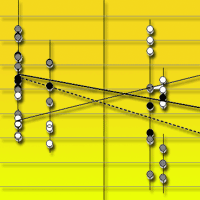
The effects of post-pasture woody plant colonization on soil and aboveground litter carbon and nitrogen along a bioclimatic transect
iForest - Biogeosciences and Forestry, Volume 6, Issue 5, Pages 238-246 (2013)
doi: https://doi.org/10.3832/ifor0811-006
Published: Jun 13, 2013 - Copyright © 2013 SISEF
Research Articles
Abstract
We investigated the effects of woody plant colonization of abandoned pastures on soil and litter organic carbon (C) stocks and nitrogen (N) content along a bioclimatic transect in a semi-arid environment (Sicily, Italy). Soil samples were taken in three successional stages (grazed pasture, shrubland, forest) within each of three bioclimates (supramediterranean - “supra”, mesomediterranean - “meso”, thermomediterranean - “thermo”). Organic C and N in litter and soil (0-10 cm and 10-30 cm depth) were determined, as well as soil bulk density. Especially at 0-10 cm depth, changes in C and N contents along successional stages differed among bioclimates. Soil organic carbon (SOC) stock decreased from pasture to shrubland and increased from shrubland to forest in “supra”, increased from pasture to shrubland and then remained stable in “thermo”, and was stable in “meso”. Soil C/N ratio decreased with succession in “supra”, showed no significant trend in “meso”, and increased with succession in “thermo”. Litter C stock increased with succession in “meso”, increased from pasture to shrubland and decreased from shrubland to forest in “thermo”, and increased from pasture to shrubland and then remained stable in “supra”. Litter C/N ratio increased in “thermo” and “supra” from pasture to shrubland and from shrubland to forest, but did not change significantly with succession in “meso”. The different trends in SOC among bioclimates may be caused by changes in the importance of litter input, litter decay rate and mineralization. Successional changes in “meso” and “supra” appeared to be most affected by litter quality, while those in “thermo” appeared to be strongly influenced by limited litter decay due to low soil moisture and high temperature.
Keywords
Introduction
After oceans, soils represent the largest carbon (C) reservoir on Earth. To a depth of 1 m, soils contain 1550 Gt of organic C and 950 Gt of inorganic C, more than the sum of C stored in terrestrial vegetation (560 Gt) and the atmosphere (760 Gt - [37], [38]). The size of the soil organic carbon (SOC) pool reflects C inputs of plant-derived organic matter residues and C losses resulting from mineralization (as carbon dioxide), leaching of dissolved organic C, and erosion. Vegetation, precipitation, and temperature determine a steady state level of C content for each soil ([25], [46], [13]), but this equilibrium between input and output can be modified by land-use change. In the last decades, one of the most important types of land-use change, particularly in the mid-latitudes of the northern hemisphere, has been the abandonment of agricultural lands ([17]). In general, the abandonment of cultivated or grazed lands results in colonization by woody plants (secondary succession). The species composition of successional plant communities varies along with macro- and mesoclimate and other abiotic and biotic factors ([57]). In the Mediterranean area, the recent abandonment of marginal agricultural areas (pasture and/or arable land) has caused an increase in the area occupied by pre-forest and forest communities ([6]).
In the context of the Kyoto Protocol, research is needed to understand the process of C sequestration in soil. Areas subject to secondary succession are of particular interest because they might have great potential as C sinks ([2]). Soil C dynamics after agricultural abandonment have been quantified in many studies ([33], [12], [56], [35]), and the spread of woody plants into grasslands/pastures/croplands is generally thought to increase the C stored in these ecosystems ([49], [36], [43], [2]). Some authors, however, have reported that secondary succession causes a reduction in soil C ([24], [25], [29], [46], [1]). It follows that further studies are required on factors that determine whether SOC increases or decreases after agricultural abandonment. One of these factors may be climate ([29]). In a study in Italy, for example, changes in SOC after agricultural abandonment were significantly related to annual rainfall ([2]).
C and N dynamics in soils of abandoned fields are also likely to be affected by plant community composition. The main way the vegetation influences C and N input into soils is through litter deposition ([34], [10], [19]). Many factors including temperature, moisture, pH, and other abiotic environmental conditions determine the quantity and quality of litter C and N inputs ([42], [48]) and the composition and abundance of the decomposer community ([54], [3], [10], [39], [18]). All these factors change with time-since-abandonment because plant community composition and soil characteristics are in continuous transformation during succession ([40], [23]).
Litter quality, often measured as C/N ratio or lignin content, is frequently used to indicate the transformability of litter to humified organic matter. Regarding secondary succession, Montané et al. ([44]) found that decomposability of aboveground litter in a Mediterranean mountain area was higher for grasslands than shrublands. The same authors concluded that a decrease in the decomposition rates of the aboveground litter was the main cause for a net increase in C sequestration upon shrub encroachment into grasslands. These observations are strongly linked to the activity of soil decomposer communities because there are close interactions and feedback between litter quality, plant community composition, and soil microbial activity and diversity ([39]).
In this paper, we investigate the effects of woody plant colonization after grazing cessation on soil and aboveground litter C and N content along a bioclimatic transect in a semi-arid environment. Bioclimatic classifications were used to subdivide the investigated territory into areas sharing homogeneous conditions for the growth of plant communities. By using a bioclimatic transect, we could directly relate variations in C and N contents to temperature and rainfall regimes, and indirectly relate these variations to plant community composition. We attempted to determine whether soil C and N storage are affected by stage of succession and bioclimate. To interpret our results, we also collected data on the changes of litter quality and mass during succession and among bioclimates. We focused on two main questions:
- Is there an overall influence of bioclimate on soil and litter C and N content?
- Do soil and litter C and N dynamics during succession differ among bioclimates?
Materials and Methods
Study site
The study site was located in the Madonie Mts., a calcareous massif of northern Sicily (Italy - Fig. 1), where large areas of marginal agricultural land were abandoned during the second half of the 20th century. Because of their elevation gradient, ranging from sea level to 1979 m a.s.l. (Pizzo Carbonara), the Madonie Mts. have a variety of bioclimatic conditions. To characterize local bioclimate, we used data reported by Drago et al. ([15]). These authors developed an accurate geostatistical model of Sicilian bioclimatic belts by interpolating data collected over 30 years by thermo-pluviometric stations all across the island. An overview of the potential vegetation of Sicily ([4]) recently confirmed this interpretation of the climatic data.
Fig. 1 - Location of the study site within the Italian territory (asterisk in inset) and of the experimental areas (circles) within the study site. Successional stages: (P): pasture; (S): shrubland; (F): forest. Bioclimatic thermotype is represented by a grey scale: (dark grey): supramediterranean; (medium grey): mesomediterranean; (light grey): thermomediterranean. Grey lines delimit ombrotype: with decreasing altitude, the ombrotypes are upper humid, lower humid, upper subhumid, lower subhumid, and upper arid (climatic data after Drago et al. 2002).
To characterize local bioclimates, RivasMartínez’s ([51]) indexes were used: the ombrotype is based on the mean annual precipitation, while the thermotype is based on the temperature regime as indicated by the thermal index It, which is calculated as follows (eqn. 1):
where T
is the mean annual temperature (°C); M
is the mean of the maximum temperatures of the coldest month (°C), and m
is the mean of the minimum temperatures of the coldest month (°C).
Our analysis showed that the study site is characterized by a combination of three thermotypes (supra-, meso-, and thermo-mediterranean) and five ombrotypes (upper humid, lower humid, upper subhumid, lower subhumid, and upper arid). We then selected three experimental areas (Fig. 1) within each of the three most representative bioclimates, i.e., within the “supramediterranean-lower humid” (henceforth: “supra”), “mesomediterranean-lower humid” (“meso”), and “thermomediterranean-lower subhumid” (“thermo”) belts (Tab. 1). In each experimental area, three successional stages representing a (post-) pasture succession series were selected: (1) grazed pasture without woody species; (2) shrubland; and (3) forest. Successional stages in each experimental area were located in direct proximity to each other and characterized by comparable abiotic conditions (aspect, slope, soil type, rock outcrop, stone cover, etc). Soil homogeneity among the three experimental areas within the same bioclimate was checked visually.
Tab. 1 - Environmental features of the experimental areas. Abbreviations: (supra): Supramediterranean-Lower Humid; (meso): Mesomediterranean-Lower Humid; (thermo): Thermomediterranean-Lower Subhumid; (T): mean annual temperature; (R): mean annual rainfall; (UTM): UTM coordinates of the areas; (Alt): altitude. Climatic data after Drago et al. ([15]); soil data after Carta dei suoli della Sicilia (Unpublished, Ass. Reg. Ris. Agr. e Al., Regione Siciliana, Palermo, Italy); soil classification after WRB ([58]).
Bioclimate | T (°C) |
R (mm) |
Soil | UTM | Alt (m a.s.l.) |
---|---|---|---|---|---|
Supra | 11 | 1100 | Haplic Luvisols Profondic |
417040 E / 4190155 N | 1.354 |
417577 E / 4190123 N | 1.296 | ||||
420185 E / 4191819 N | 1.222 | ||||
Meso | 14 | 1100 | Endoleptic Regosols Eutric |
412104 E / 4195084 N | 1.050 |
411782 E / 4197410 N | 723 | ||||
410396 E / 4198116 N | 674 | ||||
Thermo | 17 | 650 | Endoleptic Regosols Eutric |
404329 E / 4201567 N | 295 |
402264 E / 4203765 N | 177 | ||||
402902 E / 4203630 N | 175 |
Plant community composition differed depending on successional stage and bioclimate (Tab. 2). All sampled successional stages showed vegetation cover values of 95 ± 5% (mean ± standard deviation), with a maximum of 5% cover of rock outcrop and stones. Pastures were dominated by grasses and perennial herbs (Tab. 2), with woody cover < 5%. Shrublands were dominated by Rosaceae in “supra”, by Rosaceae and Spartium junceum L. in “meso”, and by sclerophyllous shrubs (Rhamnus alaternus L., Pistacia lentiscus L.) and Spartium junceum in “thermo”. In all shrublands, shrubs represented a woody cover of 65 ± 15%, while the remaining part was covered by annual and perennial herbs and grasses. Forests were dominated by beech in “supra”, by downy oak and holm oak in “meso”, and by downy oak in “thermo”. Tree cover in all bioclimates was 90 ± 5%, with intermediate values of understorey shrub (30 ± 15%) and herb (20 ± 10%) cover. According to Bazan et al. ([4]) and considering the differences between the three bioclimatic belts, potential vegetation of the selected experimental areas was ascribed to the following associations: (a) “supra”: Ilici aquifolii-Quercetum austrotyrrhenicae Brullo & Marcenò in Brullo 1984 and Anemono apenninae-Fagetum sylvaticae (Gentile 1969) Brullo 1984 em. Ubaldi et al. 1987, both referred to the alliance Doronico-Fagion (Gentile 1969) Ubaldi et al. 1990; (b) “meso”: Aceri campestris-Quercetum ilicis Brullo 1984 and Rhamno alaterni-Quercetum ilicis Brullo & Marcenò 1985, both belonging to the alliance Quercion ilicis Br.-Bl. ex Molinier 1934 em. Rivas-Martínez 1975; and (c) “thermo”: Oleo sylvestris-Quercetum virgilianae Brullo 1984 (Quercion ilicis).
Tab. 2 - Dominant plant species (in alphabetical order) in each successional stage of the studied bioclimates (information from the three replications per successional stage was grouped). Abbreviations for bioclimates are explained in Tab. 1.
Bioclimate | Successional stage |
Dominant plant species |
---|---|---|
Supra | Pasture | Carduus pycnocephalus L., Carlina nebrodensis DC., Centaurea solstitialis L. subsp. schouwii (DC.) Dostál, Eryngium amethystinum L., Juncus effusus L., Lolium perenne L., Prangos ferulacea (L.) Lindl., Schedonorus arundinaceus (Schreb.) Dumort. |
Shrubland | Astracantha nebrodensis (Guss.) Greuter, Crataegus laevigata (Poir.) DC., Euphorbia rigida M. Bieb., Prunus spinosa L., Pyrus communis L., Rosa canina L., Rubus canescens DC. | |
Forest | Euphorbia meuselii Raimondo & Mazzola, Fagus sylvatica L., Hedera helix L., Ilex aquifolium L., Quercus petraea (Mattuschka) Liebl. subsp. austrotyrrhenica Brullo, Guarino & Siracusa | |
Meso | Pasture | Bromus hordeaceus L., Calamintha nepeta (L.) Savi, Centaurea calcitrapa L., Convolvulus arvensis L., Eryngium campestre L., Euphorbia rigida M. Bieb., Ferula communis L., Foeniculum vulgare L., Lolium perenne L., Onopordum illyricum L., Thapsia garganica L. |
Shrubland | Daphne gnidium L., Crataegus laevigata (Poir.) DC., Pyrus amygdaliformis Vill., Rosa canina L., Rubus ulmifolius Schott, Spartium junceum L. | |
Forest | Acer campestre L., Quercus ilex L., Quercus virgiliana Ten. | |
Thermo | Pasture | Ampelodesmos mauritanica (Poir.) T. Dur. & Schinz, Arundo collina Ten., Carlina sicula Ten., Dasypyrum villosum (L.) Borbás, Dittrichia viscosa (L.) Greuter, Foeniculum vulgare L., Galactites tomentosa (L.) Moench |
Shrubland | Asparagus acutifolius L., Pistacia lentiscus L., Pyrus amygdaliformis Vill., Rhamnus alaternus L., Spartium junceum L. | |
Forest | Olea europaea L. var. sylvestris (Mill.) Lehr., Quercus virgiliana (Ten.) Ten. |
Abandonment age of the sampled successional stages was determined by evaluating aerial photographs taken in 1955 (produced by Istituto Geografico Militare, Florence, Italy) and 1987 (Regione Siciliana, Palermo, Italy). All sampled forests had been abandoned for more than 50 years, while all sampled shrublands had been abandoned for 25-50 years. Areas that were sampled as forests in 2011 had, in 1955, 15 ± 5%, 20 ± 5%, and 10 ± 5% tree cover and 75 ± 20%, 50 ± 10%, and 45 ± 40% shrub cover in “supra”, “meso”, and “thermo”, respectively. The same areas in 1987 were characterized by 70 ± 35%, 90 ± 10%, and 50 ± 30% tree cover and 30 ± 25%, 10 ± 5%, and 40 ± 15% shrub cover, respectively. All areas that were sampled as shrublands in 2011 were grazed pastures (woody cover < 5%) in 1955, and in 1987 had 25 ± 20%, 35 ± 5%, and 25 ± 5% shrub cover and no tree cover in “supra”, “meso”, and “thermo”, respectively.
Soil sampling and analysis
Soil was sampled according to the protocol of the Italian National Inventory of Forests and Forest Carbon Pools ([22]). Litter on soil was collected within a 30 × 30 cm square, and litter mass was expressed as t ha-1. After the litter was removed, mineral soil samples were collected at depths of 0-10 cm and 10-30 cm using a 340 and a 680 cm3 cylinder, respectively. In each successional stage, three soil samples (replications) were collected at approximately 10-m intervals along a linear transect (3 bioclimates × 3 experimental areas × 3 succession stages × 2 depths × 3 replications = 162 soil samples). In shrublands, soil samples were collected under the canopies of randomly selected shrub individuals.
Litter and soil samples were stored in a bag and immediately send to the laboratory. Litter samples were oven dried (55 °C), weighed, and then analysed for C and N content.
Soil samples were gently broken, passed through a 2-mm sieve, air dried, ground to a fine powder, treated with HCl 2:1 to remove carbonates ([28]), and then analysed for C and N content using a CHN-Elemental Analyzer. SOC content was first expressed as a percentage (g of C per 100 g of dry soil × 100) and then converted to Mg per hectare based on bulk density (BD) and soil depth using the following equation (eqn. 2):
where BD
is bulk density (g cm-3), C
conc is carbon concentration (g/100 g), D
is depth thickness (m), and CF
coarse is a correction factor [1- (gravel % + stone %) /100].
Soil bulk density was measured using the tube core method ([5]) and was based on the volume of the collected sample and the weight of dry soil in the sample.
Data evaluation
We used ANOVAs to compare the effects of bioclimate (supra-, meso-, and thermomediterranean), successional stage (pasture, shrubland, and forest), soil depth (0-10 cm and 10-30 cm), and their interactions on SOC stock, BD, soil N content, soil C/N, aboveground litter C content, aboveground litter N content, and aboveground litter C/N ratio. Because no statistical differences were found among the same successional stage within each bioclimate (data not shown), stage data were pooled within bioclimates and considered as replicates, thus obtaining 9 replications per successional stage (3 experimental areas × 3 replications). ANOVAs were also used to test for statistical differences among successional stages within each bioclimate. Means were compared with an adjusted Tukey’s least significant difference (LSD) test. In addition, regression analyses were performed to determine the significance of relationships among altitude (m a.s.l.) and soil characteristics (SOC, N, C/N ratio) or litter C/N ratio.
We also computed an average index of SOC content change (CCI
, Carbon Change Index) from early to late successional stages, respectively, as follows (eqn. 3):
where C
older is the SOC content at late successional stages, and C
early is the SOC content at early successional stages. A regression analysis was then performed to determine whether CCI
was related to altitude. A similar analysis was performed for N content (nitrogen change index, NCI
).
All analyses were carried out using the SAS statistical package (SAS Institute 2001).
Results
Overall differences among bioclimates
All measured soil and litter parameters differed among bioclimates (Tab. 3). SOC and soil N increased from “thermo” to “supra” at both soil depths (Fig. 2). C stock and N content changed significantly with soil depth (Tab. 3): the 0-10 cm depth contained more C and N than the 10-30 cm depth (Fig. 2). Litter C/N ratio was lower in “supra” than in “meso” and “thermo”, and mean soil C/N ratio was lower in “supra” and “meso” than in “thermo” (Tab. 4, Fig. 3).
Tab. 3 - Effects of bioclimate, successional stage, and soil depth as indicated by P values from ANOVAs. The factorial analysis included main effects and interactions of bioclimate (supra-, meso-, and thermomediterranean), successional stage (pasture, shrubland, and forest), and soil depth (0-10 cm and 10-30 cm). (SOC): soil organic carbon stock; (BD): bulk density; (Soil N): soil nitrogen content; (Soil C/N): ratio of carbon and nitrogen in soil; (Litter C): litter carbon stock; (Litter N): litter nitrogen stock; (Litter C/N): ratio of carbon and nitrogen in litter.
Source of variance |
Dependent variable and P values | ||||||
---|---|---|---|---|---|---|---|
SOC | BD | Soil N | Soil C/N | Litter C | Litter N | Litter C/N | |
Replication | 0.3101 | 0.3258 | 0.0123 | 0.1412 | 0.0111 | 0.3271 | 0.1211 |
Bioclimate (B) | <0.0001 | 0.0002 | <0.0001 | <0.0001 | 0.0008 | 0.0003 | 0.0087 |
Succession (S) | 0.3075 | <0.0001 | 0.0017 | 0.2475 | <0.0001 | 0.0023 | 0.00043 |
Depth (D) | <0.0001 | <0.0001 | <0.0001 | 0.2629 | - | - | - |
B*S | 0.0147 | 0.0934 | 0.0145 | 0.8186 | 0.0031 | <0.0001 | 0.0065 |
B*D | 0.093 | 0.1407 | <.0001 | 0.0001 | - | - | - |
S*D | 0.1877 | 0.7563 | 0.0283 | 0.4919 | - | - | - |
B*S*D | 0.0542 | 0.9618 | 0.1066 | 0.0453 | - | - | - |
Fig. 2 - Soil organic carbon stock (C - above) and soil nitrogen content (N - below) along an altitudinal gradient across all successional stages (pasture, shrubland, forest) at two soil depths (0-10 cm: empty circles; 10-30 cm: full circles). The gradient included three bioclimates, which are indicated by the vertical lines. Each point represents the mean of nine replicate soil samples taken in every successional stage, and bars represent standard deviation.
Tab. 4 - Mean values of soil (0-10 cm depth) and litter parameters for the three bioclimates and three successional stages. Means in columns with different uppercase or lowercase letters are statistically different at P < 0.01: lowercase letters are used to compare successional stages within a bioclimate, and uppercase letters to compare the overall means of bioclimates; (ns): not significant; (Litter): Litter mass. Other abbreviations as in Tab. 3.
Bioclimate | Successional stage | SOC (t ha-1) |
BD (g cm-3) |
Soil N (g kg-1) |
Soil C/N |
Litter (t ha-1) |
Litter C (t ha-1) |
Litter N (t ha-1) |
Litter C/N |
---|---|---|---|---|---|---|---|---|---|
Supra | Pasture | 42.84 | 1.22 | 2.54 | 15.72 | 2.56 | 0.64 | 0.02 | 19.2 |
Shrubland | 32.30 | 1.10 | 3.03 | 14.22 | 6.17 | 4.56 | 0.99 | 23.3 | |
Forest | 37.41 | 1.05 | 3.50 | 13.02 | 9.29 | 3.22 | 0.43 | 25.1 | |
Mean | 37.30 | 1.13 | 2.97 | 14.48 | 5.59 | 2.76 | 0.48 | 22.2 | |
Meso | Pasture | 34.59 | 1.21 | 2.40 | 12.53 | 4.38 | 1.64 | 0.09 | 29.7 |
Shrubland | 34.20 | 1.17 | 2.05 | 15.61 | 8.38 | 3.36 | 0.38 | 29.7 | |
Forest | 34.86 | 1.12 | 2.84 | 11.87 | 6.66 | 4.59 | 0.82 | 29.8 | |
Mean | 34.58 | 1.17 | 2.41 | 13.29 | 6.22 | 3.40 | 0.49 | 30.5 | |
Thermo | Pasture | 20.72 | 1.35 | 0.58 | 30.03 | 7.41 | 2.59 | 0.21 | 34.0 |
Shrubland | 24.99 | 1.23 | 0.49 | 43.34 | 9.75 | 4.23 | 0.51 | 41.7 | |
Forest | 22.11 | 1.06 | 0.58 | 46.68 | 9.06 | 3.80 | 0.41 | 51.0 | |
Mean | 22.67 | 1.23 | 0.55 | 38.81 | 8.70 | 3.51 | 0.37 | 41.9 |
Fig. 3 - Soil carbon stock at 0-10 cm soil depth (empty circles, solid regression line), litter C/N ratio (full black circles, thick solid regression line), and soil C/N ratio (full grey circles, dashed regression line) along an altitudinal gradient. The gradient included three bioclimates, which are indicated by the vertical lines. Each point represents a mean of three replicate soil samples taken in every successional stage, and bars represent standard deviation.
Differences among successional stages within bioclimates
Only the 0-10 cm soil depth showed significant differences for the measured parameters among successional stages within bioclimates. The CCI
showed a significant trend only for the 0-10 cm depth, with negative values in “supra”, values near zero in “meso”, and positive values in “thermo” (Fig. 4). The NCI
did not show any significant trend.
Fig. 4 - Carbon change index along an altitudinal gradient at 0-10 cm soil depth. The gradient included three bioclimates, which are indicated by the vertical lines. Each point represents a mean of three replications, and bars represent standard deviation.
At 0-10 cm soil depth, secondary succession in “supra” led to a decrease in SOC from pasture to shrubland and then to an increase from shrubland to forest (Tab. 4). Interestingly, SOC values were lower for forests than pastures in “supra”. In “meso”, however, SOC did not vary with secondary succession, while in “thermo”, SOC values increased from pasture to shrubland but did not significantly change from shrubland to forest. “Thermo” forests had the lowest SOC values of all the considered forest communities.
Soil N, on the other hand, increased with succession in “supra”, while in “meso” and “thermo” it decreased from pastures to shrubland and increased from shrubland to forest. Soil N was about 6-7 times lower in “thermo” forests than in “supra” ones.
Soil C/N ratio decreased with succession in “supra”, but showed no significant trend in “meso” and tended to increase with succession in “thermo”. Soil C/N ratio values were similar for “supra” and “meso” but were more than two times greater in “thermo” than in “supra” and “meso”.
C stocks in litter increased significantly with succession in “meso”, while in “thermo” litter C increased from pasture to shrubland and decreased from shrubland to forest. In “supra”, litter C increased from pasture to shrubland but not from shrubland to forest. Litter N increased with succession in “meso” but peaked in shrublands in “supra”; litter N in “thermo” increased from pasture to shrubland but not from shrubland to forest.
Litter C/N ratio increased in “supra” and “thermo” from pasture to shrubland and from shrubland to forest, but did not change significantly with succession in “meso”. “Supra” succession was characterized by the lowest litter C/N ratios with respect to the other two bioclimates. The largest increase in C/N ratio occurred in “thermo” secondary succession.
Discussion
Overall differences among bioclimates
The results obtained in this investigation confirmed an overall influence of bioclimate on soil and litter C and N content. Soil C stocks and N contents decreased from the colder, wetter climate to the warmer, drier climate. This observation can be explained by the combined effects of litter quality and limiting abiotic factors, which vary along an elevation gradient ([32]). Because net primary production in semi-arid environments can be limited by high temperatures and drought ([11]), the lower quantity of litterfall may account for lower soil C and N contents observed in thermomediterranean areas than in the meso- and supramediterranean areas. In contrast to soil C and N content, litter mass was greater in the warmer, drier thermomediterranean areas than in the colder, wetter meso- and supramediterranean areas. The latter findings may be interpreted as an effect of soil moisture, in that low soil moisture in drought periods may reduce litter decay rates by influencing the activity of soil microorganisms ([26], [42], [27]).
In addition, different bioclimates correspond to different vegetation types, which in turn produce litter of different quality. Indeed, mean C/N ratios were higher in “thermo” litter than in “supra” or “meso” litter, suggesting that reduced litter quality could have decreased the rate at which “thermo” litter decayed and thereby contributed to a reduced soil C and N accumulation in that bioclimate. Drier and warmer sites are also characterized by higher cover values of plants with an evergreen habit, which is another trait related to reduced litter quality ([50]).
Differences among successional stages within bioclimates
Our study confirmed that soil and litter C and N dynamics differ along the succession. Changes in C stocks and N contents in relation to successional stage may be due to change in plant community composition ([34], [49]), change in litter quality (also due to an increase in the content of secondary compounds, such as lignin or polyphenolic substances - [10], [44]), increases in soil moisture and decreases in soil temperature with shrub encroachment ([7], [20]), change in soil microbial ([54], [18], [30]) and macrofaunal ([14]) community structure, and decreases in bulk density following grazing abandonment and subsequent increases in the mineralization of soil organic matter ([42]).
Changes in C stocks and N contents with succession were greater in the 0-10 cm depth layer than at 10-30 cm depth both in the current study and in previous ones ([39], [19]). This may be due to litter, which causes the upper mineral soil layers to respond more rapidly than deeper layers to changes in aboveground C and N inputs. Moreover, bulk density decreased after grazing cessation (i.e., with the cessation of trampling) in the upper-most mineral soil horizon, but not in the deeper layers ([49]).
The successional differences in C stock and N content between the studied bioclimates may be due to the contrasting effects of litter transformability into humified organic matter and input mass, soil microclimate and soil C/N ratio, and the interaction among these factors. In general, the amount of C input into soil mineral layers is enhanced by the increase of the mass of aboveground litter, by more favorable soil microclimate for the transformation of litter to particulate organic matter, and by lower litter C/N ratios. Thus, ceteris paribus, all the above factors may determine a SOC lift up. In contrast, the presence of lignin or other secondary compounds in the litter may hamper its transformation as humified organic matter, lessening C inputs into soil mineral layers and thus reducing SOC. Lower soil C/N ratios and higher mineralization rates of soil organic matter with soil decompaction after grazing cessation would also decrease SOC. We hypothesize that the importance of some of these factors changes with bioclimate. In fact, our results indicate that SOC in “supra” succession is most influenced by changes in litter quality, while SOC in “thermo” succession is most influenced by changes in microclimate. Succession in “supra” started from pastures with high SOC stocks, high-quality litter, and a high soil C/N ratio. The subsequent decrease of SOC with shrub encroachment is caused by a decrease in litter transformability and an increase in mineralization rates of soil humified organic matter after soil C/N and bulk density decrease, which outweigh even the strong increase in litter C input occurring with succession from herb-dominated pasture to shrubland in the “supra” bioclimate. The subsequent increase of SOC with succession from shrubland to forest, however, is not driven by the same factors, because litter quality, soil C/N ratio, and bulk density decreased further. Although increased litter input might account for the SOC increase, we did not observe a significant change of litter C from shrubland to forest. It follows that SOC was influenced by some unknown factor that was not measured in our study.
In contrast to SOC in “supra”, SOC in “thermo” increased during shrub encroachment into grasslands. Because soil C/N ratio strongly increased at the same time, the SOC increase may be due to reduced mineralization rates of soil humified organic matter. The observed reduction in litter transformability into humified organic matter apparently had a weaker effect on SOC dynamics in “thermo” than in “supra”. In “thermo”, the limiting climatic conditions, i.e., low soil moisture and high temperature, could have played a major role in SOC increase because they reduce the rate of decay ([26]) especially in the pastures, where no shrub layer intercepts solar radiation and mitigates climatic conditions. The effects of low soil moisture and high temperature, however, are increasingly moderated as succession proceeds, and shrubs and then trees intercept the light and moderate the microclimate for litter decomposers ([21]).
Values of litter C/N observed in this study are comparable to those reported by other studies of thermomediterranean sites ([21], [52], [8]). Contrastingly, we observed values for soil C/N higher than those in comparable studies. Previous investigations reported soil C/N ratios ranging from 10 to 21 for thermomediterranean grasslands ([16], [41]), shrublands ([6], [55]), and forests ([47]). The high soil C/N that we observed in pastures may be due to wildfires (commonly linked to grazing activity in Sicily), and the subsequent N leaching from soil ([53]). The plant communities in thermomediterranean pastures are dominated by graminoid species with lower N leaf contents and litter decomposition than forbs ([9]), while meso- and supramediterranean pastures are dominated by forbs. Because the sclerophyllous, evergreen woody species that colonize thermomediterranean pastures are also characterized by low N contents ([31]), the transformation of their litter into humified organic matter immobilizes soil N ([45]). As a result, soil C/N increases with shrub encroachment.
As opposed to succession in “supra”, succession from shrubland to forest in “thermo” was not associated with an increase in SOC. The negative effect on SOC that could have been expected from the strong decrease of litter transformability (probably due to the growing cover of evergreen sclerophyllous species) and from a decrease in litter C input and bulk density, was clearly counterbalanced by the simultaneous reduction of mineralization rates of soil humified organic matter (due to soil C/N increase) and probably by soil microclimate or some other factors not measured in our study. Given the reduction of litter C input in the transition from shrublands to forests, we hypothesize that the increasing shrub cover could create more favorable conditions for the transformation of the high amounts of litter accumulated during shrub encroachment, whose decaying in “thermo” is hampered by the low moisture and high temperatures. Consequently, the substantial increase in the decay of litter mass would result in the substantial increase of inputs into the SOC pool.
Under “meso” conditions, the SOC stock remained stable along the succession gradient. Because litter C/N ratio but not litter quantity was also stable along the successional stages, litter quality could be a major factor influencing SOC in “meso”. However, we cannot exclude the possibility that the stabilization of SOC in “meso” could be the effect of two opposing factors: an increase in litter C input with succession would tend to increase SOC, while the decreases in bulk density and soil C/N ratio would tend to decrease SOC.
It is surprising that litter quality in “meso” remained stable along the successional gradient because shrub encroachment is accompanied by an increase in litter lignin content, which is expected to increase litter C/N ratio ([44]). Along the succession from pasture to shrubland, litter N increased less rapidly than litter C in “thermo” and “supra”, but increased at equal rates in “meso”, indicating that differences in litter quality among bioclimates were caused by differences in the colonizing shrub species. Vegetation in “supra” evolves from herb-dominated pastures with high-quality litter to deciduous shrublands, where litter quality is moderately lowered by lignin content. In contrast, vegetation in “thermo” evolves from pastures dominated by perennial grasses to evergreen low maquis, causing a strong reduction of litter quality due to enhanced lignin content and the presence of evergreen leaf litter. In “meso”, litter quality does not change significantly because the vegetation evolves from herb- and grass-dominated pastures to mainly deciduous shrublands, both of which produce litter of moderately low quality.
Conclusions
The present study showed that soil C and N storage in a semi-arid environment are affected by stage of succession and bioclimate, and that litter quality plays an important role in SOC dynamics. The differences in C stocks and N contents among bioclimates apparently reflected the contrasting effects of litter input (net primary production) and its transformation into humified organic matter (litter quality, temperature, and moisture). Successional changes in “supra” and “meso” seemed to be most influenced by litter quality, while those in “thermo” appeared to be strongly influenced by limited litter decay due to low soil moisture and high temperature.
Acknowledgments
This work was financially supported by the PRIN project “The impacts of secondary succession processes on carbon storage in soil and biomass and on biodiversity and the role of dispersal centres and vectors for recolonization processes” (National Coordinator: Riccardo Valentini). We thank Bruce Jaffee for the revision of the English version of the manuscript. We also thank the Assessorato Regionale Risorse Agricole e Alimentari - Dipartimento Interventi Infrastrutturali per l’Agricoltura - Servizio VI - Sistema Informativo Territoriale - Regione Siciliana (Palermo, Italy) for providing the unpublished pedological data. We also thank the Regional Park of Madonie for the logistic support provided.
References
CrossRef | Gscholar
Gscholar
Gscholar
Gscholar
CrossRef | Gscholar
Online | Gscholar
Gscholar
CrossRef | Gscholar
Gscholar
CrossRef | Gscholar
Gscholar
Gscholar
Authors’ Info
Authors’ Affiliation
L Gristina
E Rivaldo
S Pasta
A Novara
J Rühl
Dipartimento Scienze Agrarie e Forestali, University of Palermo, v.le delle Scienze, Ed. 4, I-90128 Palermo (Italy)
Corresponding author
Paper Info
Citation
La Mantia T, Gristina L, Rivaldo E, Pasta S, Novara A, Rühl J (2013). The effects of post-pasture woody plant colonization on soil and aboveground litter carbon and nitrogen along a bioclimatic transect. iForest 6: 238-246. - doi: 10.3832/ifor0811-006
Academic Editor
Giorgio Matteucci
Paper history
Received: Oct 05, 2012
Accepted: Mar 17, 2013
First online: Jun 13, 2013
Publication Date: Oct 01, 2013
Publication Time: 2.93 months
Copyright Information
© SISEF - The Italian Society of Silviculture and Forest Ecology 2013
Open Access
This article is distributed under the terms of the Creative Commons Attribution-Non Commercial 4.0 International (https://creativecommons.org/licenses/by-nc/4.0/), which permits unrestricted use, distribution, and reproduction in any medium, provided you give appropriate credit to the original author(s) and the source, provide a link to the Creative Commons license, and indicate if changes were made.
Web Metrics
Breakdown by View Type
Article Usage
Total Article Views: 54783
(from publication date up to now)
Breakdown by View Type
HTML Page Views: 46292
Abstract Page Views: 2758
PDF Downloads: 4277
Citation/Reference Downloads: 22
XML Downloads: 1434
Web Metrics
Days since publication: 4356
Overall contacts: 54783
Avg. contacts per week: 88.04
Article Citations
Article citations are based on data periodically collected from the Clarivate Web of Science web site
(last update: Mar 2025)
Total number of cites (since 2013): 17
Average cites per year: 1.31
Publication Metrics
by Dimensions ©
Articles citing this article
List of the papers citing this article based on CrossRef Cited-by.
Related Contents
iForest Similar Articles
Research Articles
Woody species recruitment under monospecific plantations of pioneer trees - facilitation or inhibition?
vol. 5, pp. 1-5 (online: 06 February 2012)
Research Articles
Net ecosystem production of a tropical secondary forest in Jengka, Pahang, Malaysia
vol. 18, pp. 54-60 (online: 04 April 2025)
Research Articles
First results on early post-fire succession in an Abies cephalonica forest (Parnitha National Park, Greece)
vol. 5, pp. 6-12 (online: 06 February 2012)
Research Articles
Evergreen species response to Mediterranean climate stress factors
vol. 9, pp. 946-953 (online: 07 July 2016)
Research Articles
Perceptions of forest experts on climate change and fire management in European Mediterranean forests
vol. 7, pp. 33-41 (online: 14 October 2013)
Review Papers
Large-scale effects of forest management in Mediterranean landscapes of Europe
vol. 6, pp. 342-346 (online: 29 August 2013)
Review Papers
Climate change impacts on spatial distribution, tree-ring growth, and water use of stone pine (Pinus pinea L.) forests in the Mediterranean region and silvicultural practices to limit those impacts
vol. 14, pp. 104-112 (online: 01 March 2021)
Research Articles
Spatial structure of the vertical layers in a subtropical secondary forest 57 years after clear-cutting
vol. 12, pp. 442-450 (online: 16 September 2019)
Research Articles
Scale dependency of the effects of landscape structure and stand age on species richness and aboveground biomass of tropical dry forests
vol. 16, pp. 234-242 (online: 23 August 2023)
Review Papers
Linking patterns of forest dieback to triggering climatic and weather events: an overview on Mediterranean forests
vol. 17, pp. 309-316 (online: 30 September 2024)
iForest Database Search
Search By Author
Search By Keyword
Google Scholar Search
Citing Articles
Search By Author
Search By Keywords
PubMed Search
Search By Author
Search By Keyword