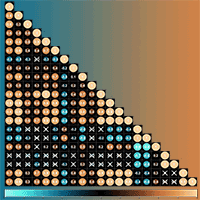
Seedling quality and short-term field performance of three Amazonian forest species as affected by site conditions
iForest - Biogeosciences and Forestry, Volume 17, Issue 2, Pages 80-89 (2024)
doi: https://doi.org/10.3832/ifor4317-016
Published: Mar 21, 2024 - Copyright © 2024 SISEF
Research Articles
Abstract
High quality seedlings are essential for the successful establishment of forest stands. Seedling quality can be assessed by the morphological attributes (e.g., height, diameter, dry mass, Dickson’s quality index) measured in the nursery phase. The defining, producing, and handling of seedlings can be based on specific characteristics suited to a site, as proposed by the Target Plant Concept. However, a target plant concept is an important research gap for Amazonian tree species. Here, we explore the associations between morphological attributes of seedlings in the nursery and determine the combined effect of the seedling quality attributes and site preparation methods on one-year field performance to define a range for nondestructive morphological attributes that can be associated with successful establishment for Amazonian commercial forest species - Bertholletia excelsa, Dipteryx odorata and Tachigali vulgaris. We measured morphological attributes in the nursery and analyzed the correlations to detect better predictors of destructive attributes. Then, we related these initial morphological attributes to survival and growth one year after planting in two site conditions: manual holing preparation and mechanical site preparation (subsoiling plus harrowing). We represent growth using different metrics: one-year size, absolute and relative growth rates in height and root collar diameter. Site conditions were assessed by soil physical properties. For all species, root collar diameter was a good predictor of destructive attributes that evaluated seedling quality, such as Dickson’s quality index. Site preparation methods resulted in different site qualities. Mechanical site preparation improved the total porosity and reduced the bulk density and resistance to penetration. Survival was not affected by initial attributes or site preparation methods. The initial attributes were poor predictors of field growth for D. odorata and T. vulgaris. The field performance of B. excelsa seedlings was affected by initial attributes, site, and the combination of both. The definition of a range for operational attributes according to site conditions is true only for B. excelsa. These results are important to help fill research gaps related to technical procedures to establish large-scale reforestation projects using Amazonian tree species.
Keywords
Plant Target Concept, Morphological Attributes, Mechanical Site Preparation, Subsoiling, Harrowing, Growth Rates
Introduction
Choosing species for plantations in a species-rich tropical forest is a complex task ([7]). Species for industrial plantations must attend to the production purpose (commercial value), to be available for planting (availability of seed or seedlings) and to grow well on the available sites ([32]). Bertholletia excelsa (Brazil nut tree), Dipteryx odorata (cumaru) and Tachigali vulgaris (tachi-branco) are Amazonian forest species with timber and/or non-timber value and with potential for restoration efforts. B. excelsa (Lecythidaceae) and D. odorata (Fabaceae) are considered priority native species for silviculture in the Amazon biome ([41]). T. vulgaris (Fabaceae) is a species with potential for energy purposes ([33]) and restoration programs due to its high growth rates, N-fixing and high litter production, and quick ground cover ([12]).
The limited supply of high-quality native seedlings and low seedling adaptation to stressful site conditions are obstacles for regeneration success and productivity ([18]). Seedling quality is defined by attributes that reach maximum survival and growth after planting in the field ([15], [16]). Seedling quality can be assessed by the morphological (e.g., height, root collar diameter, and dry mass) and physiological (e.g., chlorophyll and nutrient concentrations) attributes of the seedlings produced in the nursery, and morphological attributes are more commonly used, especially due to easier, faster, and cheaper measurements ([17]). In the nursery, attributes that are easier to measure, such as height (H), root collar diameter (RCD) and height/diameter ratio (H/D), can predict more complex morphological attributes. For example, RCD has been a strong predictor of root dry mass ([5]) and Dickson’s quality index (DQI - [2]).
Initial morphological attributes can impact field performance such as survival and growth ([15], [16]). RCD has been considered a good predictor of field performance ([23], [5], [26], [15], [30], [46]). Most of the results in the literature show a positive relationship between RCD and growth ([16]). However, the relationships can be dependent on the species. Tsakaldimi et al. ([46]) evaluated the survival after two years of five species planted in the Mediterranean. Some morphological attributes (such as RCD) had strong relationships with field survival, but the relationships were not significant for all species. For one species, survival was not related to any initial attribute, and the value range of morphological attributes considered optimum for survival varied between all species.
The effects of morphological attributes of seedling quality on field performance can be site specific. Small seedlings (RCD < 4 mm) had low survival in the dry season only when planted in thin soils, while in deeper soils, no seedling size effect was observed ([30]). Similarly, high-quality seedlings (with larger size and better nutritional status) of Quercus ilex only reached higher survival than low-quality seedlings when planted in mechanical subsoiling in comparison with manual holing ([35]). Thus, seedling selection in the nursery should be based on site requirements. The target plant concept is an effective framework for defining, producing, and handling seedlings based on specific characteristics of a site, where target plants are defined based on the conditions of the planting site, and this quality results in success ([9]). Therefore, the definition of seedling quality for plantations should be oriented by the effects of species vs. site interactions on field performance.
At Amazonia, the planting areas have historic pastures and intense management for agricultural cultivation with subsequent abandonment ([21]). In intensively managed areas, soil compaction can result in less aeration and low availability of water and nutrients, in addition to physical resistance to the growth of the root system of seedlings ([4]), reducing the survival and growth of forest species. The phase of site preparation is critical to improve the production and economic potential of forest or plantation land ([38]). The adoption of mechanized soil preparation (e.g., subsoiling) can reduce the physical limitation to root growth and allow greater acquisition of water and nutrients for seedling development ([35], [28]). In Brazil, 76% of areas were regenerated per activity using mechanized methods for site preparation ([38]). However, as in Brazil, exotic species (Eucalyptus and Pinus genera) dominate 95% of the 9.9 million hectares of planted forests ([20]), and mechanization for these species is more developed, while for native species, the use of mechanization in soil preparation is limited ([3], [28]).
In our study, we evaluated associations between morphological attributes measured in the nursery for three native Amazon species and the relationship of these attributes with one-year field performance at sites with manual (manual holing) and mechanical (subsoiling plus harrowing) preparation. Specifically, we sought to answer the following questions: (i) are nondestructive attributes (H, RCD and H/D) strong predictors of biomass in the nursery for all species? (ii) Are nondestructive attributes strong predictors of performance in the field? Do the relationships depend on the planting site and are species-specific? (iii) It is possible to define a range for nondestructive morphological attributes that can be associated with successful establishment for Amazonian commercial forest species? Our hypotheses are as follows: (i) root collar diameter is the best predictor of biomass attributes in the nursery for all species; (ii) root collar diameter is a good predictor of survival and growth after one year, and the relationships are stronger for slow-growing species (B. excelsa and D. odorata) and for the most limiting site (manual holing); and (iii) it is possible to indicate a value range of morphological attributes to help define a target plant for Amazonian commercial forest species.
Material and methods
Species selection, production and selection of seedlings in the nursery
The selection of species used in this study - Bertholletia excelsa Bonpl., Dipteryx odorata (Aubl.) Willd. and Tachigali vulgaris L.G. Silva & H.C. Lima - was based on the socioeconomic value, existence of plantations for commercial purposes with these species in the region, and different growth rates (or successional group). The seedlings of species were produced using polybags (common use in the region) with two volumes: 675 cm³ (13.5 × 8 cm, filled) and 1.200 cm³ (15.5 × 10 cm, filled), containing organic black colored soil (regionally known as “terra-preta”) and clay enriched with nutrients. The seedlings were cultivated in the nursery for a maximum of one year. The seeds of D. odorata and T. vulgaris were planted directly in the container, while the seeds of B. excelsa were sown in a germinator containing sawdust and after 30 days were transferred to the containers. The seedlings were kept under 50% shade and were irrigated twice a day with an overhead irrigation system. Thirty days before planting, the seedlings were subjected to full-sun acclimation to field conditions.
As our objective was to test the effect of seedling size on field performance at the end of the nursery phase, we used the size variation produced with the containers to group the seedlings of each species into four size classes according to the variation in the RCD. This grouping was based on the RCD because this non-destructive attribute is a good predictor of the total dry mass and the size of the root system ([5]). For B. excelsa and D. odorata, the initial seedling diameter classes were 1: 3 ≤ RCD < 4 mm; 2: 4 ≤ RCD < 5 mm; 3: 5 ≤ RCD < 6 mm; and 4: 6 ≤ RCD < 7 mm. For T. vulgaris, the initial seedling diameter classes were 1: 2.5 ≤ RCD < 3.5 mm; 2: 3.5 ≤ RCD < 4.5 mm; 3: 4.5 ≤ RCD < 5.5 mm; and 4: 5.5 ≤ RCD < 6.5 mm. In general, the first two classes were dominant in the lower containers (675 cm³), and the last two classes were dominant in the higher containers (1.200 cm³). The details about size per container are shown in Tab. S2 (Supplementary material). The initial grouping in diameter classes was necessary to implement a similar number of seedlings per size in the field.
Nursery phase measurements
To determine the seedling quality, we measured a set of morphological attributes in a sample of 40 seedlings (ten of each initial seedling diameter class) from each species (N = 120). We measured the total height (H), root collar diameter (RCD), height / diameter ratio (H/D), leaf number (LN), leaf area or leaf size (LS), total leaf area (TLA), specific leaf area (SLA), leaf dry mass (LDM), stem dry mass (SDM), shoot dry mass (LDM+SDM), root dry mass (RDM), total dry mass (TDM), root length (RL), root diameter (RD), and fine root number (FR). The shoot and root systems were separated. The number of leaves was counted, and the leaf size and total leaf area of each plant were measured using a leaf area meter (CI-202®, CID, Inc. Camas, WA, USA). The fresh mass was dried at 65 °C for 48 hours. For B. excelsa, the seeds were not considered part of the dry mass. SLA was calculated as the ratio between fresh leaf area and oven-dried mass ([36]). For compound leaves, we considered the sum of the area and mass of all the leaflets of a leaf, but the rachis was not included in the dry leaf mass. Root diameter and root length were measured with a digital caliper and a graduated ruler, respectively. Fine roots were considered roots with diameters less than 2 mm. The biomass fraction was assessed by leaf mass fraction (LMF: LDM/TDM), leaf area fraction (LAF: TLA/TDM), stem mass fraction (SMF: SDM/TDM), root mass fraction (RMF: RDM/TDM), shoot/root ratio (R/S), and shoot/height ratio (S/H). Dickson’s quality index (DQI) was calculated as follows (eqn. 1):
where h is the height (in cm), dcoll is the root collar diameter (in mm), while DMshoot, DMroot, and DMtot are the shoot, root and total dry mass (in g), respectively.
To discard possible physiological differences between the seedlings, we measured the chlorophyll content (SPAD index), which is also an indicator of nitrogen content, and the chlorophyll a fluorescence through maximum quantum yield of primary PSII photochemistry (Fv/Fm), a dynamic parameter indicative of stress. Twenty seedlings of each species were measured (five per diameter class). The SPAD index was measured in three different regions of each leaf, avoiding the veins, using a portable chlorophyll meter (SPAD model 502, Minolta, Osaka, Japan). Chlorophyll a fluorescence was determined between 8:00 and 9:00 hours using a portable fluorimeter (PEA, MK2 9600, Hansatech, Norfolk, UK). The measurements were made in two leaves per plant. Leaves were kept in the dark for 30 min before measurements. Later, leaves were exposed to a pulse of saturating light intensity of 3.000 µmol m-2 s-1 (650 nm) for one second. The JIP test was used to determine the Fv/Fm ratio ([44]).
Field experiment
The field experiment was conducted in an area of 0.55 hectares at the experimental farm at the Federal University of Amazonas (02° 38′ 57″ S and 60° 03′ 11″ W), Manaus, Amazonas, Brazil. The climate of the region is Af (tropical without dry season according Köppen climate classification system - [1]). The mean annual rainfall is 2.407 mm, with a wet season from November to May ([45]). In the past, the study area was used for agriculture cultivation, and before the installation of the experiment, the area was dominated by grasses. The predominant soil is a clayey Ferralsol ([6]), acid (pHH20 = 4.63), with medium concentrations of organic matter (22.2 mg kg-1), high values of Fe2+ (99.9 mg kg-1) and Al3+ (179.46 mg kg-1) and very low concentrations of phosphorus (1.86 mg kg-1).
Experimental design and site preparation
The field experiment was arranged in a split-plot design with two site preparation methods (mechanical site preparation, MSP; and manual holing preparation, MHP) and seedling size (according to the classes of root collar diameter) of three forest species in three randomized blocks (Fig. 1). Each block of 30 × 42 m was divided into two plots of 15 × 42 m according to the site preparation methods (MSP = subsoiling + harrowing; and MHP = only manual pit opening). In each plot, each species was allocated separately, and twenty-four seedlings of each species were distributed in four planting lines (one for diameter class) in a spacing of 3 × 2 m. As our objective was to test the effect of seedling size on field performance (and not the effect of the container size), the container was only used to achieve this variation, not being considered an experimental factor in the field and in the analyses. In each block, the distribution of the species subplots, site preparation methods and diameter classes were performed randomly. A total of 726 seedlings were planted, 432 in a useful plot and 294 as borders. Only seedlings of B. excelsa and D.odorata were used as borders.
Fig. 1 - (A) Arrangement of blocks in the area, (B) site preparation methods in each plot and (C) arrangement of planting lines, with useful plants in the root collar diameter class (RCD) and border lines (black) of one experimental block.
Mechanical site preparation was performed six months before planting (October 2018). The subsoiling was performed only in the planting row (minimum tillage) at an average depth of 40 cm using a tire tractor (Massey Ferguson model 4283) equipped with a subsoil plow (with two straight adjustable rods measuring 70 cm placed side by side). Harrowing was also realized in the planting line to break the remaining clods after subsoiling. Manual holing and acidity correction were realized four months before planting (December 2018). Manual holing of 30 × 30 × 30 cm was performed in both site preparation methods to control the soil volume for acidity correction. For correction of soil acidity, 100 g of dolomitic limestone was applied per pit. Planting was realized in April 2019. Each planting hole was fertilized with the application of 46 g of P2O5, 11.6 g N, 12 g K2O and 10 g FTEBR12 (7.1% Ca; 5.7% S; 1.8% B; 0.8% Cu; 2% Mn; 0.1% Mo and 9% Zn). Fertilizers were applied in the form of circles around each plant. Thirty and sixty days after planting, the same dosages of nitrogen and potassium were applied in each pit. Stand tending operations (weed and ant control and toppling prevention) were realized during the first year of the establishment. Seedlings were propped up with stakes and twine when necessary due to high winds in the experimental area, especially during the wet-dry season transition. A fipronil insecticide was applied with a thermonebulizer to eliminate leaf-cutting ants, as necessary. Weed control was conducted every two months using a brushcutter.
Field measurements - site quality assessment and seedling performance
As both sites did not show differences in soil fertility and texture (see Tab. S1 in Supplementary Material), we accessed site qualities through soil physics. Soil moisture, bulk density, total porosity, and soil resistance to penetration were analyzed in the planting lines every 10 cm of depth until 40 cm. Undisturbed soil samples were collected in May 2019 (one month after planting and during the wet season) using 90 cm3 volumetric rings. Two points were sampled per plot (site preparation method) per block. Soil bulk density, particle density and total porosity were determined according Donagema et al. ([11]). Soil bulk density was determined by the ratio between the soil dry weight and volume of the ring. Total porosity (Pt) was calculated by the equation [Pt = (1 - bulk density / particle density)]. Particle density was calculated by the formula (a/50 - b), where a is the dry weight, 50 is the volume of volumetric flask, and b is the volume of alcohol used in the titration. Soil moisture was determined by the gravimetric method ([14]). Soil resistance to penetration was obtained using an impact penetrometer (Sondaterra model PI-60). We used weight release heights of 30 cm and 50 cm for the mechanical and manual preparation sites, respectively. An electronic spreadsheet provided by the penetrometer manufacturer was used to calculate the soil resistance to penetration.
The survival of each species in the treatments was obtained by counting live and dead seedlings after one year. The total height and root collar diameter were measured on all plants every two months during the first year of establishment (from April 2019 until April 2020). We used different metrics to represent growth. Despite the final values in the field being important for industrial plantations, the use of growth rates (absolute and relative) provides an understanding of growth dynamics post planting. The relative growth rate expresses growth in terms of a rate of increase in size per unit of size, providing more equitable comparisons than an absolute growth rate ([19]), especially when different sizes of seedlings are used. The absolute growth rates (AGR) and relative growth rates (RGR) were calculated as AGRX = (X2 - X1) / (T2 - T1) and RGRX = [ln(X2) - ln(X1)] / (T2 - T1), respectively ([19]). where X1 is the height or root collar diameter at planting, X2 is the height or root collar diameter one year after planting and (T2 - T1) is the interval between measurements (one year).
Statistical analyses
To assess the interspecific variation in the attributes in the nursery, the coefficient of variation (CV) was calculated by the ratio between the standard deviation and mean multiplied by 100%. We generated Pearson’s correlation matrices to analyze the relationships between the morphological attributes of seedling quality measured in the nursery. To analyze the effect of site preparation on soil attributes (soil moisture, bulk density, total porosity, and soil resistance to penetration) as a function of depth, we performed a two-way ANOVA. For survival, we performed generalized linear mixed models (GLMMs) to analyze whether the effect of initial root collar diameter on frequency data (1 = alive plants, 0 = dead plants) depends on the site quality (categoric fixed factor). As we utilize the individual as repetition, we added the block as an aleatory factor due to the possible spatial autocorrelation. We used the “glmmTMB” package and binomial family distribution. We also generated Pearson’s correlation matrices to explore the relationships between initial morphological attributes and growth in the field using different metrics. Further, we used GLMMs to analyze whether the effect of initial morphological attributes on growth metrics (continuous fixed factors) depends on the site quality (categoric fixed factor). As we also utilize the individual as repetition, we added the block as an aleatory factor. Prior, for regressions with RGRs, the variables were natural logarithmically (ln) transformed to linear relationships. In addition, the relationships between H and RCD against RGRs using the original values are shown in Fig. S1 (Supplementary material). All analyses were performed using R software version 4.2.2 ([37]).
Results
Variation and associations between morphological attributes of seedlings in the nursery
The biomass attributes (LDM, SDM, RDM, TDM) and DQI had the highest coefficient of variation values, 59.8% and 53.5%, respectively. RCD (23.7%) had less variation than H (33.7%). SLA had the lowest variation for all species (average of 6.1%). D. odorata showed high CV values for the highest number of attributes (10 from 21 attributes), while T. vulgaris had the lowest number of attributes with high CV values (4 from 21 attributes). The seed mass of B. excelsa varied by 42.7%. The two physiological parameters had low CV values, 3.9% for the SPAD index and 8.3% for Fv/Fm. The absolute values for the morphological and physiological attributes per diameter class are shown in Tab. S3 (Supplementary material).
H and RCD were good predictors of biomass in the nursery phase, while H/D and leaf number were poor predictors of morphological attributes, especially for T. vulgaris (Fig. 2). For all species, H and RCD were good predictors of belowground biomass, with strong correlations with root diameter and root dry mass. RCD had a stronger correlation with root dry mass and DQI than height and H/D. Among the root attributes, root diameter had more significant correlations with other attributes, especially with biomass. The DQI had strong correlations with dry mass (leaves, stem, roots and total) and shoot/height ratio. Associations among morphological attributes were more similar between B. excelsa and D. odorata. For both species, the RMF and R/S were related to a few attributes. For T. vulgaris, H/D and FR had few significant correlations with other variables. For B. excelsa, seed mass had high correlation values (r > 0.80) with RCD, SDM, TDM and DQI (see Tab. S4 in Supplementary Material).
Fig. 2 - Correlations between morphological attributes measured in the forest nursery for Bertholletia excelsa, Dipteryx odorata and Tachigali vulgaris (n = 40). Notes: height (H, cm), root collar diameter (RCD, mm), height/diameter ratio (H/D), leaf number (LN), total leaf area (TLA, cm²), leaf size (LS, cm²), specific leaf area (SLA, cm² g-1), root length (RL, cm), root diameter (RD, mm), fine root number (FR), leaf dry mass (LDM, g), stem dry mass (SDM, g), root dry mass (RDM, g), total dry mass (TDM, g), leaf mass fraction (LMF, g g-1), leaf area fraction (LAF, cm² g-1), stem mass fraction (SMF, g g-1), root mass fraction (RMF, g g-1), root/shoot ratio (R/S, g g-1), shoot/height ratio (S/H, g cm-1), and Dickson’s quality index (DQI). Non-significant correlations (P ≥ 0.05) are indicated by the symbol X.
Changes in soil physical properties as dependent on site quality
The site preparation method affected soil physical properties, and this effect was dependent on the depth for soil moisture, bulk density, and total porosity (Fig. 3). Soil moisture (SM) varied by an average of 27.3 to 29.9% (Fig. 3A). For the superficial soil (0 to 10 cm), SM was higher (+9.6%) in MHP than in MSP. However, at the two final depths, the MSP had higher SM than the MHP, while for the 10 to 20 cm depth, there were no differences between sites. The values of bulk density (BD) varied from 1.12 g cm³ for MHP to 0.92 g cm³ for MSP (Fig. 3B). The MHP had higher values (+29%) than MSP, especially until 30 cm depth, while for the last layer, there were no differences between sites. An opposite response was observed for total porosity (TP - Fig. 3C), where the MSP (62.20%) had higher values for TP than the MHP (53.19%), especially until 30 cm depth. The site preparation method affected resistance to penetration (RP) regardless of depth, MHP (2.67 MPa) had almost three times higher RP than MSP (0.91 Mpa - Fig. 3D).
Fig. 3 - Soil physical properties (mean ± standard error) according to site quality. MHP manual holing preparation (MHP) and mechanical site preparation (MSP) (n = 3). (*): P < 0.05; (**): P < 0.01; (***): P < 0.001; (n.s.): not significant (P ≥ 0.05). Capital letters indicate significant differences for the individual effects, and small letters indicate significant differences for the interaction effect.
Performance in the field
There was low mortality one year after planting (5.3%). There was no significant effect of diameter class and site quality (Tab. S5 in Supplementary Material). Despite not having a significant effect, D. odorata had low values of survival (16.7%) for RCD 1 (3 to 4 mm) in MSP in one block. B. excelsa showed the highest values of survival among the species. The nondestructive attributes (H, RCD, and H/D) were measured in the field at planting time and correlated with performance one year after planting (Fig. 4). When disregarding the site, these initial attributes were poor predictors of plant growth, especially for T. vulgaris (Fig. 4C). D. odorata had a higher number of significant but weak relationships, while B. excelsa showed the opposite. Analyzing different metrics for growth, initial attributes had negative correlations with RGRs, while the correlations with AGRs and one-year size were positive. In general, the final values (i.e., values one year after planting) of H and RCD and H/D did not correlate with the initial values.
Fig. 4 - Relationship between initial attributes versus final attributes and absolute growth rates (AGR) and relative growth rates (RGR) in height and root collar diameter one year after planting of Bertholletia excelsa, Dipteryx odorata and Tachigali vulgaris. Nonsignificant correlations (P ≥ 0.05) are marked with X.
For example, H/D did not have strong correlations in the nursery (Fig. 2) and in the field (Fig. 4), and we evaluated the relationships between initial attributes and field growth as dependent on site only for H and RCD (Tab. 1). Such AGRs were strongly related to one-year values, and we analyzed the relationships only for final values and RGRs (Fig. 4). We considered the values in the field because the size is important in industrial plantings, especially for timber and biomass production.
Tab. 1 - Summary of GLMM for effects of initial root collar diameter (RCD) and height (H), site quality (SQ) and interaction effects on relative growth rates root collar diameter (RGR-rcd) and on height (RGR-h) and final values in height and root collar diameter after one year of three native Amazonian species. (*): P < 0.05; (**): P < 0.01; (***): P < 0.001; (ns): not significant (P ≥ 0.05).
Factors | B. excelsa | D. odorata | T. vulgaris | |||||||||
---|---|---|---|---|---|---|---|---|---|---|---|---|
RGR-rcd | RGR-h | RCD 1-y | H 1-y | RGR-rcd | RGR-h | RCD 1-y | H 1-y | RGR-rcd | RGR-h | RCD 1-y | H 1-y | |
RCD | ** | *** | * | *** | ns | ns | *** | ** | ns | ns | ns | ns |
SQ | *** | * | *** | ** | ns | ns | ns | ns | ns | ns | ns | ns |
RCD × SQ | *** | * | *** | ** | ns | ns | ns | ns | ns | ns | ns | ns |
R² | 0.09 | 0.17 | 0.33 | 0.34 | 0.05 | 0.03 | 0.16 | 0.13 | 0.06 | 0.07 | 0.15 | 0.19 |
H | * | *** | * | *** | ns | ns | *** | *** | ns | * | ns | * |
SQ | *** | ** | *** | * | ns | ns | ns | ns | ns | ns | ns | ns |
H × SQ | *** | * | *** | * | ns | ns | ns | ns | ns | ns | ns | ns |
R² | 0.08 | 0.27 | 0.31 | 0.34 | 0.03 | 0.04 | 0.15 | 0.15 | 0.05 | 0.08 | 0.16 | 0.21 |
When the site was included as a factor, H and RCD became better predictors for one-year size than for RGRs, especially for B. excelsa and D. odorata (Tab. 1). However, the initial attributes and site quality better explained the growth of B. excelsa. H and RCD were related to RGRs only for B. excelsa, and the relationships were stronger for RGR-h than for RGR-rcd (higher R²). For T. vulgaris, the initial attributes did not predict the one-year size and RGRs well.
Site quality (SQ) only influenced B. excelsa growth (Tab. 1). The SQ affected the relationships between initial attributes and growth, and the responses differed between growth metrics (Fig. 5). Overall, the RGR decreased, and the one-year size increased with the increase in the initial attributes. The RGR-h decreased with increasing H and RCD of planted seedlings, and the strongest relationships (highest slopes) were observed for MHP (Fig. 5B, Fig. 5D). In MHP, the increase in initial attributes also resulted in a reduction in RGR-rcd, while for MSP, the higher size of planted seedlings resulted in an increase in RGR-rcd (Fig. 5A, Fig. 5C). Although the relationships between initial attributes and one-year size were positive, for MSP, they were strongest. For each 1 mm of initial RCD, there was an increase of 5.5 mm in one-year RCD and 20.4 cm in one-year H (Fig. 5E, Fig. 5F). In MSP for each 1 cm of initial H, there was an increase of 0.39 mm in one-year RCD and 1.49 cm in one-year H (Fig. 5G, Fig. 5H). The effect of initial attributes on one-year size was on average 2.6 times higher for MSP than for MHP.
Fig. 5 - Relationship between initial root collar diameter (RCD) and height (H) and relative growth rates in height (RGR-h) and root collar diameter (RGR-rcd) (A, B, C, D) and absolute values of H and RCD (E, F, G, H) one year after planting according to site quality: MHP manual holing preparation (MHP) and mechanical site preparation (MSP) of Bertholletia excelsa. For relationships with RGRs, the variables were natural logarithmic (ln values). Slope values are shown for each site quality.
Discussion
Our results confirmed the hypothesis that nondestructive attributes are strong predictors of seedling biomass in the nursery, though some relationships between seedling quality attributes were species dependent. Our hypothesis that nondestructive attributes (H and RCD) are strong predictors of performance in the field was partially rejected because the relationships were dependent on three factors: species, growth metrics, and site quality. Finally, the definition of a range for nondestructive morphological attributes that can be associated with successful establishment for Amazonian commercial forest species cannot be expanded to all species and site conditions. In the next sections, we will discuss the major points that have led to these findings.
Nondestructive attributes are strong predictors of seedling biomass in the nursery
Despite the low variation in H and RCD, the biomass attributes and DQI had high CV values. This decoupling is important for the selection of better attributes to guide seedling selection in the nursery and to avoid the oversimplification of seedling selection. Despite the higher occurrence of significant than non-significant correlations between the seedling quality attributes, some correlations were species dependent (Fig. 2). Differences in the strength and significance of correlations among species were more associated with attributes of the quality of the root system (root length and number of fine roots), specific leaf area, leaf number, and biomass partition (RMF and R/S). DQI was not correlated with a few attributes, such as RMF and R/S for B. excelsa and D. odorata and SLA for T. vulgaris. DQI has been considered a better parameter to evaluate seedling quality by evaluating the biomass distribution ([13]) and impact field performance ([46], [22]). Here, the DQI was well related to most of the other attributes, especially non-destructive attributes such as H and RCD. One operational measure needs to be simple, rapid, cheap, reliable, nondestructive, quantitative, and diagnostic ([17]), and H and RCD were good predictors of DQI. However, due to the stronger relationships of RCD with RDM and DQI (Fig. 2), RCD is a good and robust parameter for seedling selection of B. excelsa, D. odorata and T. vulgaris in the nursery, as corroborated by other studies ([5], [2]). The definition of a good parameter by association with other important and non-operational parameters (such as destructive parameters) can be decisive for improving seedling quality. Differences in field performance can be associated with different physiological (demonstrated by chlorophyll fluorescence and nutritional status) vigor ([40]). In our study, we discarded this possibility due to equal physiological status between size classes at planting time.
Effects of seedling size on short-term field performance as dependent on species and site conditions
Our hypothesis that nondestructive attributes (H and RCD) are strong predictors of performance in the field was partially rejected. Most likely due to the high number of plants alive after one year, the initial attributes did not affect survival. Survival depends, in part, on the ability of seedlings to cope with environmentally stressful conditions that can occur after planting ([15]). The reduction in stressor factors, such as weed control, can contribute to the high survival of species ([23]). Here, the high survival can be associated with the study area not having strong stressors such as high levels of soil compaction and water limitation (Fig. 4) and by stand tending operations realized during the first year that must attenuate the stressful conditions. Initial morphological attributes are important for survival when environmental stress occurs ([22]).
Overall, height and root collar diameter were poor predictors of field growth, and the significance and direction of the correlations were dependent on growth metrics (Fig. 4). Positive relationships between initial attributes and size at field were expected due to the increase in size over time, and these positive significant relationships are more common in the literature ([23], [26], [22]). Positive relationships between initial H and RCD and AGRs were also reported ([8]). However, the RGRs had negative correlations with initial morphological attributes (Fig. 4); thus, the small seedlings grew more than the larger seedlings. These negative relationships can be associated with the growth metric because the increase in the same unit of size has a greater impact on small seedlings than on large seedlings. However, we expected that larger seedlings had an advantage in the field, mainly due to the larger size of the root system ([30]), but the lower growth of larger seedlings can be related to the physiological stress associated with a higher biomass ratio between leaves and roots, especially for fast-growing species. Small seedlings, generally produced under root restriction conditions, can undergo a process of acclimation and develop tolerance mechanisms that improve field performance ([25]). In addition, we do not consider the accumulation of biomass as a growth indicator. Significant relationships between initial attributes (RCD, specifically) and field performance were observed only for biomass ([5]). Here, such as B. excelsa and T. vulgaris allocating a considerable amount of biomass in branches, which is not captured by height and diameter measurements, the relationships with biomass growth can be stronger.
Mechanical site preparation improved the soil physical properties, reducing soil bulk density and penetration resistance and increasing total porosity (Fig. 4), but the site qualities had poor effects on seedling growth, where the site quality affected only the growth of B. excelsa (Tab. 1). This effect can be explained by the non-existence of strongly limiting conditions in the planting area. Clay subsoils (above 30 cm depth) are frequently limited by high bulk density (1.5 - 1.6 g cm-3 - [31]), a condition not exhibited even by the MHP. The MHP showed restriction to root growth only for the 0-20 cm layers, where values of penetration resistance (approximately 2.5 MPa) are considered restrictive ([4]). In addition, soils with a higher content of clay (see Tab. S1 in Supplementary Material), such as the study area, have a better distribution of micro- and macropores and greater water retention capacity ([4]).
Although some studies have demonstrated that mechanical soil preparation improves the growth of species ([34], [3], [35]), positive beneficial effects on growth are not always observed even with improvements in physical soil proprieties ([10]). The performance after mechanical site preparation is dependent on tree species and site ([27]). For two Amazon species, positive effects on mechanization (harrowing in total area) occurred at different times: after one year for fast-growing species (Ochroma lagopus) and after two years for slow-growing species (Jacaranda copaia - [3]). The authors associated this response to the higher abundance of fine roots for O. lagopus, which can contribute to the higher absorption of water and nutrients, especially in soils with higher aeration. However, such as here the slow-growing species (B. excelsa) was affected by site quality. It is possible that slow growth resulted in higher time under favorable MSP conditions due differences in physical soil occurred especially until 30 cm depth. For example, soil rupture is more effective on the soil surface ([39]), and deeper subsoiling could have a greater effect on species growth. For Eucalyptus, for example, a minimum of 50 cm subsoiling is recommended ([42]). In addition, the positive responses to mechanical preparation can be detectable only three years after planting and increase during the following years ([34]).
Site quality affected the relationships between initial attributes (H and RCD) and growth only for B. excelsa (Fig. 5). The initial attributes explained better one-year size (33%) than RGRs (15%) and showed opposite responses (Tab. 1). The negative and strongest relationships between H and RCD and RGRs for MHP can be explained by the smallest seedlings having advantages due to nursery acclimation (seedlings produced in smaller containers), especially when planted in more limiting sites. For RGR-rcd, in MSP, the relationships with initial attributes were positive, and in MHP, the relationships were negative. The higher porosity and lower bulk density, especially in superficial layers (Fig. 4), must have favored root growth of larger seedlings in MSP. In non-limiting conditions, large seedlings can have advantages in field growth ([24]). The stronger relationships with RGR-h than RGR-rcd can be associated with higher variation in RGR-d than RGR-h for similar values of initial attributes (see Fig. S1 in Supplementary Material). Although the RCD was a better predictor in the nursery phase, in the field, the H and RCD were equally important (R² values), at least for B. excelsa. However, changes in one unit of root collar diameter impacted more final values than changes in height, especially in MSP (Fig. 5). For example, another study also found a stronger relationship between initial attributes and growth for sites of better quality (deeper soils - [22]). Here, the larger seedlings at planting reached the highest values of H and RCD after one year, especially in MSP. At this site, the growth rates were more similar, and the largest seedlings at planting were also the largest seedlings after one year, which did not occur at the MHP site due to the more variable growth rates.
Can we define a range for operational attributes that can be associated with successful establishment for Amazonian commercial forest species? Implications for enhancing reforestation efforts in Amazonia
The definition of a range for operational attributes cannot be generalized to all species. Despite weak relationships between seedling quality attributes and growth for D. odorata and T. vulgaris, seedlings approximately 3 mm in root collar diameter had good survival and growth at the two site qualities, corroborating the recommendation of a minimum diameter of 3 mm for transplanting seedlings of most native forest species ([43]). On the other hand, for B. excelsa, the decision about seedling quality depends on the site, as suggested by the target plant concept. Larger seedlings (more than 5 mm of root collar diameter and 40 cm of height) when planted on sites with better soil physical properties (mechanical site preparation) achieve better performance after one year. This seedling size was reached in larger containers (Tab. S2 in Supplementary material). In the long term, this higher productivity can imply a minor rotation cycle.
However, the production of high-quality native seedlings does not always result in higher economic returns, as reported by nursery producers of the Amazonas state, when B. excelsa is one of the species more in demand for food-producing plantations ([29]). For this reason, establishing the range of values for initial attributes to improve field performance in a variety of site conditions (as proposed by the target plant concept) is essential to reduce losses, increase productivity and avoid unnecessary costs. Finally, in accordance with the global goals under the Paris Agreement to mitigate the effects of climate change, the Brazilian government has committed to reforestation 12 million ha of deforested areas by 2030. However, a set of research gaps related to technical procedures to establish large-scale reforestation projects using native species may impair these efforts. Specifically, we do not have full information based on science to determine the best phenotype of seedlings and management practices to cultivate most species ([41]). Thus, we hope that the main findings of this study may contribute to a recent initiative of Brazilian researchers and institutions to create a Research and Development Program in silviculture of native tree species accelerating and scaling up productive and protective plantations, especially in deforested areas in Amazonia.
Conclusions
In the nursery phase, the root collar diameter was the best predictor of seedling quality of three commercial species of Amazonian forest trees. However, this parameter did not predict performance in the field for D. odorata and T. vulgaris. Only B. excelsa was affected by the site quality and seedling size. The definition of a range for operational attributes according to site conditions is true only for B. excelsa. For T. vulgaris and D. odorata, a root collar diameter minimum of 3 mm is suggested for good performance in the field independent of site quality. For B. excelsa, the seedling size for planting must consider the site quality for productivity gains.
Acknowledgments
We are grateful to the Forest Nursery and Experimental Farm from the Federal University of Amazonas for support for seedling production and field experiments. We are grateful to the companies Agropecuária Aruanã and Empresa Brasileira de Pesquisa Agropecuária for their support in the experiment. We thank Dr. Roberval Lima, Dr. Afrânio Neves Junior, and Dr. Raimundo da Silva Júnior for the orientations for experimental installation and data collection. We are grateful to Laboratório Temático de Solos e Plantas and Instituto Nacional de Pesquisas da Amazônia for supporting the soil analyses. We acknowledge the Laboratório de Silvicultura team, Bruno Costa and José Soares for support in experimental installation and data collection.
Zilza Thayane Matos Guimarães thanks Fundação de Amparo à Pesquisa do Estado do Amazonas for granting the scholarship (Process no. 062.00973/2013) and for field finance support (Resolução N. 003/2019, N. 006/2020, and N. 008/2021 - POSGRAD). Debora Coelho da Silva thanks Fundação de Amparo à Pesquisa do Estado do Amazonas for granting the scholarship (Process no. 01.02.016301.001624/2021-10-FAPEAM). Marciel José Ferreira acknowledges the research productivity Grant - PQ provided by CNPq (Process no. 309870/2020-8). We also grateful to Coordenação de Aperfeiçoamento de Pessoal de Nível Superior (CAPES) and to Conselho Nacional de Desenvolvimento Científico e Tecnológico (CNPq).
Authors’ contribution
ZTMG: conceptualization, supervision, investigation, methodology, data curation, formal analysis, writing the original draft, writing review and editing; DCS: investigation, methodology, writing review and editing; MJF: conceptualization, investigation, funding acquisition, supervision, writing review and editing.
References
CrossRef | Gscholar
CrossRef | Gscholar
CrossRef | Gscholar
CrossRef | Gscholar
Gscholar
CrossRef | Gscholar
Gscholar
Online | Gscholar
CrossRef | Gscholar
CrossRef | Gscholar
Gscholar
Gscholar
Authors’ Info
Authors’ Affiliation
Coordination of Environmental Dynamics, National Institute of Amazon Research, Manaus, Amazonas (Brazil)
Department of Forest Science, Soils and Environment, São Paulo State University, School of Agronomic Sciences, Botucatu, São Paulo (Brazil)
Department of Forest Sciences, Federal University of Amazonas, Manaus, Amazonas (Brazil)
Corresponding author
Paper Info
Citation
Guimarães ZTM, Da Silva DC, Ferreira MJ (2024). Seedling quality and short-term field performance of three Amazonian forest species as affected by site conditions. iForest 17: 80-89. - doi: 10.3832/ifor4317-016
Academic Editor
Giorgio Vacchiano
Paper history
Received: Jan 28, 2023
Accepted: Dec 20, 2023
First online: Mar 21, 2024
Publication Date: Apr 30, 2024
Publication Time: 3.07 months
Copyright Information
© SISEF - The Italian Society of Silviculture and Forest Ecology 2024
Open Access
This article is distributed under the terms of the Creative Commons Attribution-Non Commercial 4.0 International (https://creativecommons.org/licenses/by-nc/4.0/), which permits unrestricted use, distribution, and reproduction in any medium, provided you give appropriate credit to the original author(s) and the source, provide a link to the Creative Commons license, and indicate if changes were made.
Web Metrics
Breakdown by View Type
Article Usage
Total Article Views: 3111
(from publication date up to now)
Breakdown by View Type
HTML Page Views: 768
Abstract Page Views: 741
PDF Downloads: 1518
Citation/Reference Downloads: 7
XML Downloads: 77
Web Metrics
Days since publication: 245
Overall contacts: 3111
Avg. contacts per week: 88.89
Article Citations
Article citations are based on data periodically collected from the Clarivate Web of Science web site
(last update: Feb 2023)
(No citations were found up to date. Please come back later)
Publication Metrics
by Dimensions ©
Articles citing this article
List of the papers citing this article based on CrossRef Cited-by.
Related Contents
iForest Similar Articles
Research Articles
Conservation of Betula oycoviensis, an endangered rare taxon, using vegetative propagation methods
vol. 13, pp. 107-113 (online: 23 March 2020)
Research Articles
Forecasting the field performance of Austrian pine seedlings using morphological attributes
vol. 10, pp. 99-107 (online: 13 October 2016)
Technical Reports
Effects of different mechanical treatments on Quercus variabilis, Q. wutaishanica and Q. robur acorn germination
vol. 8, pp. 728-734 (online: 05 May 2015)
Short Communications
Effect of four levels of shade on survival, morphology and chlorophyll fluorescence of Nothofagus alessandrii container-grown seedlings
vol. 8, pp. 638-641 (online: 08 January 2015)
Research Articles
Use of overburden waste for London plane (Platanus × acerifolia) growth: the role of plant growth promoting microbial consortia
vol. 10, pp. 692-699 (online: 17 July 2017)
Research Articles
Controlled-release fertilizers combined with Pseudomonas fluorescens rhizobacteria inoculum improve growth in Pinus halepensis seedlings
vol. 8, pp. 12-18 (online: 12 May 2014)
Research Articles
Growth, morphology, and biomass allocation of recently planted seedlings of seven European tree species along a light gradient
vol. 13, pp. 261-269 (online: 03 July 2020)
Research Articles
Substrates and nutrient addition rates affect morphology and physiology of Pinus leiophylla seedlings in the nursery stage
vol. 10, pp. 115-120 (online: 02 October 2016)
Short Communications
The effects of salicylic acid, oxalic acid and chitosan on damping-off control and growth in Scots pine in a forest nursery
vol. 13, pp. 441-446 (online: 24 September 2020)
Technical Reports
Nursery practices increase seedling performance on nutrient-poor soils in Swietenia humilis
vol. 8, pp. 552-557 (online: 09 December 2014)
iForest Database Search
Search By Author
Search By Keyword
Google Scholar Search
Citing Articles
Search By Author
Search By Keywords
PubMed Search
Search By Author
Search By Keyword