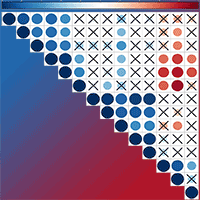
Fine root morphological traits and production in coniferous- and deciduous-tree forests with drained and naturally wet nutrient-rich organic soils in hemiboreal Latvia
iForest - Biogeosciences and Forestry, Volume 16, Issue 3, Pages 165-173 (2023)
doi: https://doi.org/10.3832/ifor4186-016
Published: Jun 08, 2023 - Copyright © 2023 SISEF
Research Articles
Abstract
Fine root production is one of the key elements of carbon (C) turnover in soil in afforested peatlands and forest lands with organic soils. We estimated variability in fine root morphology traits and annual production in hemiboreal forests dominated by coniferous trees (Norway spruce) and deciduous trees (silver birch and black alder) with nutrient-rich organic soils in Latvia. In total, 23 research sites were established in drained and naturally wet forests of different ages, and ingrowth core techniques were used to sample fine roots and subsequently determine fine root morphology traits and annual production, and calculate C input through fine root litter. Significant differences in several fine root morphological traits between coniferous- and deciduous-trees-dominated stands were found. Fine root production tended to be higher in coniferous-trees-dominated stands and positively correlated with several forest stand characteristics: stand age, average tree diameter at breast height, basal area and average tree height, but negatively correlated with nitrogen and phosphorus content in soil. C input through fine root litter ranged up to 0.46 ± 0.16 t ha-1 yr-1. It is necessary to conduct further research, including multi-annual estimates in a wider set of forest stands with diverse dominant tree species and growing conditions, to improve estimates, patterns and understanding of C flows through fine root litter in drained and naturally wet organic soils.
Keywords
Fine Roots, Hemiboreal Forests, Drained Organic Soil, Naturally Wet Organic Soil, Fine Root Production, Morphology
Introduction
Fine roots are short-lived, small-diameter (≤ 2 mm) roots associated with mycorrhizae, and they represent the main fraction of below-ground litter ([28], [11], [39]). Fine root net primary production constitutes an important but often insufficiently measured part of the C budget of forest ecosystems because below-ground litter C input is more laborious to quantify ([16], [31]). A better understanding of the factors affecting fine root production, especially in forests with nutrient-rich organic soils, which are considered to be a significant source of GHG emissions, is important for improving national-, regional-, and global-level C cycling models for forest ecosystems, and one of the approaches for gaining information is the collection of new empirical data ([11], [27]).
Growth of the root system is crucial both for the development of visible above-ground plant structures and for soil formation ([28], [39]). Fine roots play a key role in regulating biogeochemical cycles in forest ecosystems, especially of water and nutrients ([47], [34], [12]). Furthermore, fine roots form and provide the network where mycorrhizal associations can develop, improving tree nutrition, stress tolerance and disease protection ([12]). Fine roots also contribute to greenhouse gas (GHG) fluxes through, for instance, root respiration, rhizosphere methane (CH4) oxygenation and passive transport of CH4 to the atmosphere by aerenchymous roots ([18]). In addition, fine roots account for most of the surface area and length of forest root systems, and are therefore important components of forest biomass ([49]).
Fine roots are the most short-lived part of the root system ([34]). The lifespan of fine roots in woody plants, in particular of the smallest order rootlets, is typically not more than a few months ([8]). According to Fortier et al. ([12]), fine root production and mortality rates also fluctuate during the growing season, with production and mortality peaks in the spring and autumn.
Fine root production and anatomical and morphological traits vary widely due to genetic variability (plant functional type) and differing forest site types and local environmental conditions ([28], [39]). Fine root production and morphological traits are largely determined by environmental conditions such as soil temperature, soil water content (groundwater level), nutrient availability, strength (density and compaction), aeration and ecological interactions with other species including their fungal symbionts ([37], [56], [50], [55], [9], [45], [22]). The vertical fine root biomass distribution shows high densities of roots in the top soil layers and a gradual decrease of fine root biomass density with depth ([57]). Claus & George ([5]) found a clear effect of stand age on standing fine root biomass, with the highest fine root biomass in adult but not mature stands.
Through fine root turnover or uninterrupted growth and dieback fine roots play a significant role in forest C cycles, affecting the average C stock and accumulation rate in soil ([19], [21], [48], [11], [51], [32], [54]). For this reason, it is important to precisely estimate not only the standing biomass of fine roots, but also its rate of turnover ([60]). The availability of direct and reliable methods for measuring the turnover of fine roots is limited ([2]). The main reason for this is that the two component processes of root turnover - namely growth and dieback of fine roots - nearly always happen in the same place and at the same time ([20]). Our knowledge of the contribution of fine root life cycles to C cycles in forests still remains insufficient ([34]).
Forests with nutrient-rich organic soils have been highlighted due to its unclear role in achieving climate neutrality goals. To improve knowledge about hemiboreal forest ecosystems with nutrient-rich organic soils, the aim of this study was to evaluate fine root morphological traits and production (including C input through fine root litter) as well as the affecting factors in coniferous and deciduous tree forests with drained and naturally wet nutrient-rich organic soils in Latvia.
Materials and methods
Study sites
The study was performed in 23 research sites, established in 2020, representing typical forests with nutrient-rich organic soils in the hemiboreal region in Latvia (Fig. 1, Tab. 1). The research sites were dominated by coniferous trees (Norway spruce, Picea abies H. Karst.) and deciduous trees (silver birch, Betula pendula Roth and black alder, Alnus glutinosa Gaertn), respectively. All research sites were divided according to the soil moisture conditions (drained or naturally wet) based on the national forest site type classification system ([4]). Coniferous-trees-dominated stands included in the study represented stands with drained organic soils, but deciduous-trees-dominated stands represented both groups of soil moisture condition (drained and naturally wet organic soils). At sites with naturally wet nutrient-rich organic soils, the understorey is formed by Frangula alnus, Salix sp., Padus avium, Ribes nigrum and Viburnum sp.; in the herb layer species preferring wet conditions prevail, such as ferns, sedges, and on mounds Oxalis acetosella, Vaccinium myrtillus and Maianthemum bifolium; the moss layer is scarce but rich in species such as Caliergonella sp., Climacium sp., Plagiomnium sp., Calliergon sp., and on mounds Rhytidiadelphus sp., Hylocomium splendens, Eurhynchium sp. ([61]). In research sites with drained nutrient-rich organic soils, the understorey is formed by Frangula alnus, Sorbus aucuparia, Juniperus communis, Salix cinerea and Daphne mezereum; in the herb layer Vaccinium myrtillus, Oxalis acetosella, Dryopteris carthusiana, Calamagrostis sp., ferns, Cirsium oleraceum, and in the moss layer Hylocomium splendens, Pleurosium schreberi, Dicranum sp., Rhytidiadelphus, Brachythecium sp., Eurhynchium sp. and Cirriphyllum piliferum ([61]). The area of each sample plot (in total 25) was 500 m², with a radius in a plane of 12.62 m.
Tab. 1 - Stand parameters and characteristics of the research sites. (Age): stand age; (BA): basal area; (D): tree density; (GS): growing stock; (DBH): tree diameter at breast height; (H): tree height. Values are averages ± standard error, the range of values is in brackets.
Dominant species (no. sites) |
Soil moisture condition (no. sites) |
Age (yrs) |
BA (m2 ha-1) |
D (n ha-1) |
GS (m3 ha-1) |
DBH (cm) |
H (m) |
---|---|---|---|---|---|---|---|
Deciduous trees (13): silver birch (7), black alder (6) |
drained (6) | 38 ± 7 (19-62) |
20.0 ± 3.3 (11.0-33.7) |
1523 ± 353 (340-2660) |
172 ± 38 (58-307) |
16.6 ± 1.7 (10.3-21.8) |
16.4 ± 1.7 (10.0-22.0) |
naturally wet (7) | 44 ± 10 (10-73) |
24.1 ± 5.3 (2.5-39.9) |
3700 ± 1456 (1160-11700) |
272 ± 104 (8-809) |
12.9 ± 2.9 (3.3-23.0) |
14.1 ± 2.8 (4.6-25.6) |
|
Coniferous trees (10): Norway spruce (10) |
drained (10) | 52 ± 5 (27-74) |
26.6 ± 2.5 (14.6-38.5) |
825 ± 93 (362-1320) |
292 ± 31 (174-462) |
20.9 ± 0.8 (18.1-25.4) |
20.0 ± 0.7 (16.2-23.0) |
In 2020 and 2021, the weather conditions in Latvia were typical (representative) for the region and no significant deviations from the norm were detected, as well as did not variate significantly between research sites. In Latvia, the mean annual precipitation in 2020 and 2021 was 641.5 and 676.3 mm, respectively. The mean annual air temperature was +8.8 °C in 2020 and +7.0 °C in 2021 ([36]).
Fine root sampling and sample processing
The root ingrowth method was used, whereby root growth into in situ incubations of root-free soil ([52], [38], [58]) was employed to estimate annual fine-root production in the surrounding soil. Before installation, in-growth cores (diameter 40 mm, length 80 cm) were filled with local soil, which was roughly sieved to remove living and freshly-dead roots and rough wood. The cores were packed tightly to get similar bulk density with surrounding soil and thus avoid poor root growth and underestimates. Fine root (trees + understorey vegetation) samples were collected using three root ingrowth cores placed randomly in each sample plot (i.e., three root ingrowth cores per sample plot, totaling 75 root ingrowth cores) under uniform forest canopy. Ingrowth cores were inserted into the soil to a depth of 80 cm from the surface of the forest floor. Installation of root ingrowth cores was performed in May 2020, using a corer-installer and soil auger. Ingrowth core samples were collected once in May 2021 (covering one full calendar year), when all ingrowth core samples were removed.
All obtained samples were transported to the Latvian State Forest Research Institute (LSFRI) “Silava” laboratory (Salaspils, Latvia). In the laboratory, roots from samples were washed free of soil, and fine roots (diameter ≤ 2 mm) were separated. Collected fine roots were not segregated into the roots of trees and other understorey vegetation. Thus, pooled fine root samples (trees + understorey vegetation) were further used for determination of fine root morphological traits and dry mass. The dry mass was determined after determination of fine root morphological traits and drying of fine root samples at 70 °C to constant mass.
Determination of fine root morphological traits
The morphological traits of fine roots were investigated prior to drying. Each root sample (tree roots and understorey vegetation roots combined) was scanned (resolution 1200 dpi) using an EPSON Expression 12000XL. Fine root length density (m m-3 soil), specific root length (m g-1 dry fine root mass), volume density (cm3 m-3 soil), root surface area (m2 m-3 soil) and specific root surface area (m2 g-1 dry fine root mass), mean root diameter (mm), and fine root tip frequency expressed as tip number on a dry mass basis (n mg-1 dry fine root mass) were calculated from the scans and fine root biomass data using the WinRhizo™ 2019 software (Régent Instruments Inc., Quebec City, Canada).
Estimation of carbon input with fine root litter
The study assumed that annual fine root production is equal to annual fine root litter amount ([42], [11], [25], [31]). To calculate C input with fine root litter, it was assumed that the C content in root biomass was 48% for broadleaves-dominated stands and 51% for conifers-dominated stands ([26], [17], [1]).
Soil sampling and analysis
In all research sites, soil samples were collected every 10 cm of depth between 0 and 80 cm. Two sets of composite samples were collected in each research site. Soil samples were transported to the LVS EN ISO 17025:2018-accredited laboratory at the LSFRI “Silava” and were prepared for analyses according to LVS ISO 11464:2005. Soil bulk density was calculated from the mass and the volume of a soil sample according to LVS ISO 11272:2017. The following general chemistry parameters were determined: pH(KCl) was determined using a glass electrode in a 1:5 (volume fraction) suspension of soil in 1 mol L-1 potassium chloride (KCl) solution according to LVS EN ISO 10390:2022; total C (TC, g kg-1) and total nitrogen (TN, g kg-1) content were determined using elementary analysis methods (dry combustion) by LVS ISO 10694:2006 and LVS ISO 13878:1998, respectively. Organic carbon concentration (OC, g kg-1) was calculated as the difference between TC concentration and inorganic carbon (carbonate) concentration determined using a volumetry method (Eijkelkamp calcimeter), according to LVS ISO 10693:20914. Concentrated nitric acid (HNO3) extractable potassium (K, g kg-1) and phosphorus (P, g kg-1) was determined using inductively coupled plasma-optical emission spectrometry (ICP-OES) method. In addition, OC/TN (C/N) ratio was calculated as a proxy to characterise the decomposition of soil organic matter ([41], [53]).
Data analysis
Data processing and all statistical analyses were performed in the R environment (R v. 4.0.3, RStudio v, 1.3.1093). A Wilcoxon rank-sum test with continuity correction was used to evaluate possible differences in morphological traits and production of fine roots between the pooled research sites in groups of type of dominant tree species (coniferous or deciduous trees) and soil moisture conditions (drained or naturally wet). Correlations between morphological traits and production of fine roots, selected variables of soil general chemistry and forest stand characteristics were tested with Spearman’s ρ using a significance level of p < 0.05. Fig. 2 and Fig. 4 were prepared using R package “ggplot2”, and Fig. 3 was prepared using the “corrplot” and “Hmisc” packages.
Results
Morphological traits and production of fine roots
Fine root morphological traits showed relatively high variation even within forest stands with the same type of dominant tree species and soil moisture conditions (Fig. 2). In stands with drained organic soils, both mean fine root length density and specific root length was higher in deciduous-trees-dominated stands (2332 ± 1022 m m-3 and 30.6 ± 5.6 m g-1, respectively) than in coniferous-trees-dominated stands (1125 ± 121 m m-3 and 14.4 ± 1.2 m g-1, respectively). Similarly, mean fine root surface area and specific fine root surface area was higher in deciduous-trees-dominated stands (1.96 ± 0.90 m2 m-3 and 0.026 ± 0.003 m2 g-1, respectively) than in coniferous-trees-dominated stands (1.49 ± 0.16 m2 m-3 and 0.018 ± 0.001 m2 g-1, respectively). Statistically significant difference between deciduous-trees- and coniferous-trees-dominated stands was found for specific fine root length and specific fine root surface area.
Fig. 2 - Morphological traits and annual production of fine roots (average values ± standard error; tree and understorey vegetation roots combined). Different capital letters indicate significant differences among stands with coniferous- and deciduous-trees dominated stands with drained organic soils. Different lowercase letters indicate significant differences among stands with drained and naturally wet organic soils within the group of deciduous-trees dominated stands (no significant differences were found). The number of samples (n) for each grouping is shown.
In stands with drained organic soil, both average diameter and volume density of fine roots in coniferous-trees-dominated stands (0.44 ± 0.02 mm and 166 ± 19 cm3 m-3, respectively) were significantly higher than that in deciduous-trees-dominated stands (0.32 ± 0.03 mm and 142 ± 69 cm3 m-3, respectively), but the average fine root tip frequency per unit dry mass was significantly higher in deciduous-trees-dominated stands (17.6 ± 3.5 n mg-1 dry fine root mass) than in coniferous-trees-dominated stands (10.6 ± 1.7 n mg-1 dry fine root mass). No statistically significant differences in fine root morphological traits among deciduous-trees-dominated stands with different soil moisture conditions (drained or naturally wet) were found.
Similarly to fine root morphological traits, fine root production also showed a high variation. Nevertheless, in stands with drained organic soil included in the study, average fine root production was significantly higher in coniferous-trees-dominated stands (0.69 ± 0.08 t ha-1 yr-1) than in deciduous-trees-dominated stands (0.46 ± 0.15 t ha-1 yr-1), but no statistically significant differences in fine root production between deciduous-trees-dominated stands with different soil moisture condition were found.
Factors affecting fine root morphological traits and production
General forest stand characteristics and soil chemistry parameters at different depths (Tab. 2) were screened for relationships (Spearman’s correlations) with fine root morphological traits and production (Fig. 3, Fig. 4). Fine root production was positively correlated with stand age (ρ = 0.58), average tree diameter at breast height (ρ = 0.48), basal area (ρ = 0.45) and average tree height (ρ = 0.44). Several significant correlations were found between fine root morphological traits and stand characteristics as well (Fig. 3). Correlation analysis between fine root production and chemical characteristics of soil showed a negative correlations with TN content in soil at the 0-10 cm layer (ρ = -0.47) and P content in soil at the 0-10 and 10-20 cm layer (ρ = -0.46 and ρ = -0.48, respectively - Fig. 4). No significant correlations were found between fine root morphological traits and selected soil parameters, except a positive correlation between fine root volume density and P content in soil at the 50-80 cm layer (ρ = 0.51).
Tab. 2 - Characteristics of soil chemistry in research sites. (SBD): soil bulk density; (OC): organic carbon concentration; (TN): total nitrogen. Values are averages ± standard error.
Type of dominant tree species | Soil moisture condition | Soil depth (cm) |
SBD (kg m-3) |
OC (g kg-1) |
TN (g kg-1) |
C/N ratio |
K (g kg-1) |
P (g kg-1) |
pH (KCl) |
---|---|---|---|---|---|---|---|---|---|
Deciduous trees | drained | 0-10 | 239.8 ± 28.8 | 393.2 ± 40.0 | 27.4 ± 3.2 | 14.5 ± 0.5 | 0.73 ± 0.12 | 2.02 ± 0.55 | 5.0 ± 0.5 |
naturally wet | 0-10 | 281.3 ± 55.6 | 416.4 ± 53.2 | 21.9 ± 2.9 | 19.2 ± 7.0 | 0.57 ± 0.05 | 0.96 ± 0.20 | 4.2 ± 0.4 | |
Coniferous trees | drained | 0-10 | 228.7 ± 35.0 | 481.2 ± 27.0 | 21.8 ± 2.4 | 26.6 ± 6.3 | 0.46 ± 0.03 | 1.08 ± 0.15 | 4.1 ± 0.3 |
Fig. 3 - Spearman’s correlations between fine root morphological traits, fine root production and mean forest stand characteristics. Positive correlations are displayed in blue and negative correlations in red. Colour intensity and the size of the circle are proportional to the correlation coefficients. The legend below the correlogram shows the correlation coefficients and their corresponding colours. Correlations with p > 0.05 are considered non-significant (crosses are added).
Fig. 4 - Spearman’s correlations between fine root morphological traits, fine root production and soil characteristics. Positive correlations are displayed in blue and negative correlations in red. Colour intensity and the size of the circle are proportional to the correlation coefficients. The legend below the correlogram shows the correlation coefficients and their corresponding colours. Correlations with p > 0.05 are considered non-significant (crosses are added).
Carbon input through fine root litter
The average C input through fine root (tree and understorey vegetation) litter ranged from 0.038 ± 0.022 t ha-1 yr-1 in the youngest deciduous-trees-dominated stand with drained soil included in the study, to 0.46 ± 0.16 t ha-1 yr-1 in the coniferous-trees-dominated stands with drained soil, which were the oldest stands included in the study, with relatively high stem biomass parameters. The average annual C input through fine root litter in the research sites was 0.23 ± 0.03 t ha-1 yr-1. Linear regressions describing the dependence of annual C input through fine root litter (tree and understorey vegetation roots combined) on stand age, average tree diameter at breast height, basal area and TN content in soil at 0-10 cm depth are shown in Fig. 5.
Fig. 5 - Annual carbon input through fine root litter (tree and understorey vegetation roots combined) depending on stand age, average tree diameter at breast height, basal area and nitrogen content in soil at the 0-10 cm layer.
Discussion
Morphological traits of fine roots
Fine roots are one of the most physiologically active parts of plants and contribute significantly to below-ground C fluxes in forest ecosystems ([3], [11], [62]). Fine root morphological traits and production are sensitive to the connected environment and its changes ([62]). Several size-related traits of root morphology can reflect, for instance, resource availability, including nutrient and water ([43], [13], [62]). In addition, the root branching pattern of the species determines the morphological traits of fine roots ([46]). Several studies revealed significant differences in fine root morphological traits of coniferous and deciduous trees ([45], [46]), as well as the impact of tree age and soil microbial community on fine root parameters ([33], [45]).
Several traits of root morphology can be used as important indicators of a stand nutrient-uptake efficiency, and plant survival and growth strategies ([3], [62]). For instance, the specific surface area and specific length of fine roots reflects the fine root resource allocation and nutrient absorption ([62]). Generally, our estimates of fine root specific area were slightly lower compared with values previously reported in literature for stands with the same tree species in the Baltic Sea Region. Our fine root specific area values ranged from 12.5 to 44.8 m2 kg-1 dry fine root mass. In Estonia and Finland, studies revealed that the range of variation in mean specific root area was 29-74 m2 kg-1 for Norway spruce, 84-194 m2 kg-1 for silver birch ([43], [45], [46]), and 79-155 m2 kg-1 for black alder ([45]). However, these results reflect mycorrhizal roots (short roots) lacking secondary thickening and are morphologically distinct from other fine roots. In spruce stands in southeast Norway, mean specific root area expressed per unit soil volume ranged from 2.4 to 35.4 m2 m-3 ([3]) which is also slightly higher than our estimated range of corresponding values. Possible reasons for the lower fine root specific area values obtained within this study could be the depth from which fine roots were collected, that was greater in our study than in the abovementioned studies ([43], [45], [46], [3]). As fine root specific area decreases significantly from topsoil to deeper soil layers ([45], [3]), fine root sampling depth could explain lower mean fine root specific area values obtained within this study. In addition, our results reflect combined results of morphological traits of both trees and understorey vegetation which can cause differences compared to fine root morphological traits of trees only.
Our estimates of the range of variation in mean specific root length for Norway spruce were up to 23.7 m g-1 dry fine root mass, but up to 61.8 m g-1 dry fine root mass for the deciduous tree species. Ostonen et al ([45], [46]) also found that values of specific root length were higher for deciduous tree species (up to 204 m g-1 for silver birch) than for coniferous trees (up to 47 m g-1 for Norway spruce). Our results of specific root length for Norway spruce are in line with the results obtained in Norway spruce stands in southeast Norway where specific root length ranged from 13.4 to 19.8 m g-1 ([3]). Similar to the fine root specific area, specific root length tends to decrease from topsoil to deeper soil layers ([45], [3]), thus the greater fine root sampling depth could explain the lower mean fine root specific area values obtained within this study.
We found significantly higher average fine root tip frequency in deciduous-trees-dominated stands than in coniferous-trees-dominated stands. In general, our results are slightly lower than those obtained in Norway-spruce- and silver-birch-dominated stands in Finland and Estonia, where average short root tip frequency ranged from 19.9 ± 1.2 to 24.9 ± 1.2 n mg-1 dry short root mass for Norway spruce and from 54.4 ± 3.8 to 67.1 ± 6.6 n mg-1 dry short root mass for silver birch ([46]). Ostonen et al. ([45]) reported that average short root tip frequency in black-alder-dominated stands in Estonia ranged up to 126.4 ± 7.1 n mg-1 dry short root mass. In general, fine root tip frequency showed no consistent pattern and was mostly unaffected by stand age and stand above-ground biomass parameters. Similarly, Borja et al. ([3]) concluded that there was no significant effect of stand age on specific root tip density of fine roots in Norway spruce stands.
We found that Norway-spruce-dominated stands had a statistically higher average fine root diameter relative to the stands dominated by deciduous species. Ostonen et al. ([46]) observed a similar trend in their study and reported that statistically higher average short root diameter was found in Norway-spruce dominated stands (0.53 ± 0.02 mm in Estonia and 0.45-0.49 ± 0.01mm in Finland) when compared to silver-birch- and black-alder dominated stands in Estonia (0.32-0.34 ± 0.01 mm and 0.39 ± 0.01mm, respectively - [45]).
Production of fine roots and its affecting factors
In general, fine root production varies widely both within and among different types of boreal forests with organic soils ([27]). Yuan & Chen ([60]) estimated that the average production of fine roots (< 2 mm in diameter) in boreal forest ecosystems was 2.83 t ha-1 yr-1 (both mineral and organic soils were included). Furthermore, they found that broad-leaved species generally had lower fine root production than those dominated by needle-leaved species (2.01 ± 0.17 and 2.31 ± 0.12 t ha-1 yr-1, respectively - [60]). Similarly, Steele et al. ([55]) reported that production of fine roots was even four-fold higher in northern black spruce stands than in southern aspen stands in Canadian boreal forests. Finér et al. ([11]) estimated that average production of fine roots for all plants (trees + understorey) in boreal and temperate forest ecosystems was 3.11 ± 2.59 t ha-1 yr-1 and 4.28 ± 3.75 t ha-1 yr-1, respectively; however, mineral and organic (peatland) soils were not analysed separately. Lampela et al. ([27]) found that average fine root production in boreal forested peatland varied from 0.31 t ha-1 yr-1 to 1.20 t ha-1 yr-1; furthermore, there were no significant differences in total fine root production between the undrained and drained sites. Our estimates in forests with nutrient-rich organic soils showed that average fine root production ranged from 0.46 ± 0.15 t ha-1 yr-1 in deciduous-trees-dominated stands with drained organic soils to 0.69 ± 0.08 t ha-1 yr-1 in Norway spruce dominated stands with drained organic soils. Furthermore, we found a positive correlation between fine root production and stand age, average tree diameter at breast height, basal area and average tree height. These results are in line with previous findings in Finland demonstrating that stand basal area predicts fine root biomass better than any other stand variable alone ([29]). In addition, we found a negative correlation between fine root production and N and P content in soil as well as a positive correlation (although statistically insignificant) between fine root production and C/N ratio in soil. These trends could be explained by vegetation capacity to adapt to shortages in nutrients by increasing the absorptive capacity of the root system through extensive strategy enhancing root production and maintaining larger absorbing surface areas or through intensive strategy modifying root morphology and physiology in order to increase uptake efficiency per root mass ([13]). Several studies have demonstrated that fine root production is inversely related to soil nutrient status ([3], [6]) and N deposition ([59], [62]). Studies along boreal and temperate forest ecosystems showed that fine root biomass per tree stand basal area decreases significantly from nutrient-poor to nutrient-rich sites ([40], [7], [27]).
Another explanation for obtaining lower fine root production values than most values reported for boreal and temperate forests could be the use of ingrowth core method for the first year. First year results most likely reflect a lagged root growth due to cutting of the roots during the installation of ingrowth cores resulting in a slow rates of root recolonisation in the root-free cores ([55], [10], [35]). For instance, Steele et al. ([55]) concluded that the ingrowth core method provides an estimate of the minimum amount of biomass allocated to fine root production. Finér & Laine ([10]) emphasized that ingrowth core method provide a reliable and quantitative results comparable with, for instance, sequential coring method, if the time passed since the installation of bags is long enough (i.e. > 2 growing seasons). Ostonen et al. ([44]) also concluded that fine root production values from ingrowth cores were approximately two times smaller than those obtained by soil cores even in the third year. Thus, the first year results of fine root production obtained within this study using the ingrowth core method most likely provided a lowered values. Nevertheless, ingrowth core method in short-term studies (as in our case) can provide insight into the fine root growth potential and is suitable for comparing the effects of different dominant tree species, soil moisture condition and other affecting factors ([10]). Some additional drawbacks of the ingrowth core method were discussed by Vogt et al. ([58]) and Lukac ([34]).
Carbon input through fine root litter
The results of our study show a positive trend for C input through fine-root litter to increase along with stand age and average tree diameter at breast height and basal area, and a negative decreasing trend along with N and P content in soil at the 0-20 cm layer. In general, our average values of C input through fine root litter (up to 0.46 ± 0.16 t ha-1 yr-1) were slightly lower than the relatively wide range of other reported C fluxes from Norway spruce, silver birch and black alder forests in the boreal region. According to results of a study conducted in Sweden and Finland, the input of C with fine root litter in birch stands was 0.77 t C ha-1 yr-1 ([14]) and 1.16 t C ha-1 yr-1 ([6]), whereas in spruce stands it ranged from 0.51 to 1.32 t C ha-1 yr-1 ([23], [14], [30], [31]). Furthermore, their results showed that fine root lifespan was significantly shorter, and litter input higher, in warmer and more nutrient-rich soil. This should be considered in the context of global warming and increasing soil temperatures ([30], [6]).
In previous studies conducted in Latvia, modelled C annual input through tree below-ground litter in forests with drained organic soils based on stem biomass data was estimated to be 1.43 ± 0.07 t ha-1 yr-1 in Norway-spruce dominated stands and 1.70 ± 0.07 t ha-1 yr-1 in silver birch stands ([1]). Experimental outcomes from this study revealed that previously modelled annual C input through tree below-ground litter based on stem biomass data and several assumptions ([1]) could be overestimated for nutrient-rich organic soils. Although the longevity of fine roots varies depending from different affecting factors ([15], [31]), the fine root C inputs into soils are generally estimated by multiplying annual fine root production or fine root biomass multiplied by turnover rate (as the inverse of longevity) by C content in root biomass ([42], [23], [11], [31], [24]). Also, this study assumed that annual fine root production is equal to annual fine root litter amount. Certainly, this assumption comes with some limitations, for instance, the coexistence of fast cycling fine roots ([24]). Thus, it should be noted that the use of this assumption may slightly decrease accuracy of estimates of annual C input through tree below-ground litter as well.
Conclusions
In general, both fine root morphological traits and production show a high variation in nutrient-rich organic soils in Latvia. Nevertheless, significant differences in several fine root morphological traits and production between coniferous- and deciduous-trees dominated stands were found as well as fine root production was positively correlated with several forest stand characteristics, such as stand age, average tree diameter at breast height, basal area and average tree height. Because forest stand above-ground biomass characteristics can be estimated accurately and easily, our empirical data may provide useful information for predicting tree and understory vegetation fine root production and consequently C input through fine root litter at the stand level in hemiboreal forests with nutrient-rich organic soils. Furthermore, fine root production was negatively correlated with TN and P content in soil, reinforcing previously-reported trends that fine root biomass decreases from nutrient-poor to nutrient-rich sites.
Considering that our fine root production estimates in nutrient-rich organic soils are lower than values previously reported for boreal and temperate regions, it is necessary to conduct further research including multi-annual estimates in a wider set of forest stands with diverse dominant tree species and growing conditions to improve estimates, patterns and understanding of C flows through fine root litter in drained and naturally wet organic soils.
Acknowledgements
The study was implemented within the scope of the European Regional Development Fund project “Development of greenhouse gas emission factors and decision support tools for management of peatlands after peat extraction”, Grant agreement No. 1.1.1.1/19/A/064.
A. Bardulis contribution was supported by European Regional Development Fund, support for post-doctoral studies in Latvia “Evaluation of climate change mitigation potential of agroforestry systems with mineral and organic soils” (No.: 1.1.1.2/VIAA/4/20/684). A. Bardule and K. Polmanis contribution was supported by European Regional Development Fund project “Development of greenhouse gas emission factors and decision support tools for management of peatlands after peat extraction” (No.: 1.1.1.1/19/A/064). The authors thank Ivika Ostonen (the University of Tartu, Estonia) for valuable comments.
Acknowledgment to the project “Demonstration of climate change mitigation potential of nutrients rich organic soils in Baltic States and Finland” (LIFE OrgBalt, LIFE18 CCM/LV/001158) for field measurement data.
Author Contributions
Conceptualization: A. Lazdinš, A. Bardulis; methodology: A. Lazdinš, A. Bardulis; data curation: K Polmanis, L.L. Krumšteds; writing - original draft preparation: A. Bardule, K. Polmanis, A. Lazdinš, A. Bardulis; writing - review and editing: A. Lazdinš, A. Bardulis; visualization: A. Bardule, K. Polmanis, L.L. Krumšteds; supervision: A. Lazdinš.
References
Gscholar
CrossRef | Gscholar
Gscholar
CrossRef | Gscholar
Gscholar
Gscholar
Gscholar
Gscholar
CrossRef | Gscholar
Gscholar
CrossRef | Gscholar
Gscholar
CrossRef | Gscholar
Gscholar
Authors’ Info
Authors’ Affiliation
Kaspars Polmanis 0000-0003-2579-353X
Linards Ludis Krumšteds
Andis Bardulis
Andis Lazdinš 0000-0002-7169-2011
Latvian State Forest Research Institute “Silava”, Rigas str. 111, Salaspils, LV-2169 (Latvia)
Corresponding author
Paper Info
Citation
Bardule A, Polmanis K, Krumšteds LL, Bardulis A, Lazdinš A (2023). Fine root morphological traits and production in coniferous- and deciduous-tree forests with drained and naturally wet nutrient-rich organic soils in hemiboreal Latvia. iForest 16: 165-173. - doi: 10.3832/ifor4186-016
Academic Editor
Maurizio Ventura
Paper history
Received: Jul 18, 2022
Accepted: Mar 07, 2023
First online: Jun 08, 2023
Publication Date: Jun 30, 2023
Publication Time: 3.10 months
Copyright Information
© SISEF - The Italian Society of Silviculture and Forest Ecology 2023
Open Access
This article is distributed under the terms of the Creative Commons Attribution-Non Commercial 4.0 International (https://creativecommons.org/licenses/by-nc/4.0/), which permits unrestricted use, distribution, and reproduction in any medium, provided you give appropriate credit to the original author(s) and the source, provide a link to the Creative Commons license, and indicate if changes were made.
Web Metrics
Breakdown by View Type
Article Usage
Total Article Views: 16195
(from publication date up to now)
Breakdown by View Type
HTML Page Views: 13818
Abstract Page Views: 1123
PDF Downloads: 1100
Citation/Reference Downloads: 2
XML Downloads: 152
Web Metrics
Days since publication: 532
Overall contacts: 16195
Avg. contacts per week: 213.09
Article Citations
Article citations are based on data periodically collected from the Clarivate Web of Science web site
(last update: Feb 2023)
(No citations were found up to date. Please come back later)
Publication Metrics
by Dimensions ©
Articles citing this article
List of the papers citing this article based on CrossRef Cited-by.
Related Contents
iForest Similar Articles
Research Articles
Fine root production and distribution in the tropical rainforests of south-western Cameroon: effects of soil type and selective logging
vol. 3, pp. 130-136 (online: 27 September 2010)
Research Articles
Effects of understory removal on root production, turnover and total belowground carbon allocation in Moso bamboo forests
vol. 9, pp. 187-194 (online: 20 November 2015)
Research Articles
Thinning effects on soil and microbial respiration in a coppice-originated Carpinus betulus L. stand in Turkey
vol. 9, pp. 783-790 (online: 29 May 2016)
Research Articles
Impact of deforestation on the soil physical and chemical attributes, and humic fraction of organic matter in dry environments in Brazil
vol. 15, pp. 465-475 (online: 18 November 2022)
Research Articles
Soil stoichiometry modulates effects of shrub encroachment on soil carbon concentration and stock in a subalpine grassland
vol. 13, pp. 65-72 (online: 07 February 2020)
Research Articles
Effects on soil characteristics by different management regimes with root sucker generated hybrid aspen (Populus tremula L. × P. tremuloides Michx.) on abandoned agricultural land
vol. 11, pp. 619-627 (online: 04 October 2018)
Research Articles
Soil C:N stoichiometry controls carbon sink partitioning between above-ground tree biomass and soil organic matter in high fertility forests
vol. 8, pp. 195-206 (online: 26 August 2014)
Research Articles
Estimating changes in soil organic carbon storage due to land use changes using a modified calculation method
vol. 8, pp. 45-52 (online: 17 June 2014)
Research Articles
Modeling the fine root biomass dispersion using a special influence function
vol. 1, pp. 141-144 (online: 25 November 2008)
Research Articles
Dynamics of soil organic carbon (SOC) content in stands of Norway spruce (Picea abies) in central Europe
vol. 11, pp. 734-742 (online: 06 November 2018)
iForest Database Search
Search By Author
Search By Keyword
Google Scholar Search
Citing Articles
Search By Author
Search By Keywords
PubMed Search
Search By Author
Search By Keyword