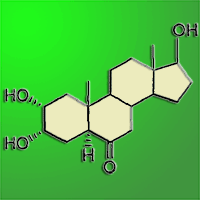
Effects of brassinosteroid application on seed germination of Norway spruce, Scots pine, Douglas fir and English oak
iForest - Biogeosciences and Forestry, Volume 10, Issue 1, Pages 121-127 (2016)
doi: https://doi.org/10.3832/ifor1578-009
Published: Oct 02, 2016 - Copyright © 2016 SISEF
Research Articles
Abstract
We tested the influence of a synthetically-produced brassinosteroid compound (2α,3α,17βtrihydroxy-5α-androstan-6-one) on seed germination in Norway spruce, Scots pine, Douglas fir and English oak. Before germination, 400 seeds of each species were steeped in a brassinosteroid solution and then placed for germination in a growth chamber under (i) optimal humidity and (ii) temporary drought stress (except for oak). Drought stress significantly reduced the germination capacity and germination rate in the control treatments of Norway spruce, Scots pine and Douglas fir. Nonetheless, the application of brassinosteroid significantly reduced the drought-stress effects in seeds of Norway spruce and Scots pine. The drought-stressed Douglas fir did not respond positively to the brassinosteroid application. English oak was germinated only under the optimal humidity regime and no differences in germination were detected between the control and brassinosteroid-treated acorns.
Keywords
Plant Hormones, Germination Percentage, Germination Rate, Picea abies, Pseudotsuga menziesii
Introduction
Rapid, synchronous full-capacity germination is an important component of commercial seedling production in forestry ([33]). Seeds germinated over a protracted period will generate seedlings with different requirements across the crop, thus the growing measures will be inevitably ill-suited for part of the seedlings ([16]). Germination problems in nursery may lead to a reduction in quality of the resulting plants and to an increase in length of the production cycle ([37]) or may even result in a loss of part of the nursery crop.
Seed germination problems are often related to adverse microclimatic conditions. Moisture availability after sowing is one of the most important factors affecting seed germination in the nursery ([37]). However, a full control of the soil moisture is not always possible (e.g., after direct seeding on a forest site or even in open outdoor nursery beds), thus drought stress may occur. Our hypothesis was that a pre-sowing application of chosen brassinosteroid compounds might improve germination parameters of seeds subjected to drought stress.
Brassinosteroids (BRs) are a group of plant hormones that are important regulators of a number of the plant physiological processes ([26], [6]). They can interact with other hormones as well as with environmental stimuli ([42]). The first BR was isolated in 1979 from rapeseed (Brassica napus L.) pollen. At that time, 40 mg of the active substance was extracted from 40 kg of pollen ([14]). The discovery of BRs promoted an intensive research on these plant hormones ([19]). Nowadays, around 70 different BR-compounds have been successfully isolated from plants, mostly in free form, but also conjugated to sugars and fatty acids ([5], [6]). BRs structure is closely related to animal steroid hormones ([8], [26]). They occur in many plant species ([42], [53]) and are present in various parts of the plant, such as pollen, seeds, leaves, flowers ([40]), roots ([24]) and also in insect galls ([51]). Their content is commonly higher in young and growing plant tissues than in older mature tissues ([8]).
BRs are able to influence plant growth and cell division even at very low concentrations ([20]), thus affecting growth performances. BRs are also able to promote plant resistance to pathogens and diseases ([48], [3]), as well as various stress factors ([35], [11]). Moreover, BRs can influence plants’ architecture, flowering and shedding of leaves ([15]). The positive effects of BRs on seed germination ([32], [23]) provided an important incentive to conduct this study.
Up to now, the applied research on BRs was conducted mainly in agriculture (see [19] for a review). Research aimed at evaluating the potential of these phytohormones in forestry are rather sporadic ([32]), and almost missing on European forest tree species.
A new BR-compound (2α,3α,17β-trihydroxy-5α-androstan-6-one) patented in 2003 ([25]) has a production cost markedly lower than that of many natural BRs. The aim of the paper is to assess the effects of this new BR-compound on seed germination of four commercially important forest tree species ([38], [39]). Its effects were investigated under conditions of optimal humidity and temporary stress induced by short-term drought during early germination.
Material and methods
Tab. 1 summarizes the main information on the seed lots investigated in this study, including the following species: Douglas fir (Pseudotsuga menziesii [Mirbel] Franco), Scots pine (Pinus sylvestris L.), Norway spruce (Picea abies [L.] Karst.) and English oak (Quercus robur L.). Prior to the launch of the experiment in April 2011, conifer seeds were stored in vacuum-sealed plastic bags at 2-3.5 °C in the dark. For coniferous species, no cold stratification was conducted before the start of the experiment. No detailed information was available on the acorn storage. Considering the year of ripening (2009), they were probably stored frozen at about -1 to -3 °C ([45], [17]). The acorns were supplied in moist sand. In the laboratory, the sand was washed off; the acorns were put into water and those floating were discarded. Immediately before the experiment, random samples of seeds were established for each species after hand-mixing of seeds; thus germination tests were conducted using pure seed lots.
Tab. 1 - Characteristics of the seed lots used in the present study. (1): Based on climatic characteristics of the forest vegetation (altitudinal) zones of the original seed sources; (2): western Bohemia. (n/a): not available.
Species | Douglas fir | Scots pine | Norway spruce | English oak |
---|---|---|---|---|
Code of seed source | SIA-473341 | CZ-3-3-BO-79-6-2-P | CZ-2-2B-SM-3735-6-3-P | CZ-1-2C-DB-388-6-3-P |
National seed lot code | CZ/9999/19/2011 | CZ/3206/6/2007 | CZ/3204/1/2010 | CZ/3201/1/2009 |
Seed ripened | 2009 | 2006 | 2009 | 2009 |
Seed source location | USA; Washington, Snoqualmie |
Czech Republic, WB2, Plzen |
Czech Republic, WB2, Plzen |
Czech Republic, WB2, Plzen |
Mean annual temperature (°C)1 | n/a | 7.5-8.0 | 6.5-7.5 | 6.5-7.5 |
Mean annual precipitation (mm)1 | n/a | 600-650 | 650-700 | 650-700 |
Weight of 1000 pure seeds (g) | 10.175 | 7.137 | 7.743 | n/a |
Number of pure live seeds per 1 kg | 86530 | 123580 | 97172 | n/a |
For each species, two different treatments were chosen: (i) the control; (ii) the brassinosteroid treatment. Except for English oak, seeds were subjected to two humidity regimes: (i) the optimal humidity regime (unstressed regime); and (ii) the drought-stressed regime (Tab. 2).
Tab. 2 - Design of the experiment. (Code): treatment-regime combination code.
Species | Treatment | Regime | Code | Seeds (n) |
Replications (n) |
---|---|---|---|---|---|
Douglas fir |
control | optimal humidity | CO | 400 | 8 |
drought-stressed | CS | 400 | 8 | ||
brassinosteroid | optimal humidity | BO | 400 | 8 | |
drought-stressed | BS | 400 | 8 | ||
Scots pine |
control | optimal humidity | CO | 400 | 8 |
drought-stressed | CS | 300 | 6 | ||
brassinosteroid | optimal humidity | BO | 400 | 8 | |
drought-stressed | BS | 200 | 5 | ||
Norway spruce | control | optimal humidity | CO | 400 | 8 |
drought-stressed | CS | 400 | 8 | ||
brassinosteroid | optimal humidity | BO | 400 | 8 | |
drought-stressed | BS | 400 | 8 | ||
English oak |
control | optimal humidity | CO | 400 | 4 |
brassinosteroid | optimal humidity | BO | 400 | 4 |
Before germination, the seeds were sterilized in sodium hypochlorite (1% solution) for 10 min and then thoroughly rinsed. For English oak, a third of each acorn was cut off at the cup scar end and the pericarp was removed in order to overcome seed dormancy ([7]), according to the Czech’s and International seed testing rules ([9], [21]). Subsequently, the BR-treated seeds were immersed into BR solution (0.1 mg l-1) for 48 hours at room temperature (20 °C). The brassinosteroid (2α,3α,17β-trihydroxy-5α-androstan-6-one) was synthesized by PHPchem Co. Ltd. (Neratovice, Czech Republic). Similarly, the control seeds were immersed into distilled water for 48 hours at 20 °C. Germination tests on conifer species were conducted in a growth chamber, while the oak acorns were tested in a thermostatic room. The conifer seeds were germinated on two layers of filter paper (50 g m-2) soaked by distilled water in transparent plastic vessels 6.5 × 9 × 3.5 cm. Acorns were placed about 2 cm deep inside a 4cm-thick layer of sterilized sand in small drained racks (40 × 30 × 10 cm) that were lined with propylene water-permeable mulch (50 g m-2) at the bottom and on the sidewalls. As for Douglas fir, Scots pine, and Norway spruce, the germination process consisted of 21 one-day cycles (24 hrs), composed of dark periods of 16 hours at 20 °C and light periods of 8 hours at 30 °C. Light at about 3000 lux was provided by means of cool white fluorescent tubes. The acorns (English oak) were germinated only in darkness at 20 °C in the moist sand for 28 days.
In the (unstressed) optimal humidity regime applied to conifer species, the relative air humidity ranged between 90-92% inside the testing vessels (Tab. 2). The seeds were placed on water-soaked filter paper and the vessels were cupped with lids. Contrastingly, vessels in the drought-stressed regime were left uncapped and exposed to conditions with 58-62% relative air humidity for 48 hours (two one-day cycles) at the beginning of the germination. Subsequently, the filter paper was re-soaked and the drought-stressed seeds were sprayed with distilled water; vessels were then cupped and the regime was shifted to that of the optimal humidity. English oak seeds were excluded from the drought-stressed regime. The acorns were placed in moist sand (about 12-15% of humidity by weight), which avoided any temporary drought.
To achieve uniformity of conditions during the course of the experiment, the germination process of all tested species was ran simultaneously. The full experimental design including the numbers of seeds and replications for each treatment-regime combination is described in Tab. 2. Each replication was placed in a separate vessel (conifers) or rack (English oak).
For the tested conifers, germinated seeds were counted on the 7th, 14th, and 21st day after the start of germination. For oaks, an additional count was taken on the 28th day. The duration of the drought-stressing regime (48 hours) in the stressed treatments was included in the total duration of germination.
Conifer seeds were considered completely germinated when their radicle was four times the length of the seed coat and no abnormalities were apparent. Acorns were scored as germinated when both radicle and shoot exhibited growth and leaves started to develop. The germinated seeds were removed from the vessels/ racks. For the purpose of this study, the germination capacity of filled seeds was defined as the percentage of seeds that had germinated during the test (i.e., over a period of 21 and 28 days for conifers and oak, respectively). The germination energy of filled seeds was defined as the percentage of the completely germinated seeds recorded on the 7th day after the beginning of the experiment. The numbers of empty and dead seeds were determined after the test completion by cutting open the seeds that failed to germinate.
To compare the percentage of germinated seeds between the treatment-regime combinations, the test of homogeneity of binomial distributions was applied by multiple comparisons (α = 0.05). Differences in the relative germination frequency among treatments were tested using the formula described by Agresti et al. ([1]), which was originally used for calculating confidence intervals of differences in probabilities. Using this approach, the null hypothesis about equality of two compared binomial proportions is rejected when (eqn. 1):
where Q
T(α
) is the critical value of Studentized range distribution for the chosen significance level and an infinite number of degrees of freedom, while pÌÂ
i and pÌÂ
j are the probabilities of success (germination) in the compared treatment-regime combinations, which were computed as follows (eqn. 2):
where y
i and y
j are the numbers of successes (completely germinated seeds), n
i and n
j are the numbers of observations (number of all seeds within a treatment-regime combination that were subjected to the germination test minus number of empty seeds within the respective treatment-regime combination) and se
was calculated as (eqn. 3):
Seed germination rate (GR
) was calculated for each species as the sum of values obtained by dividing the weekly percentages of completely germinated filled seeds by the number of days the seeds had been left in the growth chamber, using the following formula (adapted from [34] - eqn. 4):
where n
is the week of counting, GP
n is the percentage of completely germinated filled seeds in the n
-th week, Δt
n is the number of days elapsed from the beginning of the experiment (7, 14, 21 days, and 28 for oak only).
The Kruskal-Wallis test (α = 0.05) was applied to test for differences in germination rates among treatment-regime combinations in Norway spruce, as the data were not normally distributed. Two-way analysis of variance was used to compare germination rates of Scots pine and Douglas fir, where the first factor was the treatment (control vs. brassinosteroid) and the second one was regime (optimal vs. drought-stressed). For English oak, the Student’s t-test was applied (α = 0.05) to test for differences between treatments.
Results
Norway spruce
The germination of untreated Norway spruce seeds was significantly affected by the exposure to the drought stress (Fig. 1). The results also showed a markedly positive effect of brassinosteroids on germination under drought-stressing conditions. At the end of the germination process, seeds under drought-stress treated with the brassinosteroid (BS combination) showed almost four-times higher germination (cumulative germination percentage on the 21st day) than the control seeds exposed to drought stress (CS combinations). The application of the brassinosteroid also significantly increased the germination capacity under optimal humidity regime.
Fig. 1 - Cumulative percentage of germinated Norway spruce seeds in the control and brassinosteroid treatment recorded 7, 14, and 21 days after the beginning of germination under optimal humidity or drought-stressing regime. Error bars represent 95% confidence intervals. Different letters indicate significant differences (p < 0.05) between the means.
Similar results were achieved for the germination rate (Fig. 2). The highest germination rate (3.4) was recorded in the BO treatment-regime combination, while the lowest (0.6) was recorded in the control subjected to drought stress (CS). These two treatment-regime combinations differed significantly. The germination rates recorded in the CO and BS combinations were similar (2.7 and 2.6, respectively) and did not differ either from BO or CS combinations.
Fig. 2 - Growth rates calculated from the germination tests carried out for a period of 21 days in conifers and 28 days in English oak. The statistical procedures applied and the p-values obtained are reported for each species. (T): treatment; (R): regime; (TR): T×R interaction. Different letters following mean values denote significant differences (p < 0.05) after the multiple comparisons of treatment-regime combinations within each species. Error bars represent the standard deviation.
Scots pine
Germination of untreated Scots pine seeds was significantly reduced by the exposure to the drought stress (Fig. 3). The application of the brassinosteroid completely counterbalanced such effect, keeping the germination percentage of stressed brassinosteroid-treated seeds (BS) similar to that observed for untreated control seeds under optimal humidity regime (CO). Interestingly, and in contrast to Norway spruce, pine seeds treated with the brassinosteroid under optimal humidity regime (BO) showed significantly lower germination capacity (by 12%) than the control seeds germinated under optimal humidity regime (CO).
Fig. 3 - Cumulative percentage of germinated Scots pine seeds in the control and brassinosteroid treatment recorded 7, 14, and 21 days after the beginning of germination under optimal humidity or drought-stressing regime. Error bars represent 95% confidence intervals. Different letters after the values indicate significant differences between the means (p < 0.05).
The germination rate of Scots pine seed lots was significantly affected both by the treatment (p = 0.011) and the regime (p = 0.004), as well as by their interaction (p < 0.001). The germination rate of the BR-treated seeds under optimal humidity regime (BO) was reduced in comparison with the control seeds germinated under optimal humidity regime (CO). Under drought stress, however, the applied BR significantly expedited seed germination. The germination rate of the drought-stressed BR-treated seeds (BS) was almost comparable to the germination rate recorded in the CO, whereas the germination rate of the control seeds under drought stress (CS) was more than 6 times lower than that of the control seeds incubated under optimal humidity regime (CO).
Douglas fir
The impact of drought stress on Douglas fir germination was obvious and significant (Fig. 4). However, it was markedly less pronounced than in the case of Norway spruce and Scots pine (Fig. 1, Fig. 3, Fig. 4).
Fig. 4 - Cumulative percentage of germinated Douglas fir seeds in the control and brassinosteroid treatment recorded 7, 14, and 21 days after the beginning of germination either under optimal humidity or drought-stressing regime. Error bars represent 95% confidence intervals. Different letters indicate significant differences between the means (p < 0.05).
The bar chart in Fig. 2 suggests that the effect of BR application on Douglas fir germination rate was rather inhibitory (regardless of the humidity regime considered), though not significant (p = 0.11). On the other hand, the drought stress significantly inhibited the germination rate (p < 0.001) in Douglas fir. No interaction between the treatment and regime was found (p = 0.86). The highest germination rate was detected for the control seeds germinated under optimal humidity regime (CO). The BO, CS and BS treatment-regime combinations showed lower values than CO (100%) by 13.8%, 31% and 41.4%, respectively.
English oak
English oak was incubated only under the optimal humidity regime. The application of brassinosteroid did not affect the germination of acorns. The proportion of germinated seeds in the control and BR treatment remained almost equal in all the four counting dates (Fig. 5). Moreover, the germination rate of acorns (Fig. 2) did not differ between the control and BR treatment (p = 0.94).
Fig. 5 - Cumulative percentage of germinated English oak seeds in the control and brassinosteroid treatment recorded 7, 14, 21 and 28 days after the beginning of germination under the optimal humidity regime. Error bars represent 95% confidence intervals. Different letters indicate significant differences (p < 0.05) between the means after Student’s t-test.
Discussion
We tested the effects of 2α,3α,17β-trihydroxy-5α-androstan-6-one on seed germination of three native and one introduced forest tree species commonly used in Central-European forest management. The effects of the brassinosteroid application on seed germination (except for oak) were examined in combination with temporary drought stress, as could often occur after direct seeding at a forest site or in small forest nurseries with limited access to artificial irrigation.
A positive effects of BRs on seed germination has been reported for various species in the literature. For example, Leubner-Metzger ([29]) suggested that BR treatment promoted growth potential of embryos in tobacco (Nicotiana tabacum L.) seeds. Treating barley (Hordeum vulgare L.) seeds with BRs accelerated germination and development of primary and seminal roots ([23]) as well as growth of juvenile seedlings in the greenhouse and growth chamber ([13]). A stimulating effect of BRs on seed germination was also documented in Arabidopsis Heinh. by Steber & McCourt ([44]), who proposed that BRs play a role in antagonizing seed dormancy. Yamaguchi et al. ([50]) reported that brassinosteroids improved germination of aged rice (Oryza sativa L.) seeds and seedlings emergence. Srivastava et al. ([43]) described a positive effect of BRs on germination of moong bean (Phaseolus aureus Rox.).
As regards with the effects of BRs on plants under stressing conditions, Wilen et al. ([49]) found only a slight increase in freeze tolerance (by 3-5 °C) of smooth bromegrass (Bromus inermis Leyss) cell cultures treated with BRs, but cell viability after exposure to high temperature stress was markedly enhanced by the treatment. Dhaubhadel et al. ([10]) reported that oilseed rape (Brassica napus) and tomato (Solanum lycopersicum) seedlings grown in the presence of 24epibrassinolide were significantly more tolerant to a lethal heat treatment than the control seedlings grown in the absence of the BR compound. Vardhini & Rao ([46]) reported that brassinosteroids were able to reverse inhibitory effect of osmotic stress induced by 20% Polyethylene Glycol (simulating drought stress) on germination of sorghum (Sorghum vulgare Pers.) seeds. The application of brassinosteroids to rice (Oryza sativa L.) seeds reduced the impact of salt stress in the initial period after germination ([4]). The addition of BR significantly alleviated the negative impacts of NaCl on germination of cucumber (Cucumis sativus L.) seeds ([47]). Drought-stress tolerance of wheat (Triticum aestivum L.) induced by brassinosteroids was reported by Sairam ([41]). Despite the stressing conditions mentioned above are not directly comparable, and the methods used in testing seed germination often differ among studies, it has been suggested that drought, high temperature and salinity stresses are often interconnected and may induce similar cellular damage ([6]).
The effect of BRs application on seeds of forest tree species has been scarcely studied. Li et al. ([30]) investigated the effect of BR treatments on one-year-old seedlings of black locust (Robinia pseudoacacia L.). Their results suggest that the BR treatment could reduce water stress and enhance drought tolerance. They achieved the best results using a solution with BR concentration of 0.2 mg l-1. Li et al. ([32]) stated that soaking the seeds in a brassinolide (a natural brassinosteroid) solution (0.4 mg l-1) for 2 days improved the germination rate (GR
) and germination energy (GE
) of tree-of-heaven (Ailanthus altissima [Mill.] Swingle) by 17.6 and 18.7%, respectively. According to the study by Li et al. ([31]), GR
and GE
increased by 23.1% and 14.9%, respectively, using a solution of natural brassinolide at 0.05 mg l-1 in Chinese red pine (Pinus tabulaeformis Carr.), while in black locust (Robinia pseudoacacia L.) the use of a solution at 0.01 mg l-1 increased GR
and GE
by 10.9% and 15.9%, respectively.
In this study, we tested 0.1 mg l-1 concentration of the BR compound on seeds of four forest tree species. The results differed significantly depending on the species and humidity regime. For Norway spruce and Scots pine, its effects under the optimal humidity regime were less distinct than under drought stress. More specifically, differences in germination capacity were found also under optimal humidity, indicating a favorable effect of the BR treatment in Norway spruce, but a detrimental effect in the case of Scots pine. However, under drought stress, the effect of BR treatment in comparison with the control were decidedly more evident. For both Norway spruce and Scots pine, the effect of BR treatment under drought stress were positive in terms of both germination capacity (Fig. 1, Fig. 3) and germination rate (Fig. 2). For Scots pine, such effect was slightly negative under optimal humidity whilst definitively positive under drought stress (Fig. 3, Fig. 2). As for English oak seeds, the germination of the control and BR-treated seeds did not differ under optimal humidity (Fig. 5, Fig. 2). In general, these results are in accordance with the findings by Krishna ([26]) who suggested that the favorable effect of brassinosteroids on plant germination and growth is more obvious under stress and less evident under optimal conditions.
As regards Douglas fir, seed germination seems to be affected more by the humidity regime than by the BR treatment. Indeed, a slightly negative response to the BR treatment was found for this species, though this was not statistically supported. Nonetheless, the germination rate of the BR-treated seeds was detectably lower in comparison to the control, regardless of the humidity regime (Fig. 2). Similarly, the BR treatment of Douglas fir showed a noticeably lower (though not significant) germination capacity under drought stress than the control (Fig. 4). In this context, it is worth to notice that Douglas fir was the least drought-affected species among all those considered.
The concentration of BR in the solution used for soaking the seeds could partly explain the different responses observed in this study among the species considered. Indeed, seeds of different species may react differently to similar BR concentrations. Germination of Ailanthus altissima was most effectively promoted with 0.4 mg l-1 BR concentration ([32]), while Robinia pseudoacacia and Pinus tabulaeformis showed the best germination at 0.1 and 0.05 mg l-1, respectively ([31]), and a BR concentration as low as 0.01 nM (~ 0.005 mg l-1) was most effective to improve germination and seedling growth of tomato under phenanthrene stress ([2]). Furthermore, the different history of the different seed lots used in this study (in terms of age, storage conditions, etc.) could also have played a role in the different responses among species to the BR treatment.
The low germination of unstressed Norway spruce seeds in our study was probably the result of dormancy after storage, as such species usually show better germination ([36], [17]). This assumption is supported by the relatively high percentage of viable ungerminated seeds at the end of the germination test (47% and 33% for the CO and BO treatment-regime combinations, respectively). The cold stratification (moist chilling) might have increased the germination capacity ([52]). Moreover, the illumination intensity during the experiment could also have played a role in determining the low germination observed for Norway spruce seeds ([28]). However, this does not impair the results of the comparison between the BR-treated and control Norway spruce seeds, as seeds in both treatments were sampled from the same seed lot and were subjected to the same humidity regimes.
The germination capacity of the tested (unstressed and BR-untreated) Scots pine seeds showed values similar to those reported in other studies from Central Europe ([22], [17]). However, the cold stratification of seeds (moist chilling) might increase the germination capacity of the species ([27]). As for Douglas fir, the germination capacity and the germination energy of unstratified seeds recorded in this study correspond to data presented by Houšková & Martiník ([18]). The low germination rate of unstratified Douglas fir seeds has been well documented ([12]). Our results suggest that the BR treatment cannot substitute the cold stratification treatment in conifer species ([17], [52], [18]). The lower germination capacity of English oak seeds might have been the result of worse quality of the seed lot used, as well as of the duration and regime of storage.
In general, our study suggests that the BR application can reduce the impact of drought stress on seed germination of Norway spruce and Scots pine. However, these preliminary findings should be verified on a large number of seed lots. Cold stratification (moist chilling) might be desirable to achieve better physiological homogeneity of seed lots, although this pre-treatment is not prescribed for Norway spruce and Scots pine by Czech technical rules ([9]) before seed testing.
Conclusions
Drought stress significantly reduced germination capacity and germination rate of the tested seed lots of Norway spruce, Scots pine and Douglas fir. The application of BR in our study was effective in counteracting the drought-stress effects on the tested seeds in Norway spruce and Scots pine. However, Douglas fir drought-stressed seeds did not respond positively to the BR treatment. English oak was not subjected to drought-stress, and the germination of the control- and BR-treated acorns did not differ under optimal humidity. Under optimal humidity regime, the effect of BR treatment was positive only in the case of Norway spruce.
Acknowledgements
This study was supported by the Czech University of Life Sciences Prague (Project No. CIGA 20124304), and the Ministry of Agriculture of the Czech Republic (Project No. QJ1220331). The experiment was conducted in the Seed testing laboratory of the Department of Silviculture at the Truba Research Station (Faculty of Forestry and Wood Science, Czech University of Life Sciences Prague). We thank Jitka Šišáková (Translation and Editing Services, Roztoky u Prahy, Czech Republic), Andrew Leslie (National School of Forestry, Cumbria, UK) and Dr. W. Keith Moser (USDA Forest Service, Rocky Mountain Research Station, USA) for proofreading the manuscript.
References
Gscholar
Gscholar
Gscholar
Gscholar
Gscholar
Gscholar
Authors’ Info
Authors’ Affiliation
Martin Baláš
Rostislav Linda
Josef Gallo
Olga Nováková
Faculty of Forestry and Wood Sciences, Czech University of Life Sciences Prague, Kamýcká 129, 165 21 Praha 6 - Suchdol (Czech Republic)
Corresponding author
Paper Info
Citation
Kuneš I, Baláš M, Linda R, Gallo J, Nováková O (2016). Effects of brassinosteroid application on seed germination of Norway spruce, Scots pine, Douglas fir and English oak. iForest 10: 121-127. - doi: 10.3832/ifor1578-009
Academic Editor
Gianfranco Minotta
Paper history
Received: Jan 28, 2015
Accepted: Jun 05, 2016
First online: Oct 02, 2016
Publication Date: Feb 28, 2017
Publication Time: 3.97 months
Copyright Information
© SISEF - The Italian Society of Silviculture and Forest Ecology 2016
Open Access
This article is distributed under the terms of the Creative Commons Attribution-Non Commercial 4.0 International (https://creativecommons.org/licenses/by-nc/4.0/), which permits unrestricted use, distribution, and reproduction in any medium, provided you give appropriate credit to the original author(s) and the source, provide a link to the Creative Commons license, and indicate if changes were made.
Web Metrics
Breakdown by View Type
Article Usage
Total Article Views: 51586
(from publication date up to now)
Breakdown by View Type
HTML Page Views: 43542
Abstract Page Views: 2865
PDF Downloads: 3899
Citation/Reference Downloads: 77
XML Downloads: 1203
Web Metrics
Days since publication: 3149
Overall contacts: 51586
Avg. contacts per week: 114.67
Article Citations
Article citations are based on data periodically collected from the Clarivate Web of Science web site
(last update: Mar 2025)
Total number of cites (since 2017): 15
Average cites per year: 1.67
Publication Metrics
by Dimensions ©
Articles citing this article
List of the papers citing this article based on CrossRef Cited-by.
Related Contents
iForest Similar Articles
Research Articles
Influence of mother plant and scarification agents on seed germination rate and vigor in Retama sphaerocarpa L. (Boissier)
vol. 7, pp. 306-312 (online: 08 April 2014)
Technical Reports
Effects of different mechanical treatments on Quercus variabilis, Q. wutaishanica and Q. robur acorn germination
vol. 8, pp. 728-734 (online: 05 May 2015)
Research Articles
Effects of different nut pretreatments and substrates on germination and seedlings growth of Neocarya macrophylla Sabine in Basse Casamance, Senegal
vol. 17, pp. 346-352 (online: 03 November 2024)
Research Articles
Conservation of Betula oycoviensis, an endangered rare taxon, using vegetative propagation methods
vol. 13, pp. 107-113 (online: 23 March 2020)
Research Articles
Germination and seedling growth of holm oak (Quercus ilex L.): effects of provenance, temperature, and radicle pruning
vol. 7, pp. 103-109 (online: 18 December 2013)
Research Articles
Use of brassinosteroids to overcome unfavourable climatic effects on seed germination in Pinus nigra J. F. Arnold
vol. 17, pp. 1-9 (online: 02 February 2024)
Research Articles
Role of serotiny on Pinus pinaster Aiton germination and its relation to mother plant age and fire severity
vol. 12, pp. 491-497 (online: 02 November 2019)
Research Articles
Optimum light transmittance for seed germination and early seedling recruitment of Pinus koraiensis: implications for natural regeneration
vol. 8, pp. 853-859 (online: 22 May 2015)
Research Articles
The effectiveness of short-term microwave irradiation on the process of seed extraction from Scots pine cones (Pinus sylvestris L.)
vol. 13, pp. 73-79 (online: 13 February 2020)
Research Articles
Substrates and nutrient addition rates affect morphology and physiology of Pinus leiophylla seedlings in the nursery stage
vol. 10, pp. 115-120 (online: 02 October 2016)
iForest Database Search
Search By Author
Search By Keyword
Google Scholar Search
Citing Articles
Search By Author
Search By Keywords
PubMed Search
Search By Author
Search By Keyword