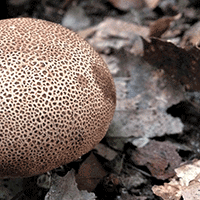
Not all long-distance-exploration types of ectomycorrhizae are the same: differential accumulation of nitrogen and carbon in Scleroderma and Xerocomus in response to variations in soil fertility
iForest - Biogeosciences and Forestry, Volume 14, Issue 1, Pages 48-52 (2021)
doi: https://doi.org/10.3832/ifor3594-013
Published: Jan 18, 2021 - Copyright © 2021 SISEF
Short Communications
Abstract
Long-distance-exploration type (LDET) ectomycorrhizae have been reported to be best adapted to infertile soils, but variation within LDET ectomycorrhizae have not been thoroughly examined. Concentrations of nitrogen (N) and carbon (C) in LDET ectomycorrhizae were examined in Xerocomus-Pinus sylvestris and Scleroderma-Quercus petraea ectomycorrhizae. The study determined how concentrations of these elements vary in ectomycorrhizae in fertile (organic, uppermost mineral) and infertile (brunic) soil layers. The organic horizon in both Scots pine and sessile oak forest soils had the highest mineral status and exchange cations. In contrast, low mineral concentrations, high base saturation, and pH were characteristic of the brunic horizon in both forest stands. Xerocomus ectomycorrhizae had a higher concentration of N in the fertile (organic and uppermost mineral) soil horizons (3.4%) than in the infertile (brunic) soil horizon (2.2%). N concentration in Scleroderma ectomycorrhizae varied from 2.8%-3.0 % and did not differ between the studied soil horizons. The mean concentration of carbon in Xerocomus ectomycorrhizae varied from 29%-46% in Scots pine stands and from 41%-44% in Scleroderma ectomycorrhizae in sessile oak stands. The concentration of carbon in both Xerocomus and Scleroderma ectomycorrhizae was significantly higher in the fertile horizons (organic and uppermost mineral) compared to the brunic (infertile) horizon. In summary, the analysis conducted in the present study indicates that the LDET ectomycorrhizae, Xerocomus and Scleroderma, possess inherent variations in C and N content to manage soil resources.
Keywords
Ectomycorrhiza, Soil Interaction, Soil Nitrogen, Nitrogen Utilization, Ectomycorrhizal Adaptation, Soil Chemistry
Introduction
Contact, short-, medium-, long-distance-, and mat-forming-exploration types of ectomycorrhizae can be distinguished based on their morphology and the biomass of emanating hyphae and rhizomorphs ([1], [2]). Each of the exploration types may be characterized by a distinct foraging strategy, however, the variation that exists within exploration types and its function under field conditions is poorly understood. Trees have evolved a variety of nutrient-foraging strategies that include variation in root functional traits, as well as an investment in hosting specific ectomycorrhizae ([6], [7], [8]). Whether spatial partitioning occurs in the functioning within a single long-distance ectomycorrhizal exploration type (LDET) along a substrate-resource gradient, however, has not been determined.
Ectomycorrhizal exploration-types differ in their ability to utilize resources and how they explore soils determines their efficiency ([20], [3], [29]). Species with extensive mycelia have been reported to be very efficient in nitrogen uptake and sequestration ([19], [33], [13]). Thus, fungal exploration strategies can affect tree nutrition ([43], [9]) due to their differential accumulation and utilization of C and N ([40]).
Nitrogen levels in fine roots are controlled by carbohydrate fluxes emanating from the host tree ([35], [30], [41]), but ectomycorrhizal fungi also differ in the level of N they provide to their hosts ([14]). The relationship between soil N availability and N content in ectomycorrhizae has been characterized under controlled conditions ([16], [17]). How ectomycorrhizae within a specific exploration type employ resources from soils that differ in their fertility in a natural forest environment, however, has not been examined.
In the present study, the long-distance ectomycorrhizae, Xerocomus and Scleroderma, were used to examine LDET ectomycorrhizae in hosts with contrasting life strategies: pioneer Scots pine (Pinus sylvestris L) and mid-successional sessile oak (Quercus petraea Liebl.). These tree species also vary in their nutritional requirements. Scots pine can grow in infertile and dry soils ([32]), while sessile oak generally prefers more fertile soils ([11]). Their root systems also differ, while sessile oak quickly forms a taproot capable of reaching deep soil depths and levels of ground water ([25]), the root system of Scots pine is fibrous and extensive, and their depth of rooting is not as great as in sessile oak ([34]).
We previously reported that root function is the most significant driver defining levels of root N in a specific soil type, however, we also reported a significant decrease in the concentration of soil and root N in infertile soil layers ([42]). Notably, the concentration of N in first-order roots was up to 20-fold higher than it was in the soil layer in which the roots were growing. This finding indicates that there is a strong need to provide roots with nitrogen irrespective of the N levels present in the surrounding soil environment. In the present study, N and C concentrations in LDET ectomycorrhizae were assessed to better determine the presence of natural variation in response to soil resources. LDET ectomycorrhizae, among all exploration types, have been reported to be the most efficient in nutrient foraging; however, there is a high cost for the host-plant to provide LDET ectomycorrhizae with C ([19], [33], [13]). We hypothesized that N and C concentrations in the LDET ectomycorrhizae, Xerocomus and Scleroderma, would not be affected by the fertility of the soil layer: the nutrient concentration in the ectomycorrhizae will be independent from soil nutrient level. We did not aim to discover the exact mechanism for this observation in the current study, but it possibly can be obtainable by some internal mechanism in the ectomycorrhizae (either plant or fungal) regulating the level of nutrient acquisition from the surrounding soil.
Materials and methods
Study site and roots collection
Soil samples were collected in September/October 2013 and 2019 from 90-100-year-old monoculture stands of sessile oak and Scots pine, located in the Zielonka Forest (52° 33′ N, 17° 06′ E), in west-central Poland. The two stands have been managed throughout their entire lifespan according to standards and policies obligatory for State Forests National Forest Holdings. This includes management practices such as cleaning and thinning. Forest management plans in Poland are designed to cover a 10-year period and are prepared by the Bureau of Forest Management and Geodesy (⇒ https://www.lasy.gov.pl/en/our-work/forest-management). The two stands were designated as research sites when the trees had reached maturity. All management operations are synchronized with research activities.
The forest site type for both stands has been classified as a fresh mixed broadleaved forest. The classification of the site conditions is based on the Polish forest typology system which utilizes distinctive features of the soil, forest floor, and stand. The two forest sites used in the study had similar soil, climate, and terrain conditions. Their productivity and utility in silviculture was also similar.
The stands were in a close vicinity (about 100 m) to each other and share the same soil type, i.e., rusty soils belonging to Arenosols ([18]). The tree basal area in the oak stand was 35, and 40 m2 ha-1 in the Scots pine stand (measured in 2015). The forest floor consisted mainly of Vaccinium myrtillus, Dryopteris filix-mas and Pleurozium schreberi in the Scots pine stand, and Carex digitata and Dryopteris filix-mas in the sessile oak stand. The undergrowth in the Scots pine stand was dense and completely covered the forest floor, while it was scattered in the oak stand with many bare-soil patches. The climate in this part of Poland is transitional between maritime and continental, with an average annual precipitation of 531 mm and a mean annual temperature of 8.5 °C.
Three soil pits, ~1 × 2 × 2 m (W × L × D) were dug up at the distance of 1-2 m from the trees to visually characterize the soil horizons. Soil horizons in the soil pits were designated as: organic (O), uppermost mineral (A), brunic (Bbr), illuvial (Bv), and bedrock (C). Soil samples were collected from each horizon (a total of 0.5 kg) in each pit for the analysis of soil texture and chemistry. Soil analyses were conducted by the Forest Management and Geodesy Agency in Poznan, Poland ([42]).
Root samples were simultaneously sampled from all pits. Each pit was surrounded by a few trees and the roots were collected from different sides of the pit, so roots encircled trees adjacent to soil pits, which resulted in a natural heterogeneity. Intact roots were collected from individual soil horizons and preliminarily separated from the soil by hand. Once collected, the roots were washed over sieves (pore size 1 mm) under tap water and cleaned with tweezers in a Petri dish to remove attached organic and mineral particles.
Healthy looking ectomycorrhizae were initially evaluated under a stereomicroscope (Olympus GmbH, Düsseldorf, Germany) and organized into morphological groups (morphotypes) based on macroscopic characteristics. Different morphotypes were then classified to the genus or species level based on ITS-barcoding (data not shown; see Fig.S3 in [42] for the results). A total of 52 root tips of Scleroderma and 23 root tips of Xerocomus morphotypes were used for molecular identification. Briefly, total genomic DNA was extracted from individual root tips possessing specific morphotypes using a NucleoSpin® Plant II kit (Macherey-Nagel GmbH & Co., Düren, Germany). The ITS1-5.8S-ITS2 region was amplified using a fungal-specific ITS1-F primer ([12]) and a universal ITS4 reverse primer ([44]). PCR products were purified and sequenced according to the manufacturer’s directions using a BigDye Terminator® v3.1 kit and an ABI Prism® 3130xl Genetic Analyzer (Applied Biosystems, USA). Sequence chromatographs were checked for accuracy in Chromas LITE v.2.01 (⇒ http://www.techenysium.com.au/) and aligned by the CodonCode Aligner (⇒ http://www.codoncode.com/aligner/) using a ≥97% identity threshold to group ectomycorrhizal sequences into operational taxonomic units (OTUs), which were subsequently used to query sequences in GenBank (⇒ https://blast.ncbi.nlm.nih.gov/Blast.cgi) and UNITE (⇒ https://unite.ut.ee/) databases. Sequences representing each OTU, including Xerocomus and Scleroderma, were deposited in GenBank under accession numbers KP731808-64.
After all of the collected ectomycorrhizal morphotypes were identified at the molecular level, ectomycorrhizal roots of Xerocomus in Scots pine and Scleroderma in sessile oak were specifically collected from the soil horizons of interest and used for C and N analyses. Molecular identification was not explicitly applied for these ectomycorrhizae as the samples were dried prior to C and N analyses.
Total nitrogen and carbon concentrations
Samples (approximately 500 mg) of fresh Xerocomus and Scleroderma ectomycorrhizal roots were collected from the soil horizons of interest in September 2013 and 2019. Depending on the size of ectomycorrhizae, samples comprised ca. 10 to 30 ECM root tips that were obtained from different root branches (to avoid root homogeneity). In total, 54 samples of Xerocomus and 70 samples of Scleroderma were analyzed. Samples were oven-dried (65 °C for 48 h), ground, and subsequently analyzed for total N and C with an elemental analyzer (model CHNS-O 4010, Costech Instruments, Italy/USA).
Data analysis
Two-way analysis of variance was used to determine the effect of soil horizon and year of harvest on levels of nitrogen (N%) and carbon (C%), and C:N ratios in ectomycorrhizae. The data on Xerocomus and Scleroderma ectomycorrhizae were analyzed separately. Due to a lack of normal distribution, data were log-transformed prior to analysis. Post-hoc Tukey-Kramer HSD comparisons were performed for all pairs. The analyses were performed in JMP® v.8.0 (SAS Institute Inc., Cary, NC, USA).
Results
Soil structure and chemistry
The soil chemical and structural parameters for each of the horizons in the soil pits were measured ([42]). The organic horizon in both sessile oak and Scots pine soil pits had the highest mineral status, exchange cations, and soil porosity, while low mineral concentrations, high density, base saturation, and pH were characteristic of the brunic horizon in both forest stands.
Molecular analysis
Analysis of the ITS data indicated that the Scleroderma and Xerocomus morphotypes were composed of four and three species, respectively. Three species of Scleroderma (S. areolatum, S. citrinum, and S. verrucosum) were detected in the studied soil horizons (Tab. 1). S. citrinum dominated in the organic horizon, S. areolatum in the uppermost mineral, and S. verrucosum in the brunic horizon. Xerocomus badius was abundant in all three of the studied soil horizons, while X. ferrugineus was only present in low abundance in the brunic horizon.
Tab. 1 - Mean (± standard error) abundance (%) of species of Scleroderma and Xerocomus ectomycorrhizae in the studied soil horizons. Means were calculated from the total number of ectomycorrhizal root tips collected in each horizon (n = 3). (a): Scleroderma bovista and Xerocomus cisalpinus were not found in the studied soil horizons, but were found in the other soil horizons (illuvial and bedrock).
Soil horizon | Quercus petraea | Pinus sylvestris | |||||
---|---|---|---|---|---|---|---|
Scleroderma
areolatum |
S. bovista a | S. citrinum | S. verrucosum |
Xerocomus
badius |
X. cisalpinus a | X. ferrugineus | |
Organic (O) | 7.0 ± 4.0 | 0.0 | 4.0 ± 3.6 | 0.0 | 51 ± 22 | 0.0 | 0.0 |
Mineral (A) | 0.8 ± 0.8 | 0.0 | 3.0 ± 2.0 | 0.0 | 43 ± 28 | 0.0 | 0.0 |
Brunic (Bbr) | 1.4 ± 0.2 | 0.0 | 4.0 ± 2.7 | 22.0 ± 22.0 | 0.0 | 0.0 | 34 ± 4 |
Concentrations of total nitrogen and carbon in the ectomycorrhizae
Mean nitrogen concentrations in Xerocomus ectomycorrhizae ranged from 2.2%-3.4% and from 2.8%-3.0% in Scleroderma ectomycorrhizae across all of the studied soil horizons (Tab. 2). The mean concentration of carbon in Xerocomus ectomycorrhizae ranged from 29%-46% and from 41%-44% in Scleroderma ectomycorrhizae across all of the studied soil horizons. Xerocomus ectomycorrhizae had a higher concentration of N (3.4%) in the fertile soil horizons (i.e., organic and uppermost mineral soil horizons) than in the infertile (brunic) soil horizon (2.2%). The concentration of N in Scleroderma ectomycorrhizae did not vary in the studied soil horizons. The concentration of carbon in both Xerocomus and Scleroderma ectomycorrhizae was significantly higher in the fertile horizons, relative to the brunic horizon. Surprisingly, the mean concentration of C was as low as 29% in Xerocomus ectomycorrhizae growing in the brunic horizon. Overall, the mean concentrations of N and C in Xerocomus were higher in ectomycorrhizae collected in 2019 than in those collected in 2013 (Tab. 3). In contrast, the concentration of carbon was higher in Scleroderma ectomycorrhizae collected in 2013 than in those collected in 2019, while no differences in mean carbon levels were observed for Xerocomus (data not shown).
Tab. 2 - Mean (± standard error) carbon (C) and nitrogen (N) concentration (%) and the C/N ratio in Xerocomus and Scleroderma ectomycorrhizae collected in the studied soil horizons: organic (O), uppermost mineral (A), and brunic (Bbr) across soil vertical profiles in Pinus sylvestris and Quercus petrea stands in the Zielonka Forest, west-central Poland. Means with different letters are significantly different.
EMF | Horizon | n | C % | N % | C/N |
---|---|---|---|---|---|
Xerocomus | O | 22 | 46 ± 0.2 a | 3.4 ± 0.1 a | 14 |
A | 18 | 45 ± 0.3 a | 3.4 ± 0.1 a | 14 | |
Bbr | 14 | 29 ± 2.0 b | 2.2 ± 0.1 b | 13 | |
Scleroderma | O | 32 | 44 ± 0.7 a | 2.8 ± 0.1 | 16 ab |
A | 19 | 43 ± 0.5 a | 2.8 ± 0.1 | 16 a | |
Bbr | 19 | 41 ± 0.9 b | 3.0 ± 0.1 | 14 b |
Tab. 3 - ANOVA of the effect of soil horizon (organic, uppermost mineral, and brunic) and harvest year (2013 and 2019) on the concentration of nitrogen and carbon and C/N ratio in Scleroderma and Xerocomus ectomycorrhizae collected in the studied soil horizons
EMF | Dependent variables | Independent variables | |||||
---|---|---|---|---|---|---|---|
Soil horizon (H) | Harvest year (Y) | H × Y | |||||
F | Prob | F | Prob | F | Prob | ||
Scleroderma | Nitrogen (%) | 0.8 | 0.5 | 1.3 | 0.3 | 0.9 | 0.4 |
Carbon (%) | 0.001 | < 0.0001 | 23 | < 0.0001 | 5.2 | 0.008 | |
C/N | 3.8 | 0.03 | 10 | 0.002 | 0.1 | 0.9 | |
Xerocomus | Nitrogen (%) | 4.1 | 0.05 | 43 | < 0.0001 | 14 | < 0.0001 |
Carbon (%) | 69 | <0.0001 | 13 | 0.0008 | 18 | < 0.0001 | |
C/N | 0.5 | 0.6 | 2.6 | 0.1 | 0.3 | 0.8 |
The C/N ratio of Xerocomus ectomycorrhizae did not vary among the soil horizons in Scots pine (Tab. 2, Tab. 3). The C/N ratio, however, was lower in Scleroderma ectomycorrhizae growing in the brunic (infertile) horizon of the sessile oak stand, relative to the Scleroderma ectomycorrhizae growing in the organic horizon.
Discussion
Contrary to our hypothesis, LDET ectomycorrhizae possess natural variations in N mobilization and/or uptake (expressed as N concentration) in response to soil fertility. As shown, Scleroderma is capable of absorbing N in infertile soil layers comparable to the N it absorbs in fertile soil horizons, while Xerocomus depends mostly on the fertile soil layer to obtain its N. It should be noted, however, that the Scleroderma and Xerocomus samples analyzed in our study may have contained more than one EMF species, which may also explain the inter-horizons variations that were observed. Although LDET ectomycorrhizae with higher mycelial biomass are more enriched in 15N than those with lower mycelial biomass ([13]), suggesting the existence of a fungal strategy for nitrogen acquisition that supports biomass accumulation, specific species of ectomycorrhizal fungi may be able to utilize nutrients from a broad range of soil resources using a variety of different processes including enzymes ([9]). Alterations in N and C levels in LDET ectomycorrhizae may also be a function, however, of varying levels of nutrients in the different nutrient pools being accessed by the fungi ([31]).
Forest soils possess diverse sources of nitrogen, mainly as a result of litter decomposition, including labile amino acids, amino sugars, nucleotides, and complex molecules like chitin and polypeptides ([9]). ECM fungi are capable of utilizing complex molecules through the secretion of proteases ([28], [36]) whose production can be inhibited by various soil factors, including high amounts of inorganic N ([22]). Inter- and intraspecific variation in the capacity to synthesize proteolytic enzymes ([28], [9]), as well as sensitivity to inorganic N loads ([23]), have also been reported to provide the opportunity for ECM fungi to differentially utilize N sources. Utilization of diverse N sources also varies among ECM exploration types ([22]), especially in those that form a hydrophobic medium-distance fringe exploration type, which are strongly sensitive to inorganic N deposition ([23]). In contrast, hydrophilic exploration types, including contact, short-distance, and medium-distance types of ectomycorrhizae, respond positively or exhibit a mixed response to N inorganic deposition. This is most likely due to a lower investment in their extramatrical hyphae ([23]). These authors also indicated that the variable response of hydrophobic long-distance exploration type fungi to inorganic N loads suggests that C allocation in this exploration type (which probably requires high amounts of C from their host) is not limited to inorganic N-sensitive taxa alone ([23]). In this regard, the response of Xerocomus and Scleroderma to soil fertility can be understood as interspecific variability in their preferences to N sources. Xerocomus may need to obtain N from soil organic matter decomposition (high N and C in fertile soil horizons), while Scleroderma can also utilize labile N compounds that are mostly in infertile soil horizons, possibly also via the weathering of minerals ([21]). To further explore this question, another study on diverse LDET ectomycorrhizae in relation with diverse forms of nitrogen (inorganic vs. organic) could be applied.
Ectomycorrhizal fungi are highly dependent on receiving carbon from their host ([27], [39]), even though some have some capacity to utilize carbon from soil organic matter ([15], [24]). It has been proposed that the high carbon cost associated with ectomycorrhizal fungi is due to their high nutrient uptake ([5]), which varies among ectomycorrhizal fungal species, as they differ in carbon sink strength ([4], [30]). It is also believed that long-distance-exploration type ectomycorrhizae are more effective in nutrient acquisition than other ectomycorrhizal exploration-types due to the extensive hyphae that they protrude out from the root surface. This mycelial extension is used to explore the surrounding soil for nutrients. The ability to acquire nutrients, however, can vary considerably between different species LDET ectomycorrhizae, independent of the biomass of mycelia produced ([4], [37]). Ectomycorrhizal species of fungi also utilize different nitrogen sources with varying degrees of efficiency ([10]), suggesting that nitrogen source may ([4]) or may not ([37]) impact the amount of carbon transferred to the ectomycorrhizal fungus. Ectomycorrhizal fungi can also retain and store nitrogen when N in the soil is limited ([13], [17]). Trees also allocate less carbon to ectomycorrhizal fungi when nitrogen levels are in excess ([16], [26]); suggesting that the level of soil N is a key factor regulating the function of ectomycorrhizae. Tedersoo et al. ([38]) reported a strong effect of ectomycorrhizal fungal lineage, rather than ectomycorrhizal exploration-type, on measured levels of stable nitrogen (15N). Although the level of enzymes in long-distance ectomycorrhizal exploration-types has been reported to be higher than in other exploration types ([38]), we suggest that an unknown mechanism may allow Scleroderma in sessile oak stands to acquire similar amounts of nitrogen in unfertile soil horizons as it does in more fertile soil horizons (e.g., organic layer). Whether the mechanism is related enzyme production, mineral weathering, or specific interactions among ECM fungi, is unknown. Additional experiment could help to explain the extent to which LDET ectomycorrhizae are capable of using natural N sources from a specific soil as well as the cost to the host for this investment.
Long-distance-exploration type ectomycorrhizae have been suggested to be best adapted to infertile soils, but variation within this exploration type exists. While N and C concentrations in Xerocomus were lowered significantly with a decrease in soil fertility along a soil vertical profile in a Scots pine stand, N and C concentrations in Scleroderma in a sessile oak stand were for the most part unaffected. This finding suggests that not all LDET ectomycorrhizae exhibit the same response to soil fertility.
Acknowledgments
LKT and BB designed the experiment, LKT, BB, AD, and JM collected the samples; LKT, AD, MF and JM analyzed the samples, LKT and AD analyzed data; LKT wrote the first version of the manuscript. All authors contributed to manuscript revisions.
This research was supported by a grant (2011/01/D/NZ8/01760) funded by the National Science Centre (Poland) to Lidia K. Trocha and the Institute of Dendrology of the Polish Academy of Sciences in Kórnik, Poland. The authors are grateful to Dr. Pawel Rutkowski for his support during soil analyses and root collection, Elzbieta Rudy for her help in the molecular analyses of ectomycorrhizal roots, and the employees of the Forest Experimental Station Murowana Goslina for information pertaining to the study site. The authors are also thankful to Dr. Dale Karlson for his irreplaceable advice in language correction and text edit.
The authors declare that they have no conflict of interest.
References
Gscholar
CrossRef | Gscholar
Gscholar
Authors’ Info
Authors’ Affiliation
Anna Durska
Department of Plant Ecology and Environmental Protection, Faculty of Biology, Adam Mickiewicz University, Uniwersytetu Poznanskiego 6, 61-614 Poznan (Poland)
Department of Silviculture, Faculty of Forestry and Wood Technology, Poznan University of Life Sciences, Wojska Polskiego 71E, 60-625 Poznan (Poland)
Department of Analytical and Environmental Chemistry, Faculty of Chemistry, Adam Mickiewicz University, Uniwersytetu Poznanskiego 8, 61-614 Poznan (Poland)
Institute of Dendrology, Polish Academy of Sciences, Parkowa 5, 62-035 Kórnik (Poland)
Corresponding author
Paper Info
Citation
Trocha LK, Bulaj B, Durska A, Frankowski M, Mucha J (2021). Not all long-distance-exploration types of ectomycorrhizae are the same: differential accumulation of nitrogen and carbon in Scleroderma and Xerocomus in response to variations in soil fertility. iForest 14: 48-52. - doi: 10.3832/ifor3594-013
Academic Editor
Daniela Baldantoni
Paper history
Received: Jul 21, 2020
Accepted: Nov 08, 2020
First online: Jan 18, 2021
Publication Date: Feb 28, 2021
Publication Time: 2.37 months
Copyright Information
© SISEF - The Italian Society of Silviculture and Forest Ecology 2021
Open Access
This article is distributed under the terms of the Creative Commons Attribution-Non Commercial 4.0 International (https://creativecommons.org/licenses/by-nc/4.0/), which permits unrestricted use, distribution, and reproduction in any medium, provided you give appropriate credit to the original author(s) and the source, provide a link to the Creative Commons license, and indicate if changes were made.
Web Metrics
Breakdown by View Type
Article Usage
Total Article Views: 34247
(from publication date up to now)
Breakdown by View Type
HTML Page Views: 29680
Abstract Page Views: 2113
PDF Downloads: 1959
Citation/Reference Downloads: 3
XML Downloads: 492
Web Metrics
Days since publication: 1607
Overall contacts: 34247
Avg. contacts per week: 149.18
Article Citations
Article citations are based on data periodically collected from the Clarivate Web of Science web site
(last update: Mar 2025)
Total number of cites (since 2021): 3
Average cites per year: 0.60
Publication Metrics
by Dimensions ©
Articles citing this article
List of the papers citing this article based on CrossRef Cited-by.
Related Contents
iForest Similar Articles
Research Articles
Effects of tree species, stand age and land-use change on soil carbon and nitrogen stock rates in northwestern Turkey
vol. 9, pp. 165-170 (online: 18 June 2015)
Research Articles
Nitrogen deposition and its impact on forest ecosystems in the Czech Republic - change in soil chemistry and ground vegetation
vol. 10, pp. 48-54 (online: 29 June 2016)
Research Articles
The effect of clear-cut age on soil organic carbon and nitrogen indices in Scots pine (Pinus sylvestris L.) stands
vol. 18, pp. 146-153 (online: 09 June 2025)
Research Articles
Response of soil bacterial communities to nitrogen and phosphorus additions in an age-sequence of subtropical forests
vol. 14, pp. 71-79 (online: 11 February 2021)
Research Articles
Effect of exogenous nitrogen and phosphorus inputs on the microbe-soil interaction in the secondary Castanopsis sclerophylla forest in east China
vol. 11, pp. 794-801 (online: 14 December 2018)
Research Articles
Soil stoichiometry modulates effects of shrub encroachment on soil carbon concentration and stock in a subalpine grassland
vol. 13, pp. 65-72 (online: 07 February 2020)
Research Articles
Growth performance and nitrogen use efficiency of two Populus hybrid clones (P. nigra × P. maximowiczii and P. trichocarpa × P. maximowiczii) in relation to soil depth in a young plantation
vol. 9, pp. 847-854 (online: 22 September 2016)
Research Articles
Controlling soil total nitrogen factors across shrublands in the Three Rivers Source Region of the Tibetan Plateau
vol. 13, pp. 559-565 (online: 29 November 2020)
Research Articles
Seasonal dynamics of soil respiration and nitrification in three subtropical plantations in southern China
vol. 9, pp. 813-821 (online: 29 May 2016)
Research Articles
Thinning effects on soil and microbial respiration in a coppice-originated Carpinus betulus L. stand in Turkey
vol. 9, pp. 783-790 (online: 29 May 2016)
iForest Database Search
Search By Author
Search By Keyword
Google Scholar Search
Citing Articles
Search By Author
Search By Keywords
PubMed Search
Search By Author
Search By Keyword