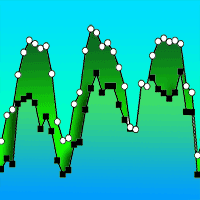
Analysis of canopy temperature depression between tropical rainforest and rubber plantation in Southwest China
iForest - Biogeosciences and Forestry, Volume 12, Issue 6, Pages 518-526 (2019)
doi: https://doi.org/10.3832/ifor3101-012
Published: Dec 09, 2019 - Copyright © 2019 SISEF
Research Articles
Abstract
Temperature change is an important environmental variable for global change sciences since it largely affects the physiology of plants in forest ecosystems. Canopy temperature depression (CTD) - the result of the deviation of the air temperature (Ta) from the plant canopy surface temperature (Tc) - varies depending on the meteorological and environmental conditions of the forests. Here, we evaluated the differences in CTD between a rubber plantation (RP) and a tropical rainforest (TR) in Xishuangbanna, southwestern China across the various time series of the period of 2011 to 2015. The mean maximum CTD values at the TR site and the RP site were 2.4°C and 0.6°C at diurnal level, 1.3°C and -0.5°C at monthly level, 0.6°C and -0.8°C at seasonal level and 5.6°C and 0.2°C at yearly time series level, respectively, while they were only significant (p < 0.01) in the diurnal time series. There was a significant (p < 0.01) negative linear relationship between CTD and global radiation (Q) in both sites at diurnal level and a significant (p < 0.05) negative linear relationship in the RP site at monthly time series level. A significant (p < 0.05) positive linear relationship between CTD and precipitation (P) at the RP site was found at diurnal level, as well as a significant (p < 0.01) positive linear relationship in the TR site at monthly time series level. The variation of CTD was critical for these two sites and largely depended on the amount of global radiation and the precipitation, while it will mainly affect the physiological variables. This study may prove useful for assessing the physiological response in terms of high temperature and drought conditions to regional and global change.
Keywords
Canopy Temperature Depression, Global Radiation, Precipitation, Tropical Rainforest, Rubber Plantation
Introduction
Global warming is gradually increasing and is consequently affecting forest ecosystems on an increasingly larger scale ([6]). It is expected that air temperatures will increase by between 0.8 °C and 1.0 °C by 2040-2050 and by between 2 °C and 4 °C by the end of the century ([34]). During this period, the increment rate of the average minimum temperatures will be larger than that of the average maximum temperatures, with the greatest chance for heat stress conditions occurring during the summer months ([13]). In fact, the annual mean temperature increased by 0.78°C in China during the period of 1906-2005 ([44]). Global radiation (Q) is the energy source for biological activities on the Earth and represents the energy exchange between the Earth’s surface and the atmosphere. In short, it is crucial for the development of weather and climate systems ([27]). During the 20th century, ground surface solar radiation was an important modulating factor in China’s surface air temperature changes ([41]), with both global and direct radiations decreasing over much of the nation ([28]). Indeed, solar radiation decreased by -1.50 Wm-2 10 yr-1 in China, which reduced the trends of the maximum surface and air temperature by about 0.139 °C 10 yr-1 and 0.053 °C 10 yr-1, respectively, during the period of 1906 to 2003 ([12]).
Precipitation (P) affects soil thermal inertia and the responses of surface vegetation, which, in turn, leads to regional and global warming ([36]). Precipitation can alter the surface absorbed energy into sensible heat flux and latent heat flux, which has an inevitable effect on both land surface and near-surface air temperatures ([49]). Changes in temperature and precipitation are the critical factors that affect plant development. Here, temperature largely affects plant growth responses directly, while it indirectly affects water use rates ([13]). Overall, temperatures are increasing on a global scale and this is expected to affect the growth of agronomic crops ([19]), while it is also associated with the increasing levels of greenhouse gases in the atmosphere.
Plant responses to climate change occur at both the leaf and the canopy level, especially in terms of responses ranging from photosynthesis to the transpiration rate of leaf expansion. Forest canopies are a critical interface between terrestrial Earth and the atmosphere. A canopy with a small number of leaves will exhibit a greater response to changing atmospheric demand ([34]), while a larger and denser canopy can create its own microclimate and will be less responsive to the atmospheric changes ([13]). Canopy surface temperature (Tc) represents the statistical mean of leaf temperature distribution ([14]) and has been shown to be highly correlated with climatic factors such as air temperature, relative humidity and long-wave radiation, as well as soil temperature at the shallowest depth ([21]). Above all, Tc serves as an indicator of plant water stress ([20], [16], [11]) and relates to both growing degree day ([22]) and yield ([10]). Tc has also been used in drought research ([29]), heat stress experiments ([3]) and forest carbon exchange studies ([21]). The leaf angle and leaf dimension have a large effect on Tc at the leaf level, while canopy height and structure largely affect the vegetation level. Meanwhile, Tc is directly proportional to air temperature (Ta) and global radiation (Q), but is inversely proportional to wind speed and relative humidity ([17]). Ta has been used to study the enzymatic and organismal response of forest canopies to the climatic and environmental changes ([45]), while it has also been shown to play an essential role in the physical processes of evapotranspiration, photosynthesis and heat transfer.
The difference between Ta and Tc is commonly used in the existing literature, with the difference distinguished according to their specific emphasis. The difference between Tc and Ta is known as “canopy temperature difference” (ΔT or CTdiff = Tc-Ta), while the deviation of the air temperature from the plant canopy temperature is known as “canopy temperature depression” (CTD, presented as Ta-Tc). When the canopy temperature falls below air temperature due to the evaporative cooling from transpiration, the CTD was found to be positive; conversely, when the canopy temperature rises above air temperature due to the closure of stomata, the CTD was found to be negative. Meanwhile, CTD has been found to be a good criterion for screening heat stress tolerance ([35]). In fact, CTD has been found to be particularly useful for assessing plant water status because it represents an overall, integrated physiological response to drought and high temperature ([3]). CTD also correlates with physiological traits such as stomatal conductance ([3], [33]), leaf chlorophyll ([31]), leaf water potential ([9]) and grain yield ([4], [23], [46]), both under water-stressed conditions ([24]) and heat- and drought-stressed conditions ([23], [32]).
China is a vast territory that has an abundance of climatic zones ranging from tropical to cold-temperate, while a special alpine climate is found in the Tibetan Plateau ([12]). Xishuangbanna represents a transitional area between the tropics and the sub-tropics, and is located at the northern edge of tropical southwestern Asia. Here, much of China’s 633.800 ha of old growth lowland tropical rainforests is found, with the region harbouring a great diversity of forestry. The area is considered to be particularly sensitive to the impacts of climate change, especially in terms of temperature and precipitation patterns. Indeed, climate records pertaining to Xishuangbanna that cover the last 40 years reveal a significant increase in air temperature. Meanwhile, in terms of the local economic aspects, the increasing demand for natural rubber has resulted in the conversion of numerous tropical rainforests into rubber plantations, which has, in turn, led to a significant reduction in biodiversity in the southwest region of China ([25]). Transforming natural forests into rubber plantations has significant ecological impacts on water balance, the carbon cycle, and biodiversity. Thus, this activity has seriously exacerbated the local climate change, largely in terms of inducing local surface warming and higher ranges of daily temperature. Therefore, the change in CTD due to the changing Tc and Ta of the two communities (rubber plantation and tropical rainforest) in response to climate change is likely to be variable.
The objectives of this study are as follows: (i) to analyse the diurnal, monthly, seasonal and yearly evolution of CTD in a tropical rainforest and a rubber plantation; (ii) to correlate canopy temperature depression (CTD) with the changes in global radiation (Q) and precipitation (P); and (iii) to evaluate and compare the differences in CTD between a tropical rainforest and a rubber plantation.
Material and methods
Study sites and species description
The autonomous prefecture of Xishuangbanna is situated in the southwestern Yunnan Province of China (Fig. 1). It borders Laos in the southeast and Myanmar in the southwest and presents a tropical and monsoon-influenced climate. In fact, the region has a typical monsoon climate, with an annual solar radiation of 116.72 Kcal cm-2 yr-1, and an annual sunshine average of 1858.7 h. Meanwhile, the mean annual temperature range is between 15.1 and 21.7 °C, while the annual precipitation is between 1200 and 2500 mm. During the dry season (from November to April) there is a frequent occurrence of heavy fog, while temperatures never drop below 15 °C, except for the very high elevation areas. Meanwhile, the precipitation during the rainy season (from May to October) accounts for more than 80% of the annual precipitation, with the highest precipitation occuring in July/August (average of 300 mm month-1), while the temperatures are high, with peaks around May and August/September ([37]).
The tropical rainforest study site (TR) is located at latitude 21° 55′ 39″ N, at longitude 101° 15′ 55″ E, at altitude of 750 m a.s.l. in the Menglun Nature Reserve, Xishuangbanna, southwestern China (Fig. 1). This means it lies at the northern edge of tropical southwestern Asia between the transition areas of the tropics and the sub-tropics. The region harbours uneven-age and complex stands with different heights and forms of tree, with the dominant tree species being Pometia tomentosa, Terminalia myriocarpa, Gironniera subaequalis, and Garuga floribunda, which can exceed 40 m in height. The main soil type is lateritic, which was derived from siliceous rocks, such as granite and gneiss, with a pH between 4.5 and 5.5.
The study area of Xishuangbanna represents the largest area of natural rubber production in China and has the largest number of rubber plantation areas. In recent years, rubber tree (Hevea brasiliensis) plantations have been replacing primary tropical rainforests with an alarming rate in China. The rubber plantation (RP) in this study is located in the experimental area of the Xishuangbanna Tropical Botanical Garden, situated at latitude 21° 55′ 30″ N, longitude 101° 15′ 59″ E and altitude 570 m a.s.l. (Fig. 1). Our observations covered a large 36-year-old rubber monoculture plantation, with mean canopy height of approximately 22 m.
The two sites (TR and RP) are located about 10 km apart and share similar climatic conditions. According to climatic observations made at a meteorological station over five decades (1959-2008), the mean annual temperature was 21.8 °C, while the mean annual rainfall was 1511 mm, with over 85% of the annual rainfall occurring in the rainy season; the mean monthly rainfall during the dry season is less than 40 mm ([40]).
Meteorological measurement
Air temperature was measured using temperature sensors (HMP45C®, Vaisala, Helsinki, Finland), installed at a height of 36 m above ground on the 72 m high observational tower at the TR site and 28.9 m above ground on the 55 m high observational tower at the RP site. Meanwhile, precipitation was measured using rain gauges (Rain Gauge 52203®, Young Co., Traverse City, MI, USA) installed at the top of the observational towers at both sites. Global radiation was measured using radiation sensors (CNR-1/CM11®, Kipp & Zonen, Delft, Netherlands) in terms of downward and upward and short- and long-wave radiation. The radiation sensors were installed at a height of 41 m above ground at the TR site and 28.6 m above ground at the RP site, using a horizontal pole fixed within three metres of the towers at both sites. All the meteorological data were collected using a CR1000® data logger (Campbell Scientific, Logan, UT, USA), with the time interval set at every 30 minutes at each site.
The canopy surface temperature was measured using infrared temperature sensors (SI-111® , Apogee Instruments, Inc., Logan, UT, USA). These were installed on the observational tower and were mounted on a 4cm-diameter galvanised metal pipe that was fixed three metres above each forest canopy at each site. The sensors were adjusted with half angles of approximately 22 °C from the nadir such that they were focused on the canopies. The absolute accuracy of the sensor is ±0. 2 °C. In fact, the instruments used here were similar to those used in the study by Song et al. ([40]), who found that the radiation detected using an infrared radiometer includes two components: the radiation directly emitted by the target surface, and the reflected background radiation (often neglected). The magnitude of the two components in the radiation detected by the radiometer was estimated using the emissivity and reflectivity of the target surface. However, due to machine error, the data related to canopy surface temperature for the period of January to March 2013 was missing.
Analysis methods
For both sites, the response of canopy temperature depression to global radiation and precipitation was evaluated through linear regression. One-way analysis of variance (ANOVA) was applied to assess the diurnal, monthly, seasonal and yearly differences in CTD ([8]). Using one-way ANOVA, the variation among five groups (from 2011 to 2015) was assessed based on a single independent variable, CTD, expressing means ± standard deviations. Correlation coefficients were calculated using Pearson’s bivariate correlation. A difference of p < 0.05 was considered as statistically significant between the groups. All statistical analyses were performed using SPSS® v. 22.0 statistical software (SPSS Inc., IBM, Armonk, NY, USA).
Results
Canopy temperature depression (CTD)
Diurnal and monthly changes of CTD
The mean diurnal and monthly changes of canopy temperature depression (CTD) at both sites across the entire measurement period of 2011 to 2015 are shown in Fig. 2. At both sites, the daytime canopy surface temperature (Tc) was higher than the air temperature (Ta), which resulted in negative values for CTD. With regard to the TR site, negative CTDs occurred during the daytime (10:00-17:00 h), while they began earlier and remained for a longer time at the RP site (2:00-18:00 h - Fig. 2a). The mean value of the monthly Ta was higher than the mean Tc, which resulted in positive values for CTD at the TR site, except for February. In contrast, negative values for CTD were found at the RP site, where the mean Tc value was higher than the mean Ta across the board (Fig. 2b).
Fig. 2 - Comparison of mean values of air temperature (Ta), canopy surface temperature (Tc) and canopy temperature depression (CTD) between the tropical rainforest (TR) and rubber plantation (RP) sites at the (a) diurnal and (b) monthly time series during 2011 and 2015.
Tc commonly followed a diurnal curve, with daytime temperatures rising due to increased temperatures with increasing global radiation ([11]). The mean diurnal temperature of Ta and Tc at the RP site was higher than that at the TR site, while the CTD at the RP site was lower than that at the TR site. Meanwhile, the mean diurnal CTD value was the highest at 20:00 h (2.4 °C) at the TR site and also at the RP site (0.6 °C - Fig. 2a). The value of the mean monthly temperature of Ta and Tc at the RP site was higher than that at the TR site, while the differences in CTD at the RP site were less significant than those at the TR site. The mean value of CTD was the highest in August (1.26 °C) at the TR site and in March (-0.54 °C) at the RP site (Fig. 2b).
The results of the ANOVA showed that Ta, Tc, and CTD at both sites had significant variation in terms of diurnal changes of temperature. However, non-significant variation of mean CTD values were found at both sites in terms of monthly changes, while mean Ta and Tc showed significant variation. The mean diurnal and the monthly minimum and maximum temperature values of Ta, Tc, and CTD are shown in Tab. 1.
Tab. 1 - Mean, minimum (min), and maximum (max) values in the diurnal and monthly time series of air temperature (Ta, °C), canopy surface temperature (Tc, °C), canopy temperature depression (CTD, °C), global radiation (Q, Wm-2) and precipitation (P, mm) in the Tropical Rainforest (TR) and Rubber plantation (RP) sites, with the matched standard deviaton (SD).
Time Series |
Climatic Factors |
TR (Mean ± SD) | RP (Mean ± SD) | ||||||
---|---|---|---|---|---|---|---|---|---|
Min | Max | Mean | Prob | Min | Max | Mean | Prob | ||
Diurnal | Ta | 17.5 ± 0.2 | 26.6 ± 0.5 | 20.7 ± 3.1 | <0.01 | 18.3 ± 0.2 | 27.8 ± 0.5 | 22.0 ± 3.4 | <0.01 |
Ts | 15.7 ± 1.4 | 29.9 ± 2.7 | 20.2 ± 5.3 | <0.01 | 18.5 ± 0.5 | 30.2 ± 1.3 | 22.8 ± 4.3 | <0.01 | |
CTD | -3.7 ± 2.8 | 2.4 ± 1.8 | 0.5 ± 2.8 | <0.01 | -3.5 ± 0.9 | 0.6 ± 0.8 | -0.8 ± 1.5 | <0.01 | |
Q | 19.3 ± 5.0 | 614.3 ± 13.6 | 295.4 ± 223.3 | <0.01 | 20.5 ± 6.0 | 581.4 ± 55.9 | 271.1 ± 209.8 | <0.01 | |
P | 0.04 ± 0.03 | 0.10 ± 0.03 | 0.06 ± 0.03 | 0.168 | 0.07 ± 0.05 | 0.19 ± 0.15 | 0.12 ± 0.08 | 0.818 | |
Monthly | Ta | 15.2 ± 0.8 | 24.1 ± 0.6 | 20.6 ± 3.3 | <0.01 | 16.6 ± 0.8 | 25.6 ± 0.4 | 22.1 ± 3.2 | <0.01 |
Ts | 14.9 ± 0.2 | 23.2 ± 2.6 | 20.3 ± 3.4 | <0.01 | 17.3 ± 0.4 | 26.3 ± 1.0 | 22.9 ± 3.3 | <0.01 | |
CTD | -0.7 ± 2.4 | 1.3 ± 3.0 | 0.5 ± 2.0 | 0.969 | -1.2 ± 0.6 | -0.5 ± 0.7 | -0.8 ± 0.7 | 0.985 | |
Q | 287.9 ± 36.7 | 429.2 ± 15.8 | 357.3 ± 49.3 | <0.01 | 239.2 ± 154.0 | 393.8 ± 30.2 | 308.8 ± 94.9 | 0.711 | |
P | 0.00 ± 0.01 | 0.17 ± 0.07 | 0.06 ± 0.07 | <0.01 | 0.02 ± 0.02 | 0.31 ± 0.15 | 0.13 ± 0.15 | <0.01 |
Seasonal changes of CTD
In all seasons, the mean values of Tc at the RP site were higher than the Ta values, which resulted in a negative CTD value, while the opposite was found at the TR site where the CTD value was positive. At both sites, the mean Ta, Tc and CTD values during the rainy season (from May to October) were generally higher than those of the dry season (from November to April - Fig. 3).
Fig. 3 - The variation of mean values of air temperature (Ta), canopy surface temperature (Tc) and canopy temperature depression (CTD) across different seasonal time series; dry season (from November to April), rainy season (from May to October) and annual between the tropical rainforest (TR) site with no color and rubber plantation (RP) site with gray color during 2011 and 2015.
As for the differences in seasonal change in temperature between Ta and Tc, the mean value at the RP site was higher than that at the TR site. The results of the ANOVA showed that the mean Ta and Tc at both sites showed significant variation in terms of seasonal changes in temperature. However, the variation of the mean CTD value was not significant at either site. The mean seasonal temperature values of Ta, Tc, and CTD are shown in Tab. 2.
Tab. 2 - Mean values (± standard deviation, SD) in seasonal time series of air temperature (Ta, °C), canopy surface temperature (Tc, °C), canopy temperature depression (CTD, °C), global radiation (Q, Wm-2) and precipitation (P, mm) at the tropical rainforest (TR) and rubber plantation (RP) sites.
Climatic Factors |
TR (Mean ± SD) | RP (Mean ± SD) | ||||||
---|---|---|---|---|---|---|---|---|
Dry | Rainy | Annual | Prob | Dry | Rainy | Annual | Prob | |
Ta | 18.1 ± 0.5 | 23.2 ± 0.4 | 20.6 ± 0.4 | <0.01 | 19.6 ± 0.5 | 24.6 ± 0.3 | 22.0 ± 0.4 | <0.01 |
Tc | 18.1 ± 1.4 | 22.3 ± 2.5 | 20.2 ± 1.8 | <0.05 | 20.4 ± 0.8 | 25.4 ± 1.0 | 22.8 ± 0.8 | <0.01 |
CTD | 0.1 ± 1.4 | 0.9 ± 2.3 | 0.6 ± 1.9 | 0.798 | -0.9 ± 0.7 | -0.8 ± 0.8 | -0.8 ± 0.7 | 0.989 |
Q | 360.4 ± 9.8 | 354.3 ± 7.7 | 365.2 ± 6.4 | 0.497 | 293.9 ± 73.0 | 313.6 ± 62.5 | 320.7 ± 18.1 | 0.789 |
P | 0.02 ± 0.01 | 0.11 ± 0.04 | 0.06 ± 0.02 | <0.01 | 0.06 ± 0.03 | 0.20 ± 0.11 | 0.12 ± 0.07 | <0.05 |
Yearly changes of CTD
At the TR site, most of the mean values of Ta were higher than those of Tc during the period of 2011 to 2013. Here, positive CTD values were found, while negative CTD values were found for the years of 2014 and 2015. Meanwhile, only negative CTD values were found at the RP site, with the exception of a number of months in 2011. The mean values of Ta and Tc at the RP site were higher than those at the TR site at all points of the year. The CTD variations at the TR site were fairly significant, while they gradually decreased later in the period. However, this was not so obvious at the RP site. The highest mean CTD values (5.6 °C at the TR site and 0.2 °C at the RP site) were found within the five-year period (Fig. 4).
Fig. 4 - Comparison of mean values of air temperature (Ta), canopy surface temperature (Tc) and canopy temperature depression (CTD) between the tropical rainforest (TR) and rubber plantation (RP) sites at the yearly time series during 2011 and 2015.
Relationship between CTD and Q in the diurnal and monthly time series
In terms of global radiation (Q), it was shown that the value decreased steadily to a minimum (almost zero) during the night hours, while it gradually increased from dawn and peaked around noon before it remained fairly constant throughout the evening for the five years under consideration. The diurnal time series of global radiation at our study sites showed a steady increase at 7:00, while peaks were observed between 13:00 and 16:00 at both sites before it steadily reduced to a minimum after 21:00 (see Fig. S1 in Supplementary material). We analysed the global radiation value range from 7:00 to 21:00. A negative correlation between CTD and Q was found at both sites in the diurnal time series (Tab. 3). Moreover, there was a significant negative linear relationship between CTD and Q at both sites, with the CTD decreasing as Q increases (Fig. 5a).
Tab. 3 - Pearson’s correlation coefficient (r) between mean values of global radiation (Q, Wm-2) and canopy temperature depression (CTD, °C), precipitation (P, mm) and canopy temperature depression (CTD, °C) for Tropical rainforest (TR) and Rubber plantation (RP) sites in different time series (diurnal and monthly) during 2011 and 2015. N = 14 in diurnal time series, N = 12 in monthly time series.
Time Series |
Climatic Factors |
TR | RP | ||
---|---|---|---|---|---|
r | p-value | r | p-value | ||
Diurnal | Q & CTD | -0.988 | <0.01 | -0.949 | <0.01 |
P & CTD | -0.148 | 0.489 | 0.478 | <0.05 | |
Monthly | Q & CTD | -0.086 | 0.791 | -0.613 | <0.05 |
P & CTD | 0.803 | <0.01 | 0.275 | 0.386 |
Fig. 5 - The relationship between mean values of global radiation (Q) and canopy temperature depression (CTD) in the tropical rainforest (TR) and rubber plantation (RP) sites at the (a) diurnal and (b) monthly time series during 2011 and 2015.
In the monthly time series, the CTD for the TR site had no correlation with Q, while for the RP site the CTD showed a negative correlation with Q (Tab. 3). Meanwhile, there was no significant relationship between CTD and Q at the TR site and a significant negative linear relationship between CTD and Q at the RP site, again, with the CTD decreasing as Q increases (Fig. 5b).
Relationship between CTD and P in the diurnal and monthly time series
In the diurnal time series, there was no correlation between CTD and P at the TR site, while we found a positive correlation at the RP site (Tab. 3). The CTD showed no significant relationship with P at the TR site and a significant positive linear relationship with P on the RP site, with the CTD increasing in accordance with the increasing P (Fig. 6a).
Fig. 6 - The relationship between the mean value of precipitation (P) and canopy temperature depression (CTD) in the tropical rainforest (TR) and rubber plantation (RP) sites at the (a) diurnal and (b) monthly time series during 2011 and 2015.
In the monthly time series, we found a positive correlation between CTD and P at the TR site, while no correlation was detected at the RP site (Tab. 3). There was a significant positive linear relationship between CTD and P at the TR site, with the CTD increasing in accordance with the increasing P, and no significant relationship at the RP site (Fig. 6b).
Discussion
Our results showed that the mean values of Ta and Tc at the RP site were higher than those at the TR site but that the CTD value of the RP site was lower than that of the TR site in the diurnal and monthly time series. In terms of the yearly time series, the same results were found, with the exception of the period between June and November of 2014. Song et al. ([40]) found in their one-year data analysis of the canopy temperature difference between Tc and Ta at these two sites that the differences at the RP site were higher than those of the TR site throughout the whole year. When we analysed the five-year data related to the canopy temperature depression between Tc and Ta, the same results were observed.
To a certain extent, the difference between Ta and Tc is related to plant water stress. The leaf temperature or the Tc of well-watered canopies is often 3-5 °C less than the Ta. As the available soil water decreases, leaf temperature or Tc are more closely tracked than Ta and, under extreme water stress, may exceed it ([13]). According to the results, a higher value of Tc than Ta was found at both sites in the diurnal time series from 11:00 to 18:00. Moreover, while similar results were found at the RP site in terms of the monthly and yearly time series, they were only found at the TR site for the years of 2014 and 2015. With regard to the relationship between Ta and Tc under plant water stress, the negative value of CTD represented a sign of an extreme water stress condition.
The canopy transpiration for the dominant tree species in the dry season was lower than that in the rainy season at both sites ([39]). At the leaf level, Tc related to physiological traits, where leaf angle and leaf dimension have an influence ([17]), while daytime CTD was associated with a morphological trait, specifically in terms of leaf size, where smaller leaf dimensions and thicker leaves resulted in greater daytime CTD ([5]). At the vegetation level, canopy height and structure affected the Tc ([17]). Song et al. ([40]) found that a normalised condition (Tc-Ta) was very sensitive to all simulated meteorological conditions that were linearly, directly proportional to solar radiation and inversely proportional with the vapor pressure deficit (VPD) and also non-linearly, inversely proportional to Ta and wind speed (Ws). Canopy height had a large influence on (Tc-Ta), in that it decreased with increasing height and increased in very low vegetation under drought stress ([17]). Furthermore, a decreasing temperature fluctuation was observed with increasing aboveground height ([42]). The temperature distribution pattern was also altered by the irrigation regime within individual crowns, while the intra-crown values depended on the onset of water stress ([16]). Since the TR site is composed of a varied canopy level of uneven-aged species, the fixed infrared sensor could not measure all the layers. In fact, we could only measure the actual leaf temperature of fully sun-exposed leaves in terms of achieving a mean canopy temperature. Thus, the value of the canopy temperature at the RP site was more precise than that of the TR site. In addition, an increase in CTD may have occurred due to increased respiration and a decreased transpiration resulting from stomatal closure ([38]). Indeed, in our results, the increment in the CTD values across all the time series at the TR site was higher than that of the RP site, which may have been a result of such increase in respiration and decrease in transpiration.
During the 20th century, the variations of incoming solar irradiance and ground surface solar radiation impacted China’s surface air temperatures ([41]). The surface and air temperatures were correlated with solar radiation used for energy to transfer heat in south China. Thus, the variations in solar radiation were the primary reason for the increasing temperature rates. Under high global radiation and drought conditions, the air temperature increased and soil moisture decreased, which in turn increased stomatal resistance. As a result, the Tc increased and the CTD decreased in mountain spruce stands and crops ([47], [32]). Moreover, in the absence of weather variability, Ta decreased due to longwave radiative cooling at night, while it increased due to solar radiation after sunrise. The values of global solar radiation were higher in the dry months (November to March) than in the wet months (April to October - [2]). At any given time and location, the amount of solar activity and the local meteorological conditions play a significant role in determining the received amount of solar radiation, resulting in a different pattern of diurnal variation from one season to another. When we focused on the daytime CTD, a significant negative linear relationship with Q was found in the diurnal time series at both sites. Further, in terms of the monthly time series, the CTD was not significantly related with Q at the TR site, though a significant negative linear relationship with Q at the RP site was detected. Thus, while in terms of the diurnal and monthly time series the global radiation rate was critical for the temperature variation at the RP site, this was only the case for the TR site in the diurnal time series.
Stand structure and topography are critical for the two studied sites as they share similar climatic conditions. However, the amount of global radiation was inversely proportional with latitude and directly proportional to altitude and to relative sunshine duration (RSD). In terms of the effect on global radiation, RSD had the greatest effect, while latitude came next and altitude had the least effect ([27]). In fact, in terms of altitude, our study revealed that the global radiation at the TR site was higher than that at the RP site across all the time series.
The moisture content of the topsoil in the monoculture rubber plantations was higher than that of the tropical forests ([26]). This is likely the result of the higher evapotranspiration and soil water withdrawal in the more densely vegetated areas of the tropical forests ([15]). Indeed, rubber can change the hydrological dynamics and can produce a drier, warmer climate ([50]). When the soil moisture is not adequate enough to meet the evaporative demands under high solar radiation and drought conditions, the stomatal conductance will decrease and Tc will increase ([46]). At both sites, the Tc values increased and the CTD values decreased during our study period. Overall, the Tc values at the RP site were higher than those at the TR site. This result indicated that the drought conditions had more of an effect on the RP site that on the TR site.
Precipitation was negatively correlated with temperature, had a lower effect than solar radiation ([12]) and reduced temperatures by increasing the surface evaporative cooling ([48]). A rubber tree is a (brevi-) deciduous tree that sheds leaves for a short two- to four-week period, but retains full foliage throughout the rest of the year ([18]). Meanwhile, rubber trees have been found to work as “water pumps” compared to rainforest vegetation ([43]), and consume more water than natural vegetation ([1]). Although rubber production is inversely correlated with rainfall, natural rubber thrives well in humid climates with well-distributed rainfall, where the rain must be evenly distributed throughout the year with no more than one dry month ([30]). However, the water consumption of rubber plantations was found to be greater than that of rainforests due to drought sensitivity ([43]).
Our results showed that in terms of correlation between CTD and precipitation, there was no significant relationship at the TR site and a significant positive relationship at the RP site in the diurnal time series. The rubber plantation, as a monoculture forest, consists of even-aged trees with similar canopy heights, while the tropical rainforest has a high diversity of tree species, including uneven-aged trees with different canopy heights. Thus, the two forests have different canopy structures. In addition, tropical rainforest roots are complex and can buffer the impact of diurnal rainfall, which means that the diurnal precipitation rate was not important for the tropical rainforest, but was important for the monoculture rubber plantation.
Tropical rainforests are evergreen (i.e., canopy density remains high throughout the year), thereby reducing the impact of seasonal drought through adaptive mechanisms such as leaf shedding and the utilisation of soil water reserves during periods of low rainfall ([7]). Overall, a rainforest creates a cool and moist environment that helps to reduce annual evapotranspiration ([43]). In our results, we found a positive significant linear relationship between CTD and P at the TR site and no significant relationship between CTD and P at the RP site in the monthly time series. Thus, the monthly precipitation rate of the TR site was more crucial than that of the RP site.
The Ta rate at both sites gradually increased according to the increasing Q during the later years, where the effect on Tc also increased. Meanwhile, the Tc rate was higher than the Ta rate during the later years, while negative values of CTD value were also found in our study, which indicated the occurrence of drought conditions. The variation of CTD is critical for these two sites and largely depended on the amount of global radiation and precipitation according to the time series interval. When drought stress occurs, plants close their stomata to avoid transpiration water losses. As a result, there is a decrease in transpiration cooling that causes an increase in canopy temperature. Meanwhile, the CTD variations affect the physiological variations and plant growth. Thus, the CTD was useful for assessing the physiological response to high temperature and drought conditions at the two study sites, especially in terms of transpiration rate and stomatal conductance.
Conclusions
The observations of CTD across the TR and RP sites correlated well with the measurements of Q and P, which paves the way for using such indicators as an alternative to assessing plant water status. The CTD reflects the canopy cooling capacity of the plants, where a negative value indicates the occurrence of extreme water stress. The significant relationship between CTD in the diurnal and monthly time series of the TR and RP sites confirms the correlation of CTD with Q and P reported in the literature. Global radiation was used in terms of selection criteria in assessing the temperature variations, while precipitation was used for reducing temperatures by increasing the surface evaporative cooling, which provided important feedback in terms of regional and global warming. The CTD at the TR site was higher than that of the RP site, which was evident from the greater differences in the Ta and Tc at the TR site than at the RP site. Given the differential behavior of both sites, it was important to avoid transpiration water losses when quantifying the level of drought stress. Hence, CTD appears to be one of the effective selection criterion for determining the tolerance to drought stress in these two sites. Moreover, this study pointed out the need to emphasise the water stress and drought effects, which would perhaps prove to be good aspects of focus in terms of future work.
Acknowledgements
This study was funded by the National Natural Science Foundation of China (NSFC - no. 31770528), the National Key Research and Development Program of China (2016 YFC0502105), and the Chinese Academy of Sciences 135 project (2017XTBG-T01; 2017XT BG-F01). We also thank the Central Laboratory and Biogeochemistry Laboratory of Xishuangbanna Tropical Botanical Garden, Chinese Academy of Sciences. We would like to acknowledge the staff and technicians of Xishuangbanna Station. Finally, STZM thanks the Myanma Timber Enterprise of Myanmar for their encouragements.
References
CrossRef | Gscholar
CrossRef | Gscholar
Gscholar
CrossRef | Gscholar
CrossRef | Gscholar
Supplementary Material
Authors’ Info
Authors’ Affiliation
Yiping Zhang
Qing-Hai Song
Xuehai Fei 0000-0002-1730-759X
Ruiwu Zhou
Youxing Lin
Liguo Zhou
Peng Zhang
CAS Key Laboratory of Tropical Forest Ecology, Xishuangbanna Tropical Botanical Garden, Chinese Academy of Sciences, Menglun 666303 (China)
Ruiwu Zhou
Youxing Lin
Liguo Zhou
Peng Zhang
University of Chinese Academy of Sciences, Beijing, 100049 (China)
Myanma Timber Enterprise, Ministry of Natural Resources and Environmental Conservation, Yangon, 11011 (Myanmar)
Xishuangbanna Station for Tropical Rainforest Ecosystem Studies, Chinese Academy of Sciences, Menglun 666303 (China)
Corresponding author
Paper Info
Citation
Tay Zar Myo S, Zhang Y, Song Q-H, Deng Y, Fei X, Zhou R, Lin Y, Zhou L, Zhang P (2019). Analysis of canopy temperature depression between tropical rainforest and rubber plantation in Southwest China. iForest 12: 518-526. - doi: 10.3832/ifor3101-012
Academic Editor
Claudia Cocozza
Paper history
Received: Mar 18, 2019
Accepted: Sep 10, 2019
First online: Dec 09, 2019
Publication Date: Dec 31, 2019
Publication Time: 3.00 months
Copyright Information
© SISEF - The Italian Society of Silviculture and Forest Ecology 2019
Open Access
This article is distributed under the terms of the Creative Commons Attribution-Non Commercial 4.0 International (https://creativecommons.org/licenses/by-nc/4.0/), which permits unrestricted use, distribution, and reproduction in any medium, provided you give appropriate credit to the original author(s) and the source, provide a link to the Creative Commons license, and indicate if changes were made.
Web Metrics
Breakdown by View Type
Article Usage
Total Article Views: 39736
(from publication date up to now)
Breakdown by View Type
HTML Page Views: 35339
Abstract Page Views: 2043
PDF Downloads: 1708
Citation/Reference Downloads: 10
XML Downloads: 636
Web Metrics
Days since publication: 1809
Overall contacts: 39736
Avg. contacts per week: 153.76
Article Citations
Article citations are based on data periodically collected from the Clarivate Web of Science web site
(last update: Feb 2023)
(No citations were found up to date. Please come back later)
Publication Metrics
by Dimensions ©
Articles citing this article
List of the papers citing this article based on CrossRef Cited-by.
Related Contents
iForest Similar Articles
Research Articles
Quantifying the vertical microclimate profile within a tropical seasonal rainforest, based on both ground- and canopy-referenced approaches
vol. 15, pp. 24-32 (online: 27 January 2022)
Research Articles
Canopy temperature variability in a tropical rainforest, subtropical evergreen forest, and savanna forest in Southwest China
vol. 10, pp. 611-617 (online: 17 May 2017)
Research Articles
Estimating changes in soil organic carbon storage due to land use changes using a modified calculation method
vol. 8, pp. 45-52 (online: 17 June 2014)
Research Articles
Do the rubber plantations in tropical China act as large carbon sinks?
vol. 7, pp. 42-47 (online: 21 October 2013)
Research Articles
Intra-annual tree growth responds to micrometeorological variability in the central Amazon
vol. 14, pp. 242-249 (online: 21 May 2021)
Research Articles
Determination of differences in temperature regimes on healthy and bark-beetle colonised spruce trees using a handheld thermal camera
vol. 14, pp. 203-211 (online: 02 May 2021)
Research Articles
Identification and characterization of gaps and roads in the Amazon rainforest with LiDAR data
vol. 17, pp. 229-235 (online: 03 August 2024)
Research Articles
Fine root production and distribution in the tropical rainforests of south-western Cameroon: effects of soil type and selective logging
vol. 3, pp. 130-136 (online: 27 September 2010)
Research Articles
Developing stand transpiration model relating canopy conductance to stand sapwood area in a Korean pine plantation
vol. 14, pp. 186-194 (online: 14 April 2021)
Research Articles
Strong relationships between soil and vegetation in reference ecosystems of a riparian Atlantic rainforest in the upper Doce River watershed, southeastern Brazil
vol. 16, pp. 226-233 (online: 17 August 2023)
iForest Database Search
Search By Author
Search By Keyword
Google Scholar Search
Citing Articles
Search By Author
Search By Keywords
PubMed Search
Search By Author
Search By Keyword