical data were analyzed using one-way ANOVA, followed by the Duncan multiple range test, and anatomical data were analyzed using a t-test. The mean values, derived from nine replicates, were considered statistically significant at p<0.05. Statistical software SPSS®, version 21 (IBM Corporation, Armonk, NY, USA). was applied for statistical analysis, and Excel software version 2021 (Redmond, WA, USA) was used for diagrams.
Results
Soil physicochemical properties and heavy metal concentrations
Soil texture analysis revealed higher silt and clay content in the polluted area compared to the control area, with no significant pH difference. The electrical conductivity (EC) was higher in the control area than the contaminated area, but neither region exhibited saline soil conditions overall (Tab. 1). The contaminated soils exhibited elevated concentrations of cadmium (Cd), lead (Pb), zinc (Zn), and chromium (Cr) in relation to the unpolluted samples, indicating significant changes in soil characteristics and the accumulation of heavy metals attributed to pollution. (Tab. 1).
Tab. 1 - Physicochemical properties and heavy metal concentrations of the studied soils. Values are presented as mean ± standard deviation, with 4 replicates. Asterisks (*) indicate significant differences at the 5% level.
Category | Parameters | Control | Polluted |
---|---|---|---|
Physicochemical properties |
Sand % | 29.00 ± 1.41 | 30.75 ± 1.70 |
Silt % | 49.00 ± 1.80 | 55.00 ± 2.06* | |
Clay % | 22.00 ± 2.00* | 13.75 ± 1.00 | |
EC | 0.85 ± 0.03 | 1.05 ± 0.02* | |
pH | 7.50 ± 0.01 | 7.53 ± 0.02 | |
Heavy metal concentrations (µg g-1) |
Cd | 1.05 ± 0.05 | 2.25 ± 0.23* |
Pb | 35.20 ± 1.83 | 38.50 ± 1.20* | |
Cu | 28.22 ± 2.71 | 24.50 ± 1.58 | |
Zn | 50.25 ± 3.75 | 69.60 ± 1.91* | |
Ni | 42.35 ± 3.96 | 40.62 ± 2.86 | |
Cr | 74.62 ± 1.97 | 131.25 ± 2.79* |
Leaf anatomy and morphological changes
Quercus castaneifolia
In describing the leaf structure of Q. castaneifolia, it is important to note the presence of a bifacial mesophyll structure, with trichomes found on both the adaxial and abaxial surfaces of the leaf. Notably, the frequency of trichomes was higher on the lower leaf surface. The midrib exhibited a large stele resulting from merging vascular bundles, surrounded by a continuous ring of phloem fibers forming a sclerenchyma sheath. The amphipheloic Siphonostele nature of the stele was evident due to the presence of phloem both externally and internally to the xylem (Fig. 1).
Fig. 1 - Cross sections of the Quercus castaneifolia leaf midrib. (A): control site; (B): polluted site; (Tr): Trichome; (Ss): Sclerenchyma sheath; (Ph): Phloem; (Xy): Xylem; (S): Sclerenchyma. The white arrow in (B) indicates dark deposits and red arrow indicates cell with phenolic compound.
Comparative anatomy between polluted and control sites showed a significant increase in the upper cuticle thickness in trees from contaminated locations. However, no significant differences were observed in the thickness of the lower cuticle, upper and lower epidermis, or trichome number. Dark deposits and phenolic compound-containing cells were visible in the midrib of Q. castaneifolia leaves from polluted areas (Fig. 1B). The thickness of the palisade parenchymic tissue increased significantly in polluted area leaves, while spongy parenchyma and leaf blade thickness showed no significant differences between contaminated and control areas (Tab. 2). No significant differences were noted in the midrib thickness, midrib stele area, or thickness of the sclerenchyma sheath surrounding the midrib stele between control and polluted samples near Neka Cement Factory and Zarrin Abad Forest. However, a significant increase in druse crystals and structural deformities in xylary vessel elements was observed in Q. castaneifolia leaves exposed to cement pollution (Fig. 2, Tab. 2).
Tab. 2 - Leaf anatomical characteristics of Quercus castaneifolia from the cement-polluted and control sites. (LCT): Lower Cuticle Thickness; (UCT): Upper Cuticle Thickness; (LET): Lower Epiderm Thickness; (UET): Upper Epiderm Thickness; (SPT): Spongy Parenchyma Thickness; (PPT): Palisade Parenchyma Thickness; (LBT): Leaf Blade Thickness; (MSA): Midrib Stele Area; (MT): Midrib Thickness; (MSST): Midrib Sclerenchyma Sheath Thickness; (DCN): Druse Crystal Number; (TN): Trichome Number. (*): p <0.05.
Parameter | Polluted | Control | t-value | p-value |
---|---|---|---|---|
LCT (μm) | 0.055 ± 0.008 | 0.053 ± 0.005 | 0.521 | 0.617 |
UCT (μm) | 0.065 ± 0.007 | 0.052 ± 0.001 | 3.707 | 0.006* |
LET (μm) | 0.153 ± 0.019 | 0.172 ± 0.007 | -2.046 | 0.075 |
UET (μm) | 0.173 ± 0.011 | 0.178 ± 0.024 | -0.396 | 0.703 |
SPT (μm) | 0.644 ± 0.120 | 0.528 ± 0.064 | 1.907 | 0.093 |
PPT (μm) | 0.646 ± 0.151 | 0.377 ± 0.106 | 3.242 | 0.012* |
LBT (μm) | 1.549 ± 0.343 | 1.201 ± 0.075 | 2.214 | 0.058 |
MSA (μm) | 1.491 ± 0.182 | 1.295 ± 0.108 | 2.071 | 0.072 |
MT (μm) | 8.699 ± 0.688 | 9.080 ± 2.106 | -0.391 | 0.712 |
MSST (μm) | 0.452 ± 0.078 | 0.487 ± 0.066 | -0.767 | 0.465 |
DCN | 10.800 ± 1.483 | 5.600 ± 1.140 | 6.215 | <0.001* |
TN | 3.600 ± 1.342 | 3.800 ± 0.837 | -0.283 | 0.784 |
Fig. 2 - Cross sections of the Quercus castaneifolia midrib in polluted site. (A): black arrow indicates cell with druse crystal; (B): black arrow indicates deformation of xylary elements.
Zelkova carpinifolia
Leaf anatomy of Z. carpinifolia resembled that of Q. castaneifolia, the main difference being the absence of inner phloem in the Z. carpinifolia leaf midrib, resulting in an ectophloic siphonostele (Fig. 3). In polluted sites, the lower cuticle thickness showed no significant difference, but the lower epidermis thickness increased significantly, along with thickening of inner periclinal walls of lower epidermal cells (Fig. 4B). The upper cuticle thickness significantly increased in leaves exposed to cement contamination, while the upper epidermis thickness showed no significant difference (Tab. 3). Trichome number exhibited no significant difference between polluted and control areas in Z. carpinifolia leaves. Notably, an increase was observed in palisade and spongy parenchyma thickness, leaf blade thickness, and leaf midrib thickness of leaves from polluted areas. The midrib stele area increased significantly in leaves from contaminated sites, while the thickness of the sclerenchyma sheath around the stele remained unchanged (Tab. 3). An increase in the sclerified parenchyma in the leaf midrib was observed near the Neka cement factory compared to Zarrin Abad Forest (Fig. 4A).
Fig. 3 - Cross sections of the Zelkova carpinifolia leaf midrib. (A): control site; (B): polluted site; (Tr): Trichome; (Pa): parenchyma; (Ss): Sclerenchyma sheath; (Ph): Phloem; (Xy): Xylem; (S): Sclerenchyma.
Fig. 4 - Cross sections of the Zelkova carpinifolia midrib in the polluted site. (A): black arrow indicates sclerified parenchyma; (B): black arrow indicates thickening of periclinal walls of epidermal cells.
Tab. 3 - Leaf anatomical characteristics of Zelkova carpinifolia from cement-polluted site and the control site. (LCT): Lower Cuticle Thickness; (UCT): Upper Cuticle Thickness; (LET): Lower Epiderm Thickness; (UET): Upper Epiderm Thickness; (SPT): Spongy Parenchyma Thickness; (PPT): Palisade Parenchyma Thickness; (LBT): Leaf Blade Thickness; (MSA): Midrib Stele Area; (MT): Midrib Thickness; (MSST): Midrib Sclerenchyma Sheath Thickness; (SPN): Sclerified parenchyma Number; (TN): Trichome Number. (*): p <0.05.
Parameter | Polluted | Control | t-value | p-value |
---|---|---|---|---|
LCT (μm) | 0.068 ± 0.014 | 0.068 ± 0.005 | 0.030 | 0.978 |
UCT (μm) | 0.081 ± 0.0057 | 0.060 ± 0.005 | 4.980 | 0.001* |
LET (μm) | 0.224 ± 0.027 | 0.179 ± 0.009 | 3.403 | 0.019* |
UET (μm) | 0.253 ± 0.028 | 0.235 ± 0.011 | 1.290 | 0.233 |
SPT (μm) | 0.688 ± 0.085 | 0.559 ± 0.050 | 2.918 | 0.019* |
PPT (μm) | 1.041 ± 0.118 | 0.726 ± 0.139 | 3.850 | 0.005* |
LBT (μm) | 2.353 ± 0.162 | 1.577 ± 0.080 | 9.539 | <0.001* |
MSA (μm) | 2.679 ± 0.572 | 1.953 ± 0.173 | 2.714 | 0.045* |
MT (μm) | 7.370 ± 1.286 | 5.622 ± 0.774 | 2.603 | 0.031* |
MSST (μm) | 0.519 ± 0.097 | 0.519 ± 0.054 | 0.004 | 0.997 |
SPN | 33.400 ± 4.219 | 7.400 ± 2.302 | 12.096 | <0.001* |
TN | 6.200 ± 1.789 | 4.800 ± 0.837 | 1.585 | 0.152 |
Biochemical responses to pollution
PAL activity significantly increased in the leaves of both species, Q. castaneifolia and Z. carpinifolia, from polluted sites, with higher levels in Q. castaneifolia leaves (Fig. 5). The total phenolic content was remarkably higher in leaves collected from contaminated sites for both species (Fig. 5). Conversely, lignin content increased only in Z. carpinifolia leaves from contaminated sites, with no significant difference in Q. castaneifolia leaves between polluted and control sites (Fig. 5).
Fig. 5 - PAL activity and total phenolic and lignin content in leaves of Q. castaneifolia and Z. carpinifolia in the polluted and control areas. Mean value ± standard deviation is shown (n = 6). Different letters indicate significant differences between the means (ANOVA and Duncan test, p< 0.05).
Discussion
Our investigation at the Neka cement factory revealed clear evidence of environmental pollution induced by cement dust and noxious emissions, corroborating prior research findings ([7]). The levels of heavy metals in the soil of the contaminated area were notably higher than in the control region. Furthermore, the contaminated soil exhibited a higher proportion of clay and silt than the control. These differences in soil composition can significantly influence heavy metal concentrations, as soils with higher clay content are more effective at absorbing and retaining heavy metals ([8]). These findings suggest that cement dust deposition significantly altered soil properties and contributed to heavy metal accumulation, likely affecting plant physiology. Such alterations in soil composition play a critical role in inducing stress responses in Q. castaneifolia and Z. carpinifolia, which were evident in both leaf anatomical changes and biochemical adaptations.
The deposition of cement dust particles on leaf surfaces, a naturally occurring phenomenon, is influenced by various factors such as phyllotaxy, leaf geometry, structure, and features of the epidermis and cuticle ([37], [38]). This study specifically focused on assessing the impact of pollution on the cuticle, the primary barrier against toxicant uptake, revealing a significant increase in the thickness of the upper cuticle in both Q. castaneifolia and Z. carpinifolia under polluted conditions. Notably, our study found no significant difference in the thickness of the lower cuticle between control and polluted areas for both species. This observation underscores the significance of the upper cuticle as the primary entry point for pollutants, particularly during nighttime when stomata are closed. The variability in the quantity and composition of the leaf cuticle, influenced by factors such as species, age, and environmental conditions, is known to impact contaminant permeability ([1]). Our results align with previous studies, such as those on Olea europaea leaves, where a thick cuticle acted as a barrier against contaminant penetration ([36]), and Platanus orientalis leaves, which exhibited a thinner cuticle in urban and polluted areas, potentially contributing to increased transpiration ([31]).
The examination of the epidermal layers indicated an increase in the upper cuticle thickness of both Q. castaneifolia and Z. carpinifolia in response to cement dust pollution. Additionally, the lower leaf epidermis thickness increased significantly in Z. carpinifolia, with thicker periclinal walls of the lower epidermal cells observed in polluted sites acting as an effective barrier to pollutant entry. This aligns with studies on Norway spruce and Brachiaria decumbens ([32], [17]). Surprisingly, the abundance of trichomes, known for their role in pollution response, did not exhibit significant changes in our study.
Concerning parenchyma thickness, Z. carpinifolia demonstrated a significant increase in palisade and spongy parenchyma thickness, while Q. castaneifolia exhibited a notable increase exclusively in palisade parenchyma. These observations align with prior studies establishing a positive correlation between palisade parenchyma thickness and resistance to urban environmental pollution, including air pollution ([9], [21], [29]). Furthermore, alterations in midrib thickness and stele area were evident in Z. carpinifolia, suggesting an adaptive mechanism to enhance water transfer. The observed augmentation in stele area implies an increase in tracheary elements, facilitating faster water transfer by reducing ion density ([3]). In a similar context, Rao et al. ([33]) noted an increase in the number of secondary xylem elements in Dalbergia sissoo under air pollution stress, accompanied by a reduction in the lumen diameter of xylem. Conversely, Q. castaneifolia leaves exhibited collapsed xylary vessel elements, indicating potential tissue damage and susceptibility to pollution-induced stress. This aligns with findings by Krzeslowska et al. ([24]) in Tilia cordata Miller growing on mining sludge, where internal tissues in the root apex displayed collapse, and abnormal vascular elements were observed. The phenomenon of cell degeneration and deformation is postulated to be affected by the disruptive effects of heavy metals on hormonal balance ([35]).
Observations of crystal formation and sclerification in both species underscored the plants’ response to severe stress conditions. Calcium oxalate crystal formation and sclerification are recognized mechanisms for regulating calcium levels and detoxifying heavy metals ([13], [20]). These adaptations were particularly prominent in Z. carpinifolia, demonstrating its ability to cope with pollution-induced stress. Sclerification, a recognized plant response to severe stress situations, has been documented in various species. For instance, Batool et al. ([4]) reported an association between enhanced sclerification in the root and stem of Cyperus alopecuroides and increased tolerance to Cd and Ni in an industrially polluted site.
In comparison, Q. castaneifolia exhibited fewer anatomical changes, aligning with the known resilience of Quercus species to pollution ([28], [26]). The observed increase in PAL activity in both species near the cement factory suggests a non-specific indicator of the plant’s adaptive response to pollution, consistent with studies on Pinus eldarica, Prosopis glandulosa, and others ([27], [18]). PAL is a key enzyme in secondary phenylpropanoid metabolism, and the levels of phenylpropanoids are regulated by environmental factors ([22]). PAL activity is positively correlated with various biotic and abiotic stresses. Enhanced activity of PPO (Polyphenol Oxidase), PAL, and CAD (Cinnamyl Alcohol Dehydrogenase) enzymes was reported in needles of Pinus eldarica and Pinus nigra exposed to heavy metal pollution from the Sarcheshmeh Copper Complex ([27]). Also, the highest increment in PAL activity was observed in leaves of Prosopis glandulosa treated with cadmium and copper ([18]). This consistency in the modulation of PAL activity levels, reflecting the presence of cement dust pollution, underscores the non-specific nature of this enzymatic response.
The substantial augmentation in total phenolic content in Q. castaneifolia and the exceptional accumulation in Z. carpinifolia under the influence of cement dust pollution indicate the activation of the phenylpropanoid pathway as a response to environmental stress ([40]). Phenolic compounds, including phenolic acids, flavonoids, coumarins, tannins, lignans, and lignin, constitute pivotal components of plant secondary metabolites synthesized through the phenylpropanoid pathway ([25]). The activation of the phenylpropanoid pathway in plants exposed to diverse stressors, such as salinity, drought, temperature variations, air pollution, and radiation, is well-established in the literature ([40]). Metal toxicity, as evidenced by increased total phenols and several polyphenols in Zea mays under such conditions, further supports the role of phenolic compounds as a response to stress ([23]). The significant increase in lignin content observed in Z. carpinifolia, as opposed to Q. castaneifolia, underscores distinct responses to pollution-induced stress within plant species. This finding aligns with the broader consensus established in prior studies on species-specific plant adaptations to varying environmental conditions, as documented by Förster et al. ([14]) and Rezapour et al. ([34]). While the typical plant response to stress involves enhanced PAL activity accompanied by an increase in both total phenolic compounds and lignin content ([30]), the reduced lignin accumulation in Q. castaneifolia leaves, despite the elevated PAL activity, suggests a deviation in the phenylpropanoid pathway. This implies a shift toward producing different phenolic compounds rather than lignin in response to cement dust pollution.
Conclusions
Our findings indicate substantial quantitative and qualitative alterations in the leaf structures of both Q. castaneifolia and Z. carpinifolia species when exposed to cement dust pollution. The higher phenylalanine ammonia lyase activity, along with increased concentrations of phenolic compounds and total lignin in both species under such pollution, confirms the key role of phenolic compounds in fostering an adaptive plant response to the adverse impacts of pollution stress. Notably, Z. carpinifolia exhibits more pronounced anatomical changes and higher concentrations of total phenolics and total lignin than Q. castaneifolia, while Q. castaneifolia demonstrates higher PAL activity. These findings highlight the species-specific mechanisms for adapting to environmental stresses and highlight the tolerance of both species to cement pollution stress. Overall, this study contributes to our understanding of plant responses to industrial pollution, emphasizing the need for species-specific considerations in ecological assessments.
Conflict of interest
The authors declare that there is no conflict of interest.
Acknowledgements
We thank Mr. Abdolreza Yadollahpour for his valuable contribution to the field work, and we would like to express our gratitude to the Narenj Espadana Soil Science Laboratory in Isfahan for their cooperation in analyzing the soil samples.
Authors’ contribution
SK carried out research, conceptualization, and supervision of the work. SK and ZH were responsible for conducting field and laboratory measurements. SK executed the statistical analysis and wrote the first draft of the manuscript. AR contributed to the conceptualization and performed writing, review, and editing of the manuscript. All authors have read and agreed to the published version of the manuscript.
References
Gscholar
Gscholar
CrossRef | Gscholar
Gscholar
CrossRef | Gscholar
Authors’ Info
Authors’ Affiliation
Zeynab Hosseinzadeh
Department of Plant Sciences, University of Mazandaran, Babolsar (Iran)
Corresponding author
Paper Info
Citation
Kelij S, Hosseinzadeh Z, Rezapour A (2025). Evaluating anatomical and biochemical responses to cement dust pollution in Quercus castaneifolia C.A. Mey. and Zelkova carpinifolia (Pall.). iForest 18: 102-108. - doi: 10.3832/ifor4574-018
Academic Editor
Michele Colangelo
Paper history
Received: Feb 03, 2024
Accepted: Jan 25, 2025
First online: May 10, 2025
Publication Date: Jun 30, 2025
Publication Time: 3.50 months
Copyright Information
© SISEF - The Italian Society of Silviculture and Forest Ecology 2025
Open Access
This article is distributed under the terms of the Creative Commons Attribution-Non Commercial 4.0 International (https://creativecommons.org/licenses/by-nc/4.0/), which permits unrestricted use, distribution, and reproduction in any medium, provided you give appropriate credit to the original author(s) and the source, provide a link to the Creative Commons license, and indicate if changes were made.
Web Metrics
Breakdown by View Type
Article Usage
Total Article Views: 1232
(from publication date up to now)
Breakdown by View Type
HTML Page Views: 279
Abstract Page Views: 442
PDF Downloads: 412
Citation/Reference Downloads: 0
XML Downloads: 99
Web Metrics
Days since publication: 48
Overall contacts: 1232
Avg. contacts per week: 179.67
Article Citations
Article citations are based on data periodically collected from the Clarivate Web of Science web site
(last update: Mar 2025)
(No citations were found up to date. Please come back later)
Publication Metrics
by Dimensions ©
Articles citing this article
List of the papers citing this article based on CrossRef Cited-by.
Related Contents
iForest Similar Articles
Research Articles
Dust collection potential and air pollution tolerance indices in some young plant species in arid regions of Iran
vol. 12, pp. 558-564 (online: 17 December 2019)
Research Articles
Effect of dust accumulation on Quercus cerris L. leaves in the Ezer forest, Lebanon
vol. 15, pp. 322-330 (online: 23 August 2022)
Research Articles
Analysis of dust exposure during chainsaw forest operations
vol. 10, pp. 341-347 (online: 23 February 2017)
Research Articles
Links between phenology and ecophysiology in a European beech forest
vol. 8, pp. 438-447 (online: 15 December 2014)
Research Articles
Effect of drought stress on some growth, morphological, physiological, and biochemical parameters of two different populations of Quercus brantii
vol. 11, pp. 212-220 (online: 01 March 2018)
Research Articles
Bioaccumulation of long-term atmospheric heavy metal pollution within the Carpathian arch: monumental trees and their leaves memoir
vol. 17, pp. 370-377 (online: 27 November 2024)
Research Articles
Relationships between leaf physiognomy and sensitivity of photosynthetic processes to freezing for subtropical evergreen woody plants
vol. 12, pp. 551-557 (online: 17 December 2019)
Research Articles
Adjustment of photosynthetic carbon assimilation to higher growth irradiance in three-year-old seedlings of two Tunisian provenances of Cork Oak (Quercus suber L.)
vol. 10, pp. 618-624 (online: 17 May 2017)
Review Papers
Monitoring the effects of air pollution on forest condition in Europe: is crown defoliation an adequate indicator?
vol. 3, pp. 86-88 (online: 15 July 2010)
Technical Reports
Air pollution regulations in Turkey and harmonization with the EU legislation
vol. 4, pp. 181-185 (online: 11 August 2011)
iForest Database Search
Search By Author
Search By Keyword
Google Scholar Search
Citing Articles
Search By Author
Search By Keywords
PubMed Search
Search By Author
Search By Keyword