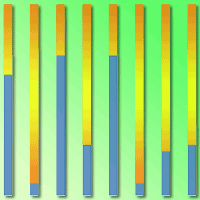
Carbon neutrality of forest biomass for bioenergy: a scoping review
iForest - Biogeosciences and Forestry, Volume 16, Issue 2, Pages 70-77 (2023)
doi: https://doi.org/10.3832/ifor4160-015
Published: Mar 05, 2023 - Copyright © 2023 SISEF
Review Papers
Abstract
The exploitation of forest biomass for bioenergy is commonly perceived as part of a broad strategy for climate change mitigation due to the view that forest biomass is carbon neutral. The aims of this study are to distinguish the most widely used definition of carbon neutrality and to identify the most frequently discussed aspects of the concept of carbon neutrality. This research is conducted in the form of a scoping review. The results of the scoping review demonstrated that there is no generally accepted definition of carbon neutrality. Eight main concepts of carbon neutrality were identified. The most frequently discussed aspects of the carbon neutrality concept were temporal and spatial boundaries, scenario-based assumptions, and the source of biomass feedstock. This research provides a comprehensive summary of the concept of carbon neutrality and contributes to the debate regarding forest biomass exploitation for bioenergy.
Keywords
Bioenergy, Biofuels, Carbon Cycle, Climate Change, Forest Residues, Life Cycle Assessment
Introduction
The evidence demonstrating the progression of global climate change (CC) is comprehensive and the phenomenon is widely accepted in the scientific community. Anthropogenic greenhouse gas (GHG) emissions are a significant factor contributing to CC ([25]). The gas making up the greatest share of GHGs emitted by human activities is CO2. According to data obtained in 2020, the amount of CO2 emitted globally is 32.018 million tons ([53], [7]); as of February 2022, the mean concentration of CO2 in the atmosphere has reached 417.81 parts per million ([40]). The Intergovernmental Panel on Climate Change (IPCC) acknowledges that “human influence on the climate system is clear, and recent anthropogenic emissions of greenhouse gases are the highest in history” ([24]). The global nature of climate change necessitates that the solutions for this problem be achieved through common efforts. For this purpose, many international scientific conferences and political conventions are focused on establishing international cooperation and drafting agreements dedicated to climate change mitigation. The IPCC is an example of such cooperation - a coalition dedicated to assessing the science related to climate change, established by the World Meteorological Organization and the United Nations Environment Programme. Furthermore, there are numerous international agreements dedicated to developing the policy mechanism for climate change mitigation such as those led by the United Nations Framework Convention on Climate Change (UNFCCC) - the Kyoto Protocol and the Paris Agreement. The Paris Agreement set the ultimate goal of keeping global average temperature increase below 2 °C above pre-industrial levels, while also pursuing efforts to limit the temperature increase to 1.5 °C ([54]). The European Council has developed several targets for climate change mitigation that should have been achieved by 2020. These targets included reducing GHG emissions to at least 20% below 1990 levels, improving energy efficiency to achieve a 20% reduction in primary energy use compared with projected levels, and increasing the share of renewable energy sources to 20% of EU final energy production ([2]).
Bioenergy is considered to be a part of the portfolio of solutions to combat climate change. For example, the Renewable Energy Directive II ([15]), the successor to the original version ([14]), is a major European Union directive that promotes the use of energy from biomass and other renewable energy sources. The overall target of the Renewable Energy Directive II (RED II) is to increase renewable energy to 32% of EU energy consumption by 2030. Energy derived from biomass is the largest renewable energy source utilized to meet the above-mentioned targets in Europe ([6]). A large share of bioenergy is derived from forest biomass. A popular point of view has been that forest biomass was a perfect substitute for fossil fuels due to its renewability and carbon neutrality (CN). Recently, however, the perception of carbon neutrality has been questioned within the scientific community ([41]). In the past decade, many studies have been published regarding the issue of carbon neutrality of forest biomass and the debate over this topic is far from over.
The current study investigates the problem of the carbon neutrality of forest biomass and contributes to the developing discussion. This research is conducted in the form of a scoping review, with the objectives of distinguishing the most widely used definition of carbon neutrality and identifying the most frequently discussed aspects of the carbon neutrality concept.
Methods
This study uses the scoping review approach, which describes existing literature and includes findings from a range of different study designs and methods. A scoping review is suitable for identifying and analyzing knowledge gaps, for clarifying general concepts and definitions in the literature, and for identifying key topics within a field of research ([34]). In this review, we utilized a framework that was originally introduced by Arksey & O’Malley ([5]), included recommendations from Preferred Reporting Items for Systematic Reviews and Meta-Analyses extension for scoping reviews ([52]), and incorporated guidelines for scoping review from Joanna Briggs Institute ([42]). The scoping review process used in this study includes five stages: (i) identifying research questions; (ii) identifying potentially relevant studies: (iii) selecting eligible studies; (iv) charting the data; and (v) summarizing and reporting findings.
Identifying research questions and objectives
The objectives of this study are to present the most widely used definition of carbon neutrality and the most frequently discussed aspects of the carbon neutrality concept. The research questions we developed are: (i) what is the definition of carbon neutrality? (ii) What are the main assumptions that carbon neutrality concepts are based on?
Identifying potentially relevant studies
In general, the step of identification of relevant studies casts a wide net for resource types such as electronic databases, peer-reviewed scientific articles, gray literature (e.g., regulatory data), working papers, etc. The databases chosen for this scoping review were Web of Science® (WoS) and Scopus®. The time frame of publication ranged from 1990 to 2020, with the time frame ending at the time the review was performed. The search strategy for both databases used the following key phrases: “carbon neutrality”, “carbon neutral biomass”, “carbon neutral bioenergy”, and “forest carbon neutrality.” The selected terms could be found in the title, the abstract, or the keywords. Furthermore, a forward-backward article search (also known as citation chaining) was applied. This search method collects references that are frequently cited in topical papers ([44]). The forward-backward search method was implemented during the process of study selection. In addition, several articles were obtained through direct contact with authors who are focusing on the study of carbon neutrality of forest biomass. For the study selection process of this scoping review, we developed a list of inclusion criteria: (i) We analyzed peer-reviewed articles and gray literature (e.g., working papers, reports, etc.); (ii) all publications must be written in English; (iii) we did not implement any restriction regarding the country of publication; (iv) all publications should be focused on the concept of carbon neutrality of forest biomass.
Selecting eligible studies
Fig. 1 summarizes the process of study selection. During the initial search using the chosen terms, we identified a total of 5699 articles - 2630 in WoS database and 3069 in Scopus database. During the screening process, we excluded 5463 articles that were not relevant to the topic based on the contents of the title and the abstract and 138 articles were excluded as duplicates. Thus, 98 articles were identified from WoS and Scopus databases and 40 articles were obtained through chaining and direct contact with authors. Finally, we performed a full-text analysis on the 138 articles. Some 99 studies were excluded based on inclusion criteria or accessibility - 87 studies did not have a focus that correspond with the objectives of this scoping review, 5 studies were not written in English, 1 study was not available in a digital format, and 6 studies were not accessible. Therefore, the final number of articles included in this scoping review is 39.
Charting the data
We used descriptive qualitative content analysis and developed a data-charting table in Microsoft Excel® for full-text analysis of eligible articles (n=39). This table includes the following information about analyzed articles: author, year of publication, type of publication, goal of the study, definition (concept) of carbon neutrality used in the article, comparison of fossil fuels to biomass with the focus on carbon neutrality, dimensions related to the concept of carbon neutrality considered in the article, and general perception of carbon neutrality of forest biomass.
Summarizing and reporting findings
The last stage is summarizing the data in relation to the purpose of the review, making conclusions, and noting any implications of the findings. This stage is thoroughly elaborated below in the results and discussion sections. The results section explains the CN concepts that were identified during the literature review and the discussion part of the study explains the assumptions that stay behind the CN concepts.
Results
Study context 39 articles were included in the scoping review after full-text analysis (see Tab. S1 in Supplementary material for full list). Although inclusion criteria had a time frame from 1990 to 2020, studies included in the scoping review were published starting in 2007 (Fig. 2). 15% of analyzed articles were published between 2007 and 2010, 46% of articles were published between 2011 and 2015, and 39% of articles were published between 2016 and 2020. Sixteen articles included in the scoping review were published in peer-reviewed journals, whereas 23 articles can be defined as gray literature.
Concepts of carbon neutrality
The concept of carbon neutrality of biomass is broad and often vague. Various scientists use this term differently according to their own understanding. As has been pointed out in many sources, there is no widely accepted definition of carbon neutrality ([39], [10], [28]). After analysis of 39 articles (Tab. S1 in Supplementary material), we distinguished 8 different concepts of carbon neutrality. A significant foundation of this research is the classification of carbon neutrality introduced by Malmsheimer et al. ([30]), which is based on the materials first presented by the National Council for Air and Stream Improvement ([38]). Additionally, we were able to contribute to the discussion on the carbon neutrality of biomass exploitation for bioenergy by ascertaining and defining concepts of carbon neutrality that were not previously described (CN of forest residues and waste, and CN of additional biomass). Below are the descriptions of each concept.
Carbon cycle neutrality
According to this concept, forest biomass that was harvested and subsequently replanted can be called carbon neutral ([18], [37]). Carbon cycle neutrality claims that the same amount of carbon (C) that was emitted to the atmosphere can be reabsorbed during the process of regrowth after a certain amount of time. Therefore, C simply circulates between atmosphere and biosphere. C released to the atmosphere during the process of biomass combustion creates a carbon debt. The process of C sequestration back into plant tissues during forest growth is called the payback period, which can take a few years for short rotation plantations to decades or hundreds of years for old-grown forests. Thus, the concept can be characterized as “harvest to regrowth”. C cycle neutrality is usually applicable at the stand level, meaning that biomass is harvested from one forest plot and regrowth occurs on the same plot. Other forest management activities existing in the rest of the forest are not considered.
Inherent carbon neutrality
This concept asserts that biomass is naturally carbon neutral because its combustion returns C to the atmosphere that was recently absorbed during the process of plant growth ([47], [20]). Unlike fossil fuels, which emit C that was sequestered deep in the earth for millions of years, biomass combustion does not add net C to the atmosphere. The inherent CN concept suggests that biomass establishes a C credit during the process of plant growth. When biomass is burned, C is released back to the atmosphere and creates a balance between absorbed and emitted carbon. This concept can be characterized as “growth to harvest”, the opposite of the perspective of carbon cycle neutrality. Yet both inherent CN and carbon cycle neutrality state that biomass C circulates in the closed biosphere-atmosphere system without adding net additional C.
Offset carbon neutrality
The amount of C released due to harvesting in a forest stand can be offset by C sequestration in other stands within the same forest ([46], [51]). Unlike carbon cycle neutrality, the offset carbon neutrality concept considers carbon stock of the forest as a system where one stand should not be analyzed in isolation from other stands. If the annual amount of C released from harvesting activities in the forest is less than or equal to the annual amount of C sequestered during the process of forest growth, there is no net reduction in C stock. Therefore, biomass from this forest can be called carbon neutral.
Carbon neutrality of forest residues and waste
Biomass can be considered carbon neutral if the C released during the process of combustion would otherwise be released into the atmosphere via the process of natural decomposition ([55], [12]). This concept can be applicable for forest residues that were left in the forest after any harvesting activities including clearcuts, thinnings, harvesting to reduce the risk of fire and pest diseases in the forest, and harvesting activities caused by construction works. In addition, the concept of carbon neutrality of forest residues and waste can be relevant to sawmill or papermill wooden waste.
Carbon neutrality of additional biomass
Biomass that is planted on non-forested land and is grown explicitly with a purpose to be used as a bioenergy source can be called carbon neutral ([57], [2]). If this biomass were not planted, the C it absorbs would have remained in the atmosphere. This principle is similar to “growth to harvest” and the inherent CN concepts. Biomass creates a C credit during growth that will be balanced to zero after biomass combustion without adding net C emissions to the atmosphere.
Accounting carbon neutrality
This concept is derived from the UNFCCC and Kyoto Protocol principles for international greenhouse gas reporting and accounting. According to these principles, C released during the process of biomass combustion should be counted as zero. The emissions are instead reported in the Land Use, Land Use Change and Forestry (LUCUCF) category at the stage of harvesting as a loss of C stock in the forest. Thus, emissions of C from tailpipes and smokestacks associated with biomass combustion for energy are not included in the emissions from the energy sector to avoid double-counting. Comparable to offset CN, this approach accounts for annual changes in C stocks but not C flows.
Substitution carbon neutrality
In the analysis of 39 articles, the concept of substitution carbon neutrality was rarely defined as an independent concept. However, its principles are relevant to many other CN concepts. Within the framework of this concept, biomass can be considered carbon neutral if the net emissions of C released during the process of combustion of biomass for energy are less than C emissions associated with the exploitation of alternative energy sources ([30], [10]). Normally, this concept is not limited to quantifying emissions that occur directly from combustion. When biomass is compared to alternative energy sources (e.g., other renewable energy sources or fossil fuels), it is common practice to use methods of Life Cycle Assessment (LCA). The International Organization for Standardization ([26]) defines LCA as the “compilation and evaluation of the inputs, outputs and the potential environmental impacts of a product system throughout its life cycle”. A distinctive feature of LCA when applied to the comparison of energy sources, is its assessment of all C emissions released over the life cycle, including throughout the steps of production. For example, LCA of biomass will assess the total amount of C released at the stages of harvesting, transportation, processing, conversion, and combustion.
Life cycle carbon neutrality
According to this concept, biomass can be called carbon neutral if it does not release net C emissions to the atmosphere during its life cycle ([39], [27]). In other words, the C emissions of biomass should be less than or equal to the amount of C sequestered in the biomass. This concept highlights the necessity of using LCA to assess life cycle emissions of the biomass. Unlike substitution CN, the life cycle CN concept assesses the balance between C released during combustion and the C sequestered during the process of biomass growth without a required comparison of biomass to alternative energy sources.
During full-text analyses of the articles included in this scoping review, we observed that the most frequently mentioned concepts of carbon neutrality are “carbon cycle neutrality” and “offset carbon neutrality”. How often the different carbon neutrality concepts were mentioned in the analyzed articles is shown on Fig. 3. It is important to note that we included concepts specifically mentioned as related to carbon neutrality as well as those that were only described without being identified as carbon neutrality by the authors. The average number of carbon neutrality concepts mentioned per article is 3-4.
Discussion
Despite the fact that there are numerous concepts of carbon neutrality that could facilitate the use of forest biomass for bioenergy, the overarching idea as well as each individual concept have received a considerable amount of criticism. A key factor that can affect CN of biomass is the method used for biomass assessment. In the analysis of articles, we observed that many authors suggest using LCA to assess carbon neutrality of forest biomass. Currently, the LCA method is widely recognized and applied to bioenergy assessment ([11], [56], [31], [35]). Almost all CN concepts (except accounting carbon neutrality) require an assessment of C emissions that can apply this method. The LCA method is not inherently a carbon neutrality concept but is an essential tool to evaluate emissions of a biofuel during its life cycle. Life cycle emissions include supply chain emissions (harvesting, transportation, processing) and emissions from combustion. LCA uses different input parameters to estimate C emissions and the carbon neutrality of biomass will depend on the parameters used for the analysis ([21]). These parameters include the type of biomass feedstock, aspects of biomass management and harvesting, means of transportation, the methods of biomass conversion and combustion, temporal and spatial parameters, and the time frame for feedstock replenishment. Some CN concepts highlight a single parameter while others focus on a combination of these parameters. For example, carbon neutrality of forest residues and waste is focused on the origin of the biomass feedstock. Carbon cycle neutrality highlights feedstock replenishment, whereas inherent carbon neutrality prioritizes the temporal aspect of biomass production. Other CN concepts focus on a combination of these parameters; for instance, the offset CN concept considers both temporal and spatial boundaries, and additional biomass CN distinguishes between origins of biomass feedstock as well as considers the temporal aspect. However, life cycle carbon neutrality concept is unique in its reliance on the LCA method and has the most thorough assessment of all parameters that can affect the CN status of a biofuel. The concept of substitution CN can also implement the LCA method to assess how bioenergy production compares to alternative energy production scenarios in terms of C emissions.
Temporal boundaries
While assessing C emissions produced during the entire life cycle of biomass, one of the challenges is to properly define the time frame of the analysis. This element of analysis is the establishment of “temporal boundaries”. Depending on the length of the designated time boundaries, biomass would be characterized as carbon neutral when C emissions from bioenergy production will be less than C sequestration in a selected time frame. It can be achieved if the boundaries will include C reabsorption via biomass regrowth after harvesting for bioenergy or C sequestration during the initial biomass growth. This parameter is relevant for concepts of carbon neutrality including carbon cycle CN, life cycle CN, inherent CN, and CN of additional biomass. Thirty-four out of 39 articles analyzed during the scoping review discussed at least one of these CN concepts, thereby considering temporal aspects. There are many opinions about when the accounting period should begin since this choice can influence the perception of biomass as CN and there is no agreement on which temporal boundaries should be applied across the board. The debate is ongoing as scientists provide individual explanations for the ideal temporal boundaries with which to determine carbon neutrality. One option is to begin accounting at the time of first biomass harvesting for bioenergy ([6]). In this case, biomass extraction creates C debt that later can be repaid via replanting of biomass. Another option is for accounting to start at the point of biomass planting and growth (relevant for inherent CN and CN of additional biomass) where biomass initially creates C credit.
It is just as important to determine when the accounting period should end. One option is to account for growth prior to harvesting, harvesting activities, and full biomass regrowth ([27]). A relatively long time frame is suggested because forest management activities commonly occur before and continue after the usual timeframe of LCA analysis. On the one hand, longer temporal boundaries will allow for the carbon debt that occurs at the moment of harvesting to be repaid by regrowth and describe the GHG mitigation benefits provided by stable forest carbon stocks ([18]). On the other hand, it is argued that longer temporal boundaries should not be applied when assessing CN of biomass because they overshoot established Paris Agreement targets and hinder efforts to mitigate climate change ([13]). In many cases, the actual carbon payback period is very long ([22]). When biomass is combusted, it immediately increases the concentration of C in the atmosphere, and it is theorized that a delay between C release and C sequestration could have a temporary warming effect ([36]). Some scientists argue that burning biomass with a long payback period has a potential impact on climate tipping points in the short term ([3], [8]). The concept of “climate tipping points” implies that an increase in global temperatures past a certain point could potentially cause an irreversible global shift from the current stable climate to a warmer stable climate and many subsequent negative consequences ([8]). For example, thawed permafrost and melted glaciers would not return to their original magnitude at a higher temperature equilibrium even after full carbon emissions offset by biomass regeneration. Nevertheless, this issue has not yet been sufficiently studied and research is ongoing.
Spatial boundaries
While assessing the emissions released from biomass, it is also important to correctly identify the physical border of the study area. This aspect of assessment is referred to as spatial boundaries. Just like temporal boundaries, spatial boundaries can influence the calculation of biomass emissions ([36]). Therefore, whether or not an examined biomass type is carbon neutral depends on selected spatial boundaries. This aspect is the foundation of offset carbon neutrality. There are two common options for spatial boundaries: plot-level approach and landscape-level approach. The plot-level approach assesses a single plot with harvesting at year 0 followed by subsequent regrowth. In that case, the amount of time required for regrowth (payback period for C sequestration) will be significant. The landscape-level approach considers an entire forest area. According to this approach, emissions from harvesting on one plot can be offset by forest growth in other plots in the same forest. Therefore, biomass from this forest can be considered carbon neutral if the total carbon stock remains the same or increasing despite the undertaken harvesting activities. The more widely held opinion is that the landscape-level approach is the most suitable for assessing the C emissions of biomass ([30], [12], [23]) and that the minimal spatial boundaries should include the entire supply area ([39], [18]). One reason for this constraint is the possibility to account for “leakage”. Leakage can be defined as unexpected emissions or additional sequestration associated with forest management activities within the forest plot but occurring outside of the assessed area ([18]). Plot-level assessment does not consider emissions or sequestration related to unexpected forest activities, whereas the landscape-level approach allows for more thorough measurement within a larger area of consideration.
However, some argue that the C balance in the areas of the forest unaffected by direct forest management activities is irrelevant and that emissions of a specific bioenergy project cannot be offset by biomass growth in the adjacent area ([13]). In addition, a report published by the Manomet Center for Conservation Sciences ([33]) points out that landscape-level assessment ignores the scenario in which biomass was not harvested for bioenergy (i.e., business as usual scenario) because it is hard to predict forest management activities when applying wider spatial boundaries. Considerations in biomass assessment, such as the BAU scenario, and possible omissions of foregone C sequestration will be explained in the next section.
Scenario-based assumptions
As is pointed out by many authors ([19], [32]), it is essential to apply assumptions from different scenarios when assessing GHG emissions of biomass. This component of assessment can be applied to all CN concepts. When assessing biomass, it is reasonable to compare the “bioenergy scenario” - the scenario in which biomass is harvested for energy production through processes of its combustion - with the scenario in which biomass is never harvested for bioenergy production. This scenario can be called baseline, business-as-usual (BAU), reference, or counterfactual scenario. However, BAU scenarios can vary. For example, if a forest has been actively managed with a certain amount of biomass consistently harvested, the BAU scenario assumes that the same amount of biomass will continue to be harvested in the future. Conversely, if forest management activities were absent in the assessed forest area, the BAU scenario assumes that the forest will remain untouched and continue to absorb carbon as it grows. The process of ongoing plant growth in a non-bioenergy scenario is called foregone carbon sequestration. Foregone carbon sequestration poses a challenge to the promotion of forest biomass for bioenergy production and to its categorizing as carbon neutral. Many authors are concerned that encouraging the harvest of biomass for energy will lead to the loss of potential carbon stock ([16], [36], [3], [28]). Additionally, biomass can only reduce C emissions if the total C sequestered after the processes of growth and harvesting of biomass is greater than the amount that would have been sequestered anyway ([45]). While they may be important to consider, counterfactual scenarios are often hard to predict. There are a variety of factors that can impact future forest conditions that may or may not make biomass suitable as a carbon neutral source for energy production. The potential risk of natural disturbances like fires, storms, or pest diseases is often mentioned among these factors ([32]). Another consideration is market effects. The conditions of the current economic market (e.g., prices of biomass), or even the anticipation of future markets, can affect forest owner behavior. Making decisions regarding forest management activities based on expected future markets can be called a forward-looking approach ([47], [48]). According to this approach, high market prices for biomass, or anticipated high prices in the near future, can increase forest owner investment and improve forest management. Improvement of forest management can mean new plantings, the use of more rapidly growing seedlings, the intensification of management to increase quality and quantity of feedstock, and expansion of forests ([48], [50], [12], [37]). In that case, these positive effects should be included in the BAU scenario. However, market effects can also have negative impacts on the forest. High prices of timber, as well as large subsidies for bioenergy, can increase the rate of harvesting. If harvestings are not conducted within the sustainable forest management framework, the C stock can eventually be diminished ([48], [13]). Forest owner behavior, market effects, and natural ecosystem dynamics are difficult to forecast. Consequently, the fate of C stocks is difficult to predict as it is closely associated with these factors. This demonstrates why assessments of the exploitation of biomass for bioenergy should precisely define alternative scenarios and include all the details that can affect the results of the assessment.
Many authors assert that it is necessary to account for supply chain emissions when assessing emissions from biomass ([12], [28], [41]). Even though the energy content of the fossil fuels used for harvesting, chipping and transportation is typically less than 5% of the energy content of the processed biomass ([6]), emissions related to the combustion of these fossil fuels should be included in LCA. According to this logic, a comprehensive comparison between a bioenergy scenario and a counterfactual scenario in which only fossil fuels are used should consider the C sequestration of the biomass that continues to grow in the counterfactual scenario as well as the supply chain emissions from fossil fuels used for mining (or harvesting), transportation, and production in either scenario. This idea is relevant to the substitution CN concept.
Feedstock
The feedstock of biomass for bioenergy is an important factor examined in the analyzed articles. Selection of biomass feedstock affects the overall suitability of its use as an energy source. Currently, the main feedstocks used for bioenergy consist of byproducts from the production of pulp, paper, and sawn timber, as well as from forest residues left after various forest management activities ([58]). Forest residues and wastes seem to be favorable biomass feedstocks that can positively contribute toward climate change mitigation ([9], [12], [41]). According to the principle of CN of forest waste and residues, this biomass can be defined as C neutral if used for bioenergy because it would decay and emit C into the atmosphere if it had been left unused.
A related dilemma is whether it is appropriate to harvest biomass in old-growth forests or if it is better to maintain it as a carbon pool. There are two interconnected aspects regarding this issue. First, some authors assert that forest C sequestration capacity is limited with age ([20], [51]). Rates of carbon uptake decline as forests reach maturity. Whereas young forests grow rapidly and sequester higher amounts of C, old-growth forests eventually stop absorbing additional C and only function as C storage. Second, old-growth forests have a higher risk of natural disturbances. There is general agreement that the risk of forest fires, storm fellings, and insect attacks increases with the age of the trees ([30], [20]). The loss of C pools in an old-growth forest as a result of natural disturbances would be significantly greater than if the disturbance occurred in a young forest.
The link between climate change and forest fires ([1], [17]) has increased the frequency and severity of wildfires in recent years and has led to the degradation of both old-growth and young forest areas. As of October 10th, 2022, land burned by wildfires in Europe was estimated at 1.4 million hectares. This is a significant increase considering about 0.45 million ha of land were burned in Europe on average between 2006 and 2021 ([49]). It is recognized that high tree stand density correlates with high fire severity ([4]). Accordingly, forest management practices can positively affect wildfire mitigation. Research shows that forest fuel treatments (i.e., thinning and prescribed fire) can decrease fire severity, crown fire occurrence, and lower C emissions related to wildfire ([43]). Moreover, Madrigal et al. ([29]) demonstrated that collection of forest biomass for energy production reduces fire risk if forest management activities are done at a landscape level and if biomass is derived from thinning and understory clearing. Thus, proper forest management facilitates wildfire mitigation while providing biomass resource for bioenergy production.
Conclusion
Despite the wide use of the term carbon neutrality, an analysis of recent literature demonstrated the lack of a common definition of the concept. We were able to identify eight distinct definitions of CN from 39 analyzed articles. These definitions include the following carbon neutrality concepts: carbon cycle neutrality, Inherent carbon neutrality, offset carbon neutrality, carbon neutrality of forest residues and waste, carbon neutrality of additional biomass, accounting carbon neutrality, substitution carbon neutrality, and life cycle carbon neutrality. The majority of investigated studies used more than one definition of CN. Based on the analysis of the selected articles, CN is not an inherent property of biomass. Meanwhile, the inherent status as carbon neutral is frequently used to promote and justify the utilization of biomass for bioenergy purposes. Using the term in a generic sense can be confusing and even misleading given the variety of concepts of CN. Therefore, the concept of CN should perhaps instead be understood as a relative characteristic of a bioenergy product based on an assessment of the carbon emissions and environmental impact caused by its production and exploitation. This definition is most correlated with the life cycle carbon neutrality concept.
Biomass used for bioenergy production can be labeled as carbon neutral using a clear assessment procedure. This can be accomplished by applying the LCA method. LCA provides a tool to investigate all GHG emissions throughout the biofuel life cycle starting at the biomass plantation phase, following through the processes of biomass preparation and transformation, and ending with biofuel combustion. Due to the context-reliant nature of the concept of carbon neutrality of biomass, the results of the LCA scenario that utilizes biomass for bioenergy purposes should be compared to the results of an alternative scenario in which conventional fossil fuels are used as the source of energy production. Furthermore, it should be noted that the LCA of a particular biomass can be affected by systemic, temporal, or spatial boundaries. Therefore, these boundaries should be thoroughly defined before finalizing any assessment.
A topical example of biomass assessment is within the framework of RED II. The term “carbon neutrality” is not explicitly stated in RED II ([15]), but the CN concepts used can be identified from the directive’s guidelines for assessing the sustainability of biomass fuels and associated GHG emissions during its life cycle. The directive argues that carbon emissions from biofuel combustion should be treated as zero which indicates the principles of inherent carbon neutrality. Additionally, RED II agrees with the principles of the offset CN concept. The directive recognizes biomass as sustainable and proposes that it can be used for bioenergy production as long as levels of carbon stocks and sinks in the forest are maintained after harvesting. Overall, the methodology recommended for sustainability and GHG emissions assessment provided in RED II correlates with the life cycle CN concept which considers emissions that occur during the biomass cultivation, transportation, processing, etc. Nevertheless, RED II does not clearly define temporal boundaries which could affect the results of the assessment suggested in the methodology. Temporal boundaries, as well as the feedstock- and scenario-based assumptions mentioned earlier in this article, should be considered by stakeholders and policymakers when discussing bioenergy production.
Acknowledgements
We thank Alisa Royer Selivanova for her assistance in English Language revision.
References
Gscholar
Online | Gscholar
Gscholar
CrossRef | Gscholar
Gscholar
Gscholar
Gscholar
Gscholar
CrossRef | Gscholar
Gscholar
Gscholar
Gscholar
Gscholar
CrossRef | Gscholar
CrossRef | Gscholar
Gscholar
Authors’ Info
Authors’ Affiliation
Pavel Cudlín 0000-0003-1464-5160
Department of Ecosystem Functional Analysis of the Landscape, Global Change Research Institute CAS, Belidla 986/4a, 603 00, Brno (Czech Republic)
Department of Forest Ecology, Faculty of Forestry and Wood Technology, Mendel University in Brno, Zemedelska 3, 613 00, Brno (Czech Republic)
Department of Xylogenesis and Biomass Allocation, Global Change Research Institute CAS, Belidla 986/4a, 603 00, Brno (Czech Republic)
Corresponding author
Paper Info
Citation
Selivanov E, Cudlín P, Horáček P (2023). Carbon neutrality of forest biomass for bioenergy: a scoping review. iForest 16: 70-77. - doi: 10.3832/ifor4160-015
Academic Editor
Giorgio Alberti
Paper history
Received: Jun 17, 2022
Accepted: Dec 10, 2022
First online: Mar 05, 2023
Publication Date: Apr 30, 2023
Publication Time: 2.83 months
Copyright Information
© SISEF - The Italian Society of Silviculture and Forest Ecology 2023
Open Access
This article is distributed under the terms of the Creative Commons Attribution-Non Commercial 4.0 International (https://creativecommons.org/licenses/by-nc/4.0/), which permits unrestricted use, distribution, and reproduction in any medium, provided you give appropriate credit to the original author(s) and the source, provide a link to the Creative Commons license, and indicate if changes were made.
Web Metrics
Breakdown by View Type
Article Usage
Total Article Views: 25573
(from publication date up to now)
Breakdown by View Type
HTML Page Views: 20923
Abstract Page Views: 2435
PDF Downloads: 1852
Citation/Reference Downloads: 10
XML Downloads: 353
Web Metrics
Days since publication: 856
Overall contacts: 25573
Avg. contacts per week: 209.13
Article Citations
Article citations are based on data periodically collected from the Clarivate Web of Science web site
(last update: Mar 2025)
Total number of cites (since 2023): 3
Average cites per year: 1.00
Publication Metrics
by Dimensions ©
Articles citing this article
List of the papers citing this article based on CrossRef Cited-by.
Related Contents
iForest Similar Articles
Research Articles
An assessment of climate change impacts on the tropical forests of Central America using the Holdridge Life Zone (HLZ) land classification system
vol. 6, pp. 183-189 (online: 08 May 2013)
Research Articles
Assessing food sustainable intensification potential of agroforestry using a carbon balance method
vol. 12, pp. 85-91 (online: 24 January 2019)
Research Articles
Factors of soil CO2 emission in boreal forests: evidence from Central Siberia
vol. 16, pp. 86-94 (online: 19 March 2023)
Research Articles
Seeing, believing, acting: climate change attitudes and adaptation of Hungarian forest managers
vol. 15, pp. 509-518 (online: 14 December 2022)
Research Articles
Potential impacts of regional climate change on site productivity of Larix olgensis plantations in northeast China
vol. 8, pp. 642-651 (online: 02 March 2015)
Research Articles
Comparison of alternative harvesting systems for selective thinning in a Mediterranean pine afforestation (Pinus halepensis Mill.) for bioenergy use
vol. 14, pp. 465-472 (online: 16 October 2021)
Research Articles
Life cycle assessment of tannin extraction from spruce bark
vol. 10, pp. 807-814 (online: 25 September 2017)
Research Articles
Approaches to classifying and restoring degraded tropical forests for the anticipated REDD+ climate change mitigation mechanism
vol. 4, pp. 1-6 (online: 27 January 2011)
Research Articles
Predicting the effect of climate change on tree species abundance and distribution at a regional scale
vol. 1, pp. 132-139 (online: 27 August 2008)
Research Articles
Impact of climate change on radial growth of Siberian spruce and Scots pine in North-western Russia
vol. 1, pp. 13-21 (online: 28 February 2008)
iForest Database Search
Search By Author
Search By Keyword
Google Scholar Search
Citing Articles
Search By Author
Search By Keywords
PubMed Search
Search By Author
Search By Keyword