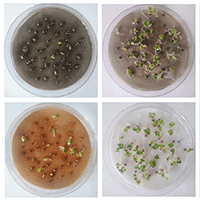
Physiological dormancy and dormancy release of Sassafras tzumu, a colored-leaf tree species with high landscape and economic value
iForest - Biogeosciences and Forestry, Volume 15, Issue 5, Pages 349-355 (2022)
doi: https://doi.org/10.3832/ifor4031-015
Published: Sep 01, 2022 - Copyright © 2022 SISEF
Research Articles
Abstract
Sassafras tzumu is one of the most valuable tree species in southern China. However, the dormancy of the seed limits its seedling quality. In order to improve the germination percentage of seeds, characteristics, causes of dormancy, changes in endogenous hormones and nutrients during storage were investigated. The results showed that seed viability was 78.11%, and the highest water absorption rate was 26.09%. The germination percentage of the embryo without cotyledon was 21.13%, but intact seed and embryo could not germinate on hormone-free medium. Seed embryo and coat extracts were found to have a significant inhibitory effect on seed germination. Germination percentage were significantly positively correlated to free amino content (p < 0.01) and negatively correlated to soluble sugar content (p < 0.05) during storage. The levels of GA3 and GA3/ABA had significant effects on the germination percentage of S. tzumu seed storage at 4°C. The results suggest that S. tzumu seeds are in intermediate physiological dormancy at maturity and are mainly caused by the presence of inhibitory substances in the seed tissues. Furthermore, changes in endogenous hormones and metabolism of nutrients at 4°C can significantly promote the release from dormancy of S. tzumu seeds.
Keywords
Sassafras tzumu, Seed Dormancy, Wet Sand Storage, Phytohormones, Nutrients
Introduction
Seeds are the main units of dispersal of most plants and allow colonization of new geographic areas, which is of great significance to the continuation of species ([2], [12]). To regenerate successfully, it is essential for plants to produce viable seeds ([41]). The germination of seed is crucial to the propagation of plant species ([30], [22]). To reduce the impact of adverse environments, seeds of many species are dormant until environmental conditions become favourable, which is a trait exhibited by newly produced seeds ([13], [20], [21]).
Based on numerous studies, it is obvious that many seeds are dormant at maturity. A freshly matured dormant seed (or other germination unit) is said to have primary dormancy, which develops during seed maturation on the mother plant ([5]). In addition to the environment factors, the endogenous factors such as embryo development, seed coat and phytohormones also play an important role in seed dormancy. The seed coat has been shown to regulate permeability to water, oxygen, or germination inhibitor, and is closely related to seed permeability in many species ([33], [1], [47]). Generally, when the seed coat is removed, respiration and water absorption are enhanced. Seeds with testa-imposed dormancy will germinate following damage or removal of the testa ([14]). Phytohormones play a key role in seed development processes such as maturation, dormancy and germination. The balance between abscisic acid (ABA) and gibberellic acid (GA) is considered to be the key to seed dormancy and germination, but not the absolute concentration or content of ABA and GA ([2], [11], [34]). Generally, bioactive GA contributes to embryo development ([26]), and ABA is the most prominent germination-inhibiting hormone in dormancy and germination control ([9], [6]). The sharp changes of indole acetic acid (IAA) are recognized as one of the first signals leading to embryogenesis ([38]) and inhibition of seed germination of seeds to a certain degree ([31], [25]). The Zeatin riboside (ZR) has also been confirmed to be closely related to seed dormancy and germination ([46]). In addition, each phytohormone has a specific effect on the germination process, either acting alone or working together.
Sassafras tzumu is one of the most important species in the south of China ([8]), and it has begun to come into focus as a colored-leaf tree in recent years ([24]). The S. tzumu is propagated from seeds, however, it is considered that the seeds of S. tzumu are dormant at maturity ([49]). Therefore, a suitable preservation technology is essential to maintain seed vitality and ensure the continuous supply of viable seeds. Dormancy release by cold stratification has been reported in many species, especially those with physiologically dormant seeds ([5], [41], [45]). In production practice, cold stratification for 8 months can release the dormancy of S. tzumu seeds and more than 90% of embryo release the dormancy under 0-5 °C ([43], [49]). However, the effects of cold stratification on dormancy release and physiological characteristics of S. tzumu are still unclear. To improve the understanding of dormancy in S. tzumu seeds, three questions would be discussed in this study: (i) what are the characteristics of S. tzumu seeds? (ii) What are the causes of seed dormancy and the type of dormancy? (iii) What preparation for seed dormancy release and germination during storage? The research will provide some new insights and support for the reproduction of S. tzumu.
Materials and methods
Study area and species
S. tzumu seeds were collected from the Research Institute of Subtropical Forestry, Chinese Academy of Forestry, Zhejiang, Hangzhou, China (119° 57′ E, 30° 03′ N). The mature fruits were collected in batches from June to July in 2018. Seeds were collected after removing the pericarp and impurities.
Seed characteristics
Seeds were randomly selected by quartering and the color of the seed coat was assessed. The vertical and horizontal diameter of the seeds were measured using a Vernier caliper. One hundred seeds were weighed to calculate the 1000-grain weight. For initial seed moisture measurement, about 5 g seeds were dried in an oven at 103 °C until constant weight, and the percentage of moisture was calculated based on fresh weight ([4]). The seeds were cut longitudinally and placed in 0.4% 2.3,5-triphenyl-tetrazolium chloride (TTC) solution for 3 h at 30 °C, and the seeds with pink or red embryos indicated viability ([22]). Intact seeds were placed in a 100-mL beaker, soaked with distilled water, and allowed to swell at 25 °C. The seeds were weighted for six times every 2 h and then weighted every 4 h until constant weight. All measurements were repeated thrice. The water absorption rate (WAR, %) and water uptake rate (WUR, g h-1) were determined by using the following equations (eqn. 1, eqn. 2):
where Wi represent the initial weight of seeds, Wn is the weight of seed at weighed n-th, and Tn is the processing time at weighed n-th.
Germination test
The mature seeds were placed in sand: leaf mold (1:3) at 15 °C, 20 °C, 25 °C, 30 °C, 35 °C, 25 °C/15 °C, 30 °C/15 °C, 30 °C/20 °C (14h/10h, light/dark), and the germination percentage was recorded 60 days (d) later, respectively.
To determine which tissues contributed to prevent germination in dormant S. tzumu seeds, different seed tissues were sequentially removed and the germination percentage was evaluated. Seeds were sterilized with 70% ethanol for 1 minute, 2% sodium hypochlorite for 10 minutes, followed by washing four times in sterile water. The intact seed, embryo (seed without coat) and embryo without cotyledon were inoculated on Murashige-Skoog (MS) medium. Both the hormone-free MS medium and the MS with 1 g L-1 GA3 were used, which were supplemented with 3% sucrose and 0.8% agar at pH 5.8. All cultures were incubated in a chamber at 25 °C ± 1 °C with 10 h dark and 14 h light, and the germination percentage was recorded 60 days later. Each experiment was repeated with 100 seeds thrice.
Bioassay of seed extracts
The seed coat and embryo parts with a weight of 200 g were separately grounded, and then extracted with 500 mL of 80% methanol at 4 °C for 24 h. This extraction process was repeated once. The extract solution was successively extracted by petroleum ether, ether, ethyl acetate and methanol. All extract solutions were concentrated to 100 mL. The Brassica campestris seeds were used to test the germination inhibitory substances activity ([6], [15]). Two pieces of filter paper were placed in each petri dish, and 3 mL of the extracts were added to the dishes. When the organic solvent was completely volatilized, approximately 3 mL of water was added to each petri dish. The B. campestris seeds were placed in petri dishes and germinated in a phytotron at 25 °C. The germination percentage was calculated after 48 h. Seedling height and root length were measured after 72 h. Each experiment with 100 seeds was repeated thrice.
Treatment and preservation of seeds
Seeds were mixed with wet sand (disinfected with 0.5% KMnO4) in the proportion of 1:3, and packed in self-sealed bags (25 × 17 cm). Three holes were cut to maintain enough air permeability and stored at 4 °C in refrigerator for 240 days, whereas the control was stored at 25 °C (three replicates). The sands were turned over and the right amount of distilled water was added every 15 days to maintain moisture. The seeds were taken out after storage for 0 d (S0), 60 d (S60), 120 d (S120), 180 d (S180), and 240 d (S240) and stored at -80 °C after frozen in liquid nitrogen until analysis.
Endogenous hormones and seed reserves
Samples (1.0 g) using 10 mL of 80% (v/v) pre-cooling methanol at 4 °C for 12 h in dark, followed by centrifuging at 10.000×g at 4 °C for 15 min ([6]). The final 2 µL of filtrate solution was used for the quantification of hormones using an ultra-performance liquid chromatography system (model 1290 Infinity®, Agilent Corp., Santa Clara, CA, USA). The content of ABA, GA3, IAA and ZR was determined using the external standard method and was expressed as μg g-1 FW ([45]).
Soluble sugar content was measured by using anthrone colourimetry ([28]). The seeds were transferred to a pre-cooled mortar and ground into a power before being mixed with 10 mL phosphate buffer solution (0.05 mol L-1, pH=7.8) that had been kept on ice. The homogenate was centrifuged and the soluble protein was measured by Coomassie brilliant blue G-250 staining method ([44]). After seeds were ground, concentration of fat was determined by the Soxhlet extraction method ([35]). The free amino acid of seeds was measured by using ninhydrin. Three biological replications were performed.
Statistical analyses
All data was processed using the package Excel® 2019 (Microsoft, Redmond, WA, USA). Multiple comparisons were used to determine the differences between germination percentage, root length and seedling height of B. campestris seed by using the least significant difference (LSD - [6]) in SPSS® ver. 19.0 (IBM, Armonk, NY, USA), and drawn with Origin® 2018 (Northampton, MA, USA). The relationship between physiological parameters and germination percentage of S. tzumu seeds during wet sand storage was analyzed by R v. 4.0.3 ([29]) using the packages “psych” ([32]) and “corrplot” ([40]).
Results
Characteristics and germination test of S. tzumu seeds
The mature seeds were nearly circular with an average diameter of 5.00 mm, 1000-grain weight of 61.74 g and water content of 8.58%. Seed viability was 78.11%, which shows that the seeds have a high germination potential, and it is the premise of seed preservation (Tab. 1). The water absorption rate sharply increased in the earlier 12 h, slightly increased from 12 h to 24 h, and finally 26.09% of the initial weight of water was absorbed. The highest water uptake rate was 0.125 g h-1 in the initial 2 h. The intact seed stopped absorbing water after 24 h (Fig. 1).
Tab. 1 - Characters of S. tzumu seeds.
Seed characteristics | Measured value |
---|---|
Colour of seed coat | Black |
Transversediameter | 5.24 ± 0.16 mm |
Longitudinal diameter | 5.02 ± 0.14 mm |
1.000-grain weight | 61.74 ± 0.51 g |
Water content | 8.58 ± 0.09 % |
Seed viability | 78.11 ± 4.59% |
The results showed that mature seeds could not germinate at different temperatures placed on the medium sand:leaf mold (1:3, v:v). Intact seed and embryo could not germinate on hormone-free medium, but 13.33% embryo germinated on medium with 1 g L-1 GA3. In addition, the germination percentage of embryo without cotyledon on medium with hormone-free and 1 g L-1 GA3 were 21.13% and 77.14%, respectively (Tab. 2). These results suggested that excised embryo produces normal seedling, and proved that embryo fully developed at maturity. Following our reasoning, however, the seed coat or cotyledon inhibited the germination of S. tzumu seeds.
Tab. 2 - Germination characteristics of S.tzumu seeds.
Medium | Seed type | GA3 g·L-1 | Germination percentage (%) |
---|---|---|---|
MS | Intact seed | 0 | 0 |
MS | Embryo | 0 | 0 |
MS | Embryo without cotyledon | 0 | 21.13 ± 1.25 |
MS | Intact seed | 1.00 | 0 |
MS | Embryo | 1.00 | 13.33 ± 0.58 |
MS | Embryo without cotyledon | 1.00 | 77.14 ± 1.34 |
Inhibitory effect of S. tzumu seed extract
Compared to the control, the ethyl acetate extracts both in seed embryo and seed coat most significantly affected B. campestris seed germination percentage (p < 0.01). The effects of the water extract and ethyl acetate extract, methanol extract, and ether extract on germination percentage were significantly different (p < 0.01). However, compared with the petroleum ether extract, the difference was not significant. In seed embryo and seed coat, the germination percentage of B. campestris using ethyl acetate extract was 2.64% and 7.18%, respectively. As compared to the control, the extracts of S. tzumu seeds significantly affected the growth of B. campestris roots (p < 0.01). The shortest roots of B. campestris were 0.2 mm (methanol extract of seed kernel added) and 0.16 mm (ethyl acetate extract of seed coat added). B. campestris seeds did not normally grow in the presence of ethyl acetate extract and methanol extract of the seed embryo and showed a significant difference in seedling height compared to the others (p < 0.01). In terms of the seed coat, the most significant effect on seeding height of B. campestris was observed using ethyl acetate extract, followed by methanol extract (Fig. 2).
Fig. 2 - The effect of different solvent extracts on B. campestris seed germination. The different lowercase letters (p < 0.05) and uppercase letters (p < 0.01) indicate significant differences. The abbreviation E-PEE, E-EE, E-EAE, E-ME, and E-WE, respectively, stand for the petroleum ether extract, ether extract, ethyl acetate extract, methanol extract, water extract of seed embryo, whereas C-PEE, C-EE, C-EAE, C-ME, and C-WE stand for extracts of seed coat.
Contents and ratio of seed endogenous hormones
The content of IAA in seeds initially decreased and then increased during the wet sand storage at 4 °C, and it was higher than that at 25 °C. The content of GA3 storage at 25 °C rapidly increased in the first few days (S60 to S120), the highest value (4.82 ng g-1 FW) was found at S60 and then gradually decreased (S180 to S240). The content of GA3 gradually increased with storage at 4 °C. The ABA profile was similar to the IAA. The lowest level of ABA at 4 °C and 25 °C were observed at S120 (110.93 ng g-1 FW) and S60 (84.04 ng g-1 FW), respectively. The levels of ZR storage at 25 °C were higher than that at 4 °C (S120 to S180), and the opposite was observed at S210 and S240 (Fig. 3).
The ratio of IAA/ABA initially decreased and then increased with storage at 4 °C, and the lowest was at S180. However, during storage at 25 °C, the ratio of IAA/ABA increased (S0 to S60), gradually decreased (S60 to S180), and then rapidly increased (S180 to S240). The level of GA3/ABA rapidly increased with storage at 25 °C; the highest value was found at S60, and then sharply decreased (S60 to S240). The ratio of ZR/ABA decreased after an initial increase; moreover, it was always higher with storage at 25 °C than storage at 4 °C (Fig. 4).
Nutrients of S. tzumu seeds during storage
The content of soluble sugar, soluble protein, and free amino acid was higher at 4 °C than at 25 °C except for S240 (soluble protein). The level of soluble sugar gradually decreased during wet sand storage. The content of soluble protein showed the opposite trend at 4 °C and 25 °C with two peaks and two valleys at S60 and S180 separately. Fat content sharply decreased in the early stage of the storage (S0 to S60) and remained relatively constant during S120 to S240. The content of free amino acid first sharply increased (S0 to S60), slightly decreased (S60 to S120), and then sharply increased (S120 to S240) at 4 °C, and the highest was at S240 (4.6 mg g-1). In contrast, the content of free amino acid steadily increased with storage at 25 °C (Fig. 5).
Germination percentage and effect of physiological parameters during storage
The germination percentage of S. tzumu seeds increased sharply with the extension of storage time. Germination was first observed at S60 (2.22%) and S120 (7.78%) under two temperature levels. After 8 months of wet sand storage, the germination percentage of S. tzumu seeds was 54.4% (stored at 4 °C) and 37.8% (stored at 25 °C - Fig. 6). Therefore, wet sand storage could accelerate seed dormancy release, and low temperature has a better curative effect. In seeds, significant positive correlations were found between germination percentage and GA3 (p < 0.05), GA3/ABA (p < 0.05), and free amino (p < 0.01), and negatively associated with soluble sugar (p < 0.05) storage at 4 °C. However, the germination percentage was negatively correlated to soluble sugar (p < 0.01) and positively correlated to free amino (p < 0.01) storage at 25 °C (Tab. 3).
Tab. 3 - Relationship between physiological parameters and germination percentage of S. tzumu seeds during storage.
Physiological parameters |
Germination percentage of S. tzumu seeds | |||
---|---|---|---|---|
Storage at 4°C | Storage at 25°C | |||
Correlation (r) |
Probability values (p) |
Correlation (r) |
Probability values (p) |
|
IAA | -0.028 | 0.921 | -0.161 | 0.568 |
GA3 | 0.855 | <0.001 | -0.487 | 0.066 |
ABA | 0.286 | 0.301 | -0.107 | 0.705 |
ZR | 0.261 | 0.347 | -0.499 | 0.058 |
IAA/ABA | -0.301 | 0.276 | -0.113 | 0.689 |
GA3/ABA | 0.616 | 0.015 | -0.331 | 0.228 |
ZR/ABA | -0.048 | 0.867 | -0.215 | 0.442 |
Soluble sugar | -0.581 | 0.023 | -0.787 | <0.001 |
Soluble protein | -0.320 | 0.244 | 0.287 | 0.299 |
Fat | -0.488 | 0.065 | -0.486 | 0.066 |
Free amino | 0.802 | <0.001 | 0.885 | <0.001 |
Discussion
Seed characteristics and dormancy types
Seeds may possess various morphological and physiological characteristics that partially represent the plant’s response to selective pressure within the current environment during plant evolution ([37]). S. tzumu is always growing in sparse or dense forests ([42]), and previous research has suggested that ripe seeds are often scattered around the seed-bearer and only a few migrated with birds ([16]). In addition, it is difficult for seeds to reach distant areas through wind transport under natural conditions with a diameter of 5.00 mm and a 1000-grain weight of 61.74 g. A recent research has indicated that about 8.53%-27.22% of ripe fruits are without embryos ([8]), thus implying that the number of available seeds may be even lower. Water uptake is a fundamental requirement for intact seed germination ([17]) and is related to the structure of seed coat ([39]). In our study, a sufficient amount of water was absorbed in 12 hours to support the germination of seeds. The germination test, however, indicated that mature seeds cannot germinate, indicating that seeds are dormant at maturity. From an ecological perspective, the dormancy of S. tzumu seeds prevents germination and allows the seedlings to avoid severe winter conditions.
In this study, intact seed and embryo could not germinate on hormone-free medium. However, the germination percentage of the embryo without cotyledon on hormone-free medium was 21.13%. The results suggest that there may be a substance in the cotyledon or seed coat that significantly inhibits the germination of seeds. A bioassay test of S. tzumu seed extracts confirmed that extracts significantly inhibited radicle elongation and seedling height growth, which was also observed in other types of dormant seeds such as Taxus yunnanensis seeds ([6]) and Grevillea sp. seeds ([27]). Physical dormancy (PY) is caused by one or more water-impermeable layers of palisade cells in the seed coat, and the lack of imbibition in intact seeds serves as the hallmark for the identification of physical dormancy ([5]). In this study, intact seeds rapidly imbibed water and increased in mass by 23.6% in the first 12 h. Therefore, we conclude that S. tzumu seeds are not physically dormant at maturity. The mature seeds cannot germinate, but the excised embryo produces normal seedling on MS medium with GA3 (1 g L-1). The germination percentage of embryo without cotyledon on medium with 1 g L-1 GA3 was 77.14%, which proved that the embryo was fully developed at maturity. In addition, the treatment of cold stratification significantly improved the percentage of seed germination. According to the dormancy classification of Baskin & Baskin ([5]), we can conclude that seeds of S. tzumu had intermediate physiological dormancy at maturity.
The balanced relationships among plant hormones play an important regulatory role in seed dormancy, including breaking seed dormancy and improving germination ([3]). During the entire process of seed dormancy release, the contents of IAA decreased slightly and then increased. Generally, GA contributes to embryo development and increases seed germination ([26]). In our study, the content of GA3 slightly increased, which was similar to previous findings in Paeonia lactiflora ([23]). Moreover, a positive correlation was found between the germination percentage of S. tzumu and GA3 content (p < 0.05) with storage at 4 °C. The decrease in ABA content during long periods of cold stratification will trigger germination ([7]). In the seeds of Arabidopsis sp., physiological dormancy is associated with high levels of ABA and during dormancy breaking, ABA levels decrease ([36]). However, the content of ABA was relatively stable except for S120 at 4 °C in this study, which suggested that the germination of S. tzumu seeds was not insensitive to the absolute concentration of ABA. Furthermore, a positive correlation was observed between germination percentage and GA3/ABA (p < 0.05) that also proved that the balance between GA3 and ABA significant affects dormancy release.
The quantitative and qualitative differences in seed nutrients may be related to the germination characteristics of species ([48]), and different seed nutrients may have different roles during germination ([18]). Generally, the soluble sugar contents decreased with the extension of storage time. However, due to environmental stress and seed identity, the situation is more complicate. Lower total soluble sugar content has been associated with higher germination in Jatropha curcas seeds ([10]), but the opposite was observed during the storage of Pseudolarix kaempferi seeds ([19]). In this study, soluble sugar content decreased and was lower at 4 °C than at 25 °C, which may be due to weak respiration and less energy consumption storage at 4 °C. The dynamic changes in soluble protein and free amino acid play an important role in the process of seed internal material metabolism. During storage, the protein contents fluctuate with storage time and the amino acid contents gradually increase. This phenomenon indicates that protein synthesis and metabolism are active in seeds. However, the germination percentage is positively correlated to free amino and had no significant relationship with soluble protein. Our experiments showed that seeds have high fat content after harvest (55.4%) and rapidly decompose in the first two months. It is possible that seeds may retain vitality by decomposing a large amount of fat at the earlier stage of storage.
Conclusions
Overall, S. tzumu seeds are in intermediate physiological dormancy at maturity with high seed viability. The seeds normally absorb water and the physiological dormancy mainly result from the germination inhibitors in seed embryo and coat. Wet sand storage at 4 °C is an effective, low-cost method of breaking S. tzumu seed dormancy. Furthermore, the levels of GA3, GA3/ABA, soluble sugar, and free amino significantly affect the germination percentage of seeds during 4 °C storage. The results improve understanding of the S. tzumu seed dormancy, and provide the basis for seed preservation and reproduction for this specific plant species.
Acknowledgments
HC designed the study, conducted the experiment, and wrote the manuscript; JL, JJ and YL supervised the experiments at all stages and reviewed the manuscript; ZT supported the experimental material collection. All authors read and approved the final manuscript.
This research was supported by the Forestry science and technology innovation project of Jiangxi Forestry Department (201804) and the Zhejiang Science and Technology Major Program on Agricultural New Variety Breeding (2021C02070-7).
References
Gscholar
Gscholar
Gscholar
Authors’ Info
Authors’ Affiliation
Jingmin Jiang
Jun Liu 0000-0003-2365-9214
Zifeng Tan
Yanjie Li 0000-0001-7750-757X
Research Institute of Subtropical Forestry, Chinese Academy of Forestry, Fuyang 311400 (China)
Corresponding author
Paper Info
Citation
Chen H, Jiang J, Liu J, Tan Z, Li Y (2022). Physiological dormancy and dormancy release of Sassafras tzumu, a colored-leaf tree species with high landscape and economic value. iForest 15: 349-355. - doi: 10.3832/ifor4031-015
Academic Editor
Federica Brunoni
Paper history
Received: Dec 02, 2021
Accepted: Jul 05, 2022
First online: Sep 01, 2022
Publication Date: Oct 31, 2022
Publication Time: 1.93 months
Copyright Information
© SISEF - The Italian Society of Silviculture and Forest Ecology 2022
Open Access
This article is distributed under the terms of the Creative Commons Attribution-Non Commercial 4.0 International (https://creativecommons.org/licenses/by-nc/4.0/), which permits unrestricted use, distribution, and reproduction in any medium, provided you give appropriate credit to the original author(s) and the source, provide a link to the Creative Commons license, and indicate if changes were made.
Web Metrics
Breakdown by View Type
Article Usage
Total Article Views: 25973
(from publication date up to now)
Breakdown by View Type
HTML Page Views: 22898
Abstract Page Views: 1673
PDF Downloads: 922
Citation/Reference Downloads: 1
XML Downloads: 479
Web Metrics
Days since publication: 1045
Overall contacts: 25973
Avg. contacts per week: 173.98
Article Citations
Article citations are based on data periodically collected from the Clarivate Web of Science web site
(last update: Mar 2025)
Total number of cites (since 2022): 2
Average cites per year: 0.50
Publication Metrics
by Dimensions ©
Articles citing this article
List of the papers citing this article based on CrossRef Cited-by.
Related Contents
iForest Similar Articles
Research Articles
Is there an effect of storage depth on the persistence of silver birch (Betula pendula Roth) and rowan (Sorbus aucuparia L.) seeds? A seed burial experiment
vol. 14, pp. 224-230 (online: 06 May 2021)
Review Papers
Should the silviculture of Aleppo pine (Pinus halepensis Mill.) stands in northern Africa be oriented towards wood or seed and cone production? Diagnosis and current potentiality
vol. 12, pp. 297-305 (online: 27 May 2019)
Research Articles
The effect of seed size on seed fate in a subtropical forest, southwest of China
vol. 9, pp. 652-657 (online: 04 April 2016)
Research Articles
Seed trait and rodent species determine seed dispersal and predation: evidences from semi-natural enclosures
vol. 8, pp. 207-213 (online: 28 August 2014)
Research Articles
Influence of mother plant and scarification agents on seed germination rate and vigor in Retama sphaerocarpa L. (Boissier)
vol. 7, pp. 306-312 (online: 08 April 2014)
Research Articles
Potential for utilization of wood ash on coastal arenosols with limited buffer capacity in KwaZulu-Natal and its effect on eucalypt stand nutrition and growth
vol. 10, pp. 180-188 (online: 19 November 2016)
Research Articles
Wood production and nutritional status of Pinus taeda L. in response to fertilization and liming: a meta-analysis of the Americas
vol. 16, pp. 195-201 (online: 25 July 2023)
Review Papers
Genetic diversity and forest reproductive material - from seed source selection to planting
vol. 9, pp. 801-812 (online: 13 June 2016)
Research Articles
Variation of wood and bark density and production in coppiced Eucalyptus globulus trees in a second rotation
vol. 9, pp. 270-275 (online: 08 September 2015)
Research Articles
Seed germination traits of Pinus heldreichii in two Greek populations and implications for conservation
vol. 15, pp. 331-338 (online: 24 August 2022)
iForest Database Search
Search By Author
Search By Keyword
Google Scholar Search
Citing Articles
Search By Author
Search By Keywords
PubMed Search
Search By Author
Search By Keyword