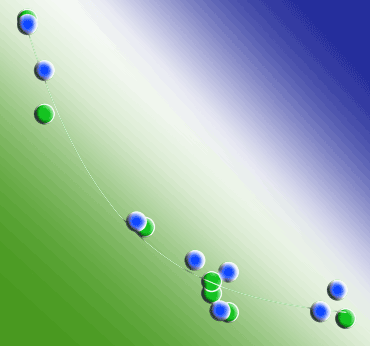
Testing a dual isotope model to track carbon and water gas exchanges in a Mediterranean forest
iForest - Biogeosciences and Forestry, Volume 2, Issue 2, Pages 59-66 (2009)
doi: https://doi.org/10.3832/ifor0491-002
Published: Mar 18, 2009 - Copyright © 2009 SISEF
Research Articles
Abstract
Due to climate change, drier summers have been observed over the last ten years in Mediterranean areas. Increasing drought levels may have a different weight in influencing the stomatal versus photosynthetic activity of forests, altering the water-use efficiency (i.e., WUE, the amount of carbon gain per water lost) and, consequently, the global carbon balance. By combining leaf gas exchanges and leaf carbon/oxygen isotope measurements, we tested under Mediterranean conditions a semi-quantitative dual isotope model to track adjustments in stomatal conductance (gs) and maximum CO2 assimilation at saturating light (Amax) in response to changes in air and soil water availability. The experiment was established at Allumiere site (Rome, Italy) over the course of two consecutive years. There, we modified the amount of precipitation reaching the soil on water depleted (D) and watered (W) replicate plots (~100 m2) of an Arbutus unedo L. forest using a system of rain gutters and sprinklers, respectively. Changes in soil water availability affected gs and Amax in parallel. As an application of the model, we found that, in response to reduced air and soil water availability, constant carbon (δ13C) and increasing oxygen (δ18O) isotope values were consistent with a parallel decline of either gs and Amax. As a result of parallel decline, WUE did not differ or only slightly differed between treatments, in contrast with most of the studies that found a wide-spread increase of WUE in response to enhanced drought. This study highlights the potentiality of the dual isotope model to provide insights of forest ecosystem functioning in Mediterranean environments.
Keywords
Carbon assimilation, Drought, Isotope model, Arbutus unedo, Stomatal conductance, Water-use efficiency
Introduction
In a Mediterranean-type climate, forest ecosystems are typically subjected to high temperature and scarce soil water availability during most of the summer. Moreover, due to the impact of climate change, more extreme drought periods are expected for the coming decades as a consequence of reduced precipitation during summers ([18], [31]). Under such conditions, gas exchanges will be largely limited by the availability of water for transpiration ([28]). Partial stomatal closure is one of the key mechanisms adopted by trees to save water and to avoid cavitation risks ([36], [19], [5]).
In turn, climate change may result in a negative impact on plant carbon assimilation through the increase of stomatal limitation to photosynthesis ([20], [22]). Thus, by the adjustments of stomatal conductance (gs) and maximum CO2 assimilation (Amax) in response to increasing drought will depend the amount of carbon that can be assimilated by trees. Additionally, these adjustments will determine the ability of Mediterranean forests to counterbalance the negative effect of increasing drought on growth and, consequently, on the gross primary production (GPP - [28]). In semi-arid environments, the shape and the values of Amax and gs will also determine the intrinsic water-use efficiency (WUEint), a component of the long-term water-use efficiency (WUET, the amount of carbon gain per water lost). WUEint is also a key parameter for deriving the productivity and the amount of carbon assimilated by forests ([29]).
Previous models were based on the simplifying assumption of a constant linear relationship between gs and Amax, with a simple dependence upon vapour pressure deficit (VPD - [38]). However, other studies have shown that this relationship is influenced by other environmental stresses such as soil moisture ([1], [23]). Non-stomatal limitations (i.e., mesophyll conductance and biochemical reactions - Rubisco, etc.) may be involved even under mild-moderate water stress ([13], [15]), thus changing the Amax and gs relationship (and consequently the WUE).
The use of stable carbon (δ13C) isotope as a powerful tool for investigating the balance between Amax and gs (see isotope theory) has grown steadily during the past two decades. The positive relationship between WUE and δ13C arises through their independent linkages to the ratio of internal to ambient CO2 concentrations (ci /ca - [10], [17]). To differentiate between the changes in δ13C driven by Amax or gs, Scheidegger et al. ([33]) proposed the incorporation of δ18O in a dual quantitative model. In fact, δ18O is affected by transpiration rates, which is closely correlated with gs ([3]).
The broader purpose of this study was to test in the Mediterranean environment a dual isotope conceptual model ([33]) to infer CO2 and H2O gas exchange activities of Arbutus unedo forest. To this aim, δ13C and δ18O in combination with direct measurements of leaf gas exchanges have been employed to give insight into the adjustments of gs and Amax resulting from different vapor pressure deficit (VPD) and soil water availability; this latter was changed by an experimental manipulation of the amount of precipitation reaching the soil. Furthermore, based on the observed variations in gs-Amax and δ13C, the effects of water restriction on WUE were also examined.
Isotope theory
Carbon and oxygen isotopes
The stable isotope technique has been revealed to be an important tool in identifying medium and long-term effects of environmental factors on CO2 and H2O gas exchanges in plants. The carbon isotope composition (δ13C) of leaf organic matter reflects the fractionation processes occurring during the diffusion of 12CO2 and 13CO2 through stomatal pores and photosynthetic assimilation. In C3 plants, discrimination against 13C is linked to photosynthesis via ci/ca, the ratio of intercellular (ci) to atmospheric (ca) CO2 concentrations as described by Farquhar et al. ([9] - eqn. 1):
where δ
13C
leaf and δ
13C
atm are the carbon isotope compositions of leaf organic matter and atmospheric CO2, respectively, and a
and b
are fractionation factors occurring during diffusion of CO2 through stomata pores (-4‰) and enzymatic C-fixation by Rubisco (-27‰), respectively. According to eqn. 1, a lower ci/ca ratio results in an increased δ13Cleaf due to a lower discrimination (Δ) against 13CO2. Environmental factors such as water availability and irradiance can cause variations of ci/ca, mainly through their effects on both stomatal conductance (gs) and photosynthetic activity ([10]).
δ
13C has largely been used as a proxy for long-term water-use efficiency (WUE, the amount of carbon gained per water transpired) of C3 plants ([10]), thanks to their independent linkages to ci/ca (eqn. 2):
where VPD
is the vapour pressure difference between the intercellular spaces and the atmosphere and Φ
is the fraction of carbon respired by the plants. However, a simpler concept of WUE is often used (i.e., intrinsic water-use efficiency (WUEint = A/gs, the ratio of CO2 assimilation to stomatal conductance) or instantaneous water-use efficiency (WUEinst = A/E, the ratio of assimilation to leaf transpiration) when the leaf-to-air vapour pressure difference is known ([10]). These are both components of long-term WUE. Although it successfully captures WUE trends, δ13C fails to account for factors responsible for variations in WUE. These variations can be the result of changes in gs or A. For example, an increase in δ13C, interpreted as a reduction in ci and an improvement in WUE in the Farquhar model, can be the result of either: (1) reduced gs (at constant A); or (2) increased A (at constant gs).
The oxygen isotope composition (δ18O) of leaf organic material can be used for distinguishing between possible causes of variation in δ13C, thanks to the link of δ18O with the isotopic fractionation of water during transpiration in leaves. During transpiration, molecules of water containing lighter isotopes (H2 16O) tend to diffuse faster from the site of evaporation to the atmosphere. In this way, water becomes enriched in the heavier isotopes of 18O, compared to water coming from the soil. The oxygen isotopic composition of leaf water at the sites of evaporation (ΔE) is expressed as follows ([6], [8], [11] - eqn. 3):
where ΔV is the oxygen isotopic composition of water vapour in the air, ε* is the temperature-dependent fractionation associated with the lower vapour pressure of H218O compared to that of H216O, εk is the kinetic fractionation during evaporative water diffusion through the stomata and boundary layer, and ea/ei are the vapour pressures in the atmosphere and intercellular air spaces, respectively.
Thus, according to this equation, the degree of leaf water enrichment depends on the rH. The latter represents the evaporative driving force, and a reduced rH causes an increase of δ18O in the leaf water. This enrichment is then expected to be reflected in the organic matter ([7], [39], [12], etc). δ18O, determined in this manner, has often been found to be negatively correlated with gs ([3], [14]).
Linking δ13C and δ18O in a dual isotope conceptual model
The dual isotope conceptual model proposed by Scheidegger et al. ([33]) represents a simplified tool to infer Amax and gs from the variation of δ13C and δ18O in plants. Fig. 1 shows all combinations of δ13C and δ18O values in eight likely scenarios where the outputs of the model (i.e., gs and Amax) are selected on the basis of changes in rH. Different environmental conditions can cause higher (↑), lower (↓) or similar (≈) δ13C and δ18O values. As an example, we refer to scenario b) where both δ13C and δ18O values increased. We know from the increase in δ18O that rH must have increased, while from the increasing δ13C, we infer a decreasing ci. This reduction is explained by two possible cases: (1) Amax ↑ and gs ≈ or (2) Amax ≈ and gs ↓. Because plants in dry air tend to close their stomata, we choose case (2) as it is physiologically more plausible than case (1). Thus, δ13C reflects variation in ci, while δ18O is affected by variation in rH. This variation, in turn, drives changes in transpiration rates and gs.
Fig. 1 - The conceptual isotope model from Scheidegger et al. ([33]): scheme of the eight scenarios from a) to h) based on all the likely δ13C-δ18O combinations (model input). The changes are shown by the arrows. Relative humidity (rH) is derived from δ18O, while ci is derived from δ13C (symbols: ↑, ≈ and ↓ represent increase, no response and decrease in levels, respectively). For each scenario there are two possible cases, indicated as 1 and 2, with corresponding changes in Amax and gs. The model output at the bottom gives relative Amax and gs based on the rH changes.
Materials and methods
Study site and experimental design
The research was carried out in a drought-prone 50 ha of Mediterranean macchia. The site was dominated by the coppice A. unedo, which covers 65% of the surface. The study site, environmental conditions and stand characteristics are detailed in Tab. 1.
Tab. 1 - Site, environmental conditions and stand characteristics. (1): Soil Taxonomy; USDA Soil Survey Staff 1999.
Location | Allumiere, Lazio, Italy |
---|---|
Latitude | 42° 11’ |
Longitude | 11° 56’ |
Altitude (m a.s.l.) | 180 |
Slope | Uniform |
Soil type | Andsols(1) |
Soil depth (cm) | 31 |
pH | 4 |
Annual rainfall (mm) 1951 - 2005 |
919 |
Summer rainfall (mm) 1951 - 2005 |
120 |
Annual temperature (°C) 1951 - 2005 | 13.6 |
Age of trees (years) | 25 |
Tree density (trees ha-1) | 4070 |
Stem diameter at 1.3 m aboveground (cm) | 5 |
Height (m) | 5 |
Leaf area index (m m-2) | 5.5 |
Total basal area (m2 ha-1) | 19.6 |
Two different levels of volumetric soil water content (SWC, volume of water per volume of soil, multiplied by 100) have been imposed by an alteration of the amount of precipitation reaching the soil. This was done during the summers of 2004 and 2005 (June to August) on three replicated plots (~100 m2). A mean value of 7% in SWC was obtained by partial rain exclusion (-20%), using a system of pipes suspended about 1.8 m above the forest floor (water-depleted plots, D). A mean value of 14% in SWC was obtained by adding water through a sprinkler net to simulate rain events (watered plots, W). A 10% threshold of SWC, established in a pre-treatment experiment, represented the dry (below) and well-watered (above) conditions ([30]).
Water relations and gas exchange measurements
SWC was measured within the D and W treatments by probes (Campbell Scientific, INC, Logan, Utah, USA). These probes consisted of two 30 cm long stainless steel rods, fully inserted into the soil at six different locations per replicate. The time domain reflectometry (TDR) method was used to translate the readings in SWC ([35]).
Eight intensive field campaigns were carried out during 2004 and 2005 to assess plant water status. Predawn leaf water potential (Ψ
pd) was measured on six to eight experimental trees per replicate in D and W plots. For each tree we measured five fully expanded leaves with a Scholander-type pressure chamber (PMS Instruments, Corvallis, OR, USA).
Maximum CO2 assimilation at saturating light (Amax) and stomatal conductance (gs) were measured using a portable infrared gas analyzer (LI-6400 Li-cor, Lincoln, NE, USA). Measurements were performed on 10 sunlit leaves of six trees growing in the central portion of each replicated plot to avoid the “edge effect”. Hours between 11:30 am and 15:30 pm on cloudless days were chosen for all measurements. This is when environmental conditions were most stable and when photosynthetic photon flux density (PPFD) was above 1200 μmol m-2 s-1 (above saturating light conditions for A. unedo - data not shown). In order to take into account the effect of vapour pressure deficit (VPD) in driving variation in WUE, we considered the instantaneous WUE (WUEinst), calculated from gas exchange measurements, as the ratio of Amax to leaf transpiration rate (E).
Carbon and oxygen isotope analysis
Six samples of non-fully expanded leaves were collected from each replicate plot in five field campaigns (June, July 2004 and June, July, September 2005) to measure carbon (δ13C) and oxygen (δ18O) isotopes. Leaf samples were collected from the same shoots where gas exchange measurements were performed.
Bulk leaf samples were dried, ground to a fine powder and then weighed in tin capsules, using a value of 0.6-0.8 mg for δ13C and 1.1-1.3 for δ18O. Bulk leaf material was combusted to CO2 for carbon isotope analysis in an elemental analyzer (EA-1108, Carlo Erba, Italy), which was connected to a mass spectrometer (Delta-S Finningan MAT, Germany) via a variable open split interface (CONFLO II Finnigan MAT, Germany). For determination of δ18O, leaf bulk material was pyrolized ([32]) to CO with an elemental analyzer (EA-1108, Carlo Erba, Italy), connected to the same mass spectrometer.
δ13C and δ18O were calculated as (eqn. 4):
where R is the isotope ratio and 13C/12C or 16O/18O refer to the sample and to the standard, respectively. The isotope values are expressed in delta notation, with V-PDB for carbon and VSMOW for oxygen. The accuracy of the method was ± 0.2‰ for δ13C and ± 0.2‰ for δ18O.
Statistical analysis
Values of treatments (W, D) are presented as the mean ± standard error and compared using the Student-Newman-Keuls test. Statistical significance was defined as P ≤ 0.05 and P ≤ 0.01. Linear regressions were analysed using Pearson correlation coefficients. All statistics were computed with the SPSS statistical package (SPSS, Chicago, IL).
Results
Carbon and water relations
As a consequence of treatments, significant differences in soil water content (SWC) emerged between water depleted (D) and watered (W) plots, although such differences were higher in 2005 than 2004 (Tab. 2).
Tab. 2 - Variations in vapour pressure deficit (VPD), soil water content (SWC), predawn water potential (Ψpd), stomatal conductance (gs), maximum CO2 assimilation (Amax), intercellular CO2 concentration (ci), instantaneous water-use efficiency (WUEinst), bulk leaf carbon (δ13C) and oxygen (δ18O) isotope composition in water depleted (D) and watered (W) plots during the experiment. Values marked with asterisks are significant for P < 0.05.
Date | Plot | VPD (kPa) |
SWC (%) |
Predawn Ψ (MPa) |
gs (mol m-2s-1) |
Amax (μmol m-2s-1) |
ci (ppm) |
WUEinst (μmol mol-1) |
δ13C (‰) |
δ18O (‰) |
---|---|---|---|---|---|---|---|---|---|---|
Jun-04 | D | 3.2 | 13* | -0.54 | 0.107 | 5.63 | 254 | 1.82 | -26.7 | 29.11 |
W | 3.3 | 17* | -0.46 | 0.113 | 5.21 | 257 | 1.79 | -26.9 | 29.30 | |
Jul-04 | D | 4.6 | 12* | -0.97* | 0.044* | 3.20* | 235 | 2.21* | -26.6 | 30.37* |
W | 4.4 | 15* | -0.86* | 0.078* | 5.24* | 230 | 1.81* | -26.8 | 31.22* | |
Jan-05 | D | 0.9 | 16 | -0.07 | 0.108 | 6.56* | 253 | 7.20 | - | - |
W | 0.9 | 18 | -0.05 | 0.125 | 7.86* | 246 | 7.30 | - | - | |
Feb-05 | D | 1.1 | 17 | -0.47 | 0.084* | 6.06 | 239 | 6.34* | - | - |
W | 1.1 | 20 | -0.45 | 0.110* | 7.05 | 252 | 5.52* | - | - | |
Apr-05 | D | 4.4 | 23 | -0.57 | 0.130* | 7.92* | 267 | 1.80 | - | - |
W | 4.7 | 23 | -0.52 | 0.161* | 9.02* | 273 | 1.68 | - | - | |
Jun-05 | D | 2.9 | 8* | -0.65 | 0.121* | 7.80 | 227 | 2.77* | -25.8* | 28.60 |
W | 3.1 | 14* | -0.61 | 0.148* | 8.93 | 235 | 2.15* | -26.3* | 28.70 | |
Jul-05 | D | 3.3 | 5* | -0.89* | 0.078* | 5.84* | 216 | 2.55 | -26.1 | 28.80* |
W | 3.1 | 15* | -0.44* | 0.162* | 10.11* | 228 | 2.37 | -25.9 | 29.26* | |
Sep-05 | D | 2.2 | 10* | -0.34 | 0.122* | 7.88* | 239 | 3.50 | -26.3 | 27.20* |
W | 2.3 | 21* | -0.41 | 0.151* | 8.95* | 238 | 3.39 | -26.5 | 27.90* |
In general, predawn water potentials (Ψpd) reflected the SWC conditions during the two years of the experiment (Tab. 2). Significant differences in Ψpd were observed in the summer of 2004 and 2005 between D and W plots (P < 0.05), demonstrating the effectiveness of the applied treatments, even if the mean values for D plots never exceeded -1.0 MPa. Minimum Ψpd in the D treatment was similar in both years.
Leaf vapour pressure deficit (VPD) showed a bimodal pattern with a minimum in winter-spring and a maximum in summer, although July 2004 (4.8 kPa) was drier than July 2005 (3.5 kPa - Tab. 2).
Drier conditions during the summer had a strong effect on gas exchange activities. Significant reductions of stomatal conductance (gs) and maximum CO2 assimilation (Amax) were observed over the seasons, in response to changes in water availability (Tab. 2). gs reached the maximum value (0.16 mol m-2 s-1) in spring 2005 when environmental conditions were most favourable, whilst the lowest minimum rate was recorded in July 2004 (0.04 mol m-2 s-1) under air and soil humidity stress. Despite the same Ψpd, the difference in gs measured between July 2004 and 2005 was 0.3 mol m-2 s-1. This was likely due to differences in VPD recorded between the two dates.
Significant differences in Amax between the D and W treatments were observed during the experiment, in particular during the summer drought. However, Amax values in D plots did not fall below 60% of those observed in the W treatment (Tab. 2); this was the consequence of not marked drought treatment imposed.
Because of parallel declines in gs and Amax, computed intercellular CO2 (ci) concentration and instantaneous water-use efficiency (WUEinst) did not differ between treatments (Tab. 2). A slight increase in WUEinst was observed in D plots during July 2004 and 2005. However, WUEinst showed large fluctuations over the season, mainly related to changes in VPD (Fig. 2).
Fig. 2 - Correlation of instantaneous water-use efficiency (WUEinst) and vapour pressure deficits (VPD) in water depleted (D) and watered (W) plots during the experiment.
13C/12C and 18O/16O isotope ratios in leaf organic matter
The carbon isotope composition (δ13C) was not affected by changes in SWC and VPD during the experiment (Tab. 2), with the exception of June 2005, when only slight and significant differences were shown between D and W plots. This result was consistent with our gas exchange findings, in which little or no changes in intercellular CO2 concentration (ci) and WUEinst were found (Tab. 2). Furthermore, δ13C was negatively correlated with ci/ca as derived from leaf gas exchange assessments (Fig. 3). However, this result was significant only for treatment D (P < 0.05) and D+W (P < 0.01). A significant and positive relationship (r = 0.76, P < 0.05) was also found between δ13C and WUEinst (data not shown).
Fig. 3 - Correlation between leaf carbon isotope composition (δ13C) and intercellular to ambient CO2 concentrations (ci/ca) assessed by gas exchange in water depleted (D) and watered (W) plots during the summer in 2004 and 2005. Symbols represent the mean value ± standard error for each date of measurement.
In contrast, δ18O was found to be more sensitive to variations in soil water availability than δ13C. In fact, δ18O differed significantly between D and W plots in July 2004 and July/September 2005 (Tab. 2). A lack of difference in δ18O values recorded in June 2004 between D and W treatments suggests that full access to water for A. unedo trees did not differentiate g s from the transpiration response.
δ18O significantly increased in response to reduced stomatal aperture (P < 0.05) and to the consequent leaf transpiration rate (Fig. 4). The correlation was not significant when the two treatments were considered separately, but they showed a parallel increase in δ18O with a reduction of gs. Both had a similar slope from the linear fit. A significant and negative correlation (r = -0.67; P < 0.05) was observed between δ18O and WUEinst (data not shown).
Fig. 4 - Correlation between leaf oxygen isotope composition (δ18O) and stomatal conductance (gs) assessed by gas exchange in water depleted (D) and watered (W) plots during the summer in 2004 and 2005. Symbols represent the mean value ± standard error for each date of measurement.
As a test of the dual isotope conceptual model, δ13C was found not significantly correlated with δ18O (r = 0.42, P = 0.23 - Fig. 5, top-left panel). The higher VPD during the period of leaf formation in 2005 led to a parallel increase in δ18O (more positive values) in both D and W plots. In contrast, δ13C was less sensitive to VPD changes, showing only about 1.0‰ of variation. The direction of the arrow in the δ13C-δ18O relationship matches scenarios C) and G), indicating that g s and A max were simultaneously affected by decreasing (scenario C) or increasing (scenario G).
Fig. 5 - The δ13C-δ18O relationship (above left panel) in water depleted (D) and watered (W) plots during the summer in 2004 and 2005. The top right panel provides information derived from the δ13C-δ18O relationship: this result is consistent with case c) in the Scheidegger model with the arrow pointing to right side; the model output of gs-Amax indicates a decrease of either gs or Amax. The bottom panel shows the correlation between Amax and gs from gas exchange measurements. Symbols represent the mean value ± standard error for each date of measurement.
Discussion
Although the study period was slightly wetter than the area’s long-term average (i.e., 1095 mm in 2004 and 935 mm in 2005 - Tab. 2), the summer (June to August) was drier (i.e., 90 mm in 2004 and 80 mm in 2005). Thus, the reduction in precipitation was 25% for the summer of 2004 and 33% for the summer of 2005 when compared to the long term average. This gives further evidence in predicted drier summers for Mediterranean regions ([18]). Moreover, the reduction of total precipitation (-20%) induced by the experimental manipulation exacerbated the summer drought.
As a result, the enhanced drought affected water and carbon relations, leading to reductions in maximum CO2 assimilation (Amax) and stomatal conductance (gs) for A. unedo forests over the two monitored seasons. This result is in agreement with other studies on the same species ([4], [16]). Furthermore, variations in directly measured leaf physiological traits, e.g., the intercellular to ambient CO2 (ci/ca) ratio and gs, were well reflected in the carbon (δ13C) and oxygen (δ18O) isotope signature measured in bulk leaf material. In particular, our data show that measurements of δ13C can be used to make time-integrated estimates of ci/ca at the tree or stand scale ([10]). In addition, the negative correlation between δ18O and gs that we observed for D+W is consistent with other studies ([3], [2], [34]). This indicates that δ18O values reflect the signal of gs variations under Mediterranean conditions. Although the correlation was not significant when the two treatments were considered separately, the similar slope and the parallel trend for the linear fit highlights the differences in gs behaviour in response to different water availabilities. In fact, with δ18O equal, gs was lower in D compared to W. This confirms stomatal control of transpiration, imposed by soil water restriction and coupled with high VPD conditions.
Thus, the reliable correlation observed between δ18O and gs suggests that bulk leaf material was a suitable medium to infer the physiological performance of trees. This was previously observed by Sullivan & Welker ([34]) and Barbour et al. ([2]). While in other studies, the extraction of leaf cellulose was necessary because the δ18O determined in leaf matter was not correlated with gs ([14]).
The combination of δ13C and δ18O in a semi-quantitative model revealed qualitative variations of Amax and gs across two different soil water regimes. Thus, in this investigation, the dual isotope model proposed by Scheidegger et al. ([33]) was modified with the inclusion of SWC and rH (or VPD) as model inputs. Isotopic measurements on bulk leaf material showed an increase in δ18O and a slight variation in δ13C. Among the eight different δ13C and δ18O scenarios proposed in the model, our results matched scenarios C and G (see isotope theory). This means that ci remained almost constant, that g s and A max were simultaneously affected, and that both increased (scenario G) or decreased (scenario C). Therefore, as air and soil water availability reduced in our experiment, we can assume that both g s and A max decreased (scenario C). The output of the model found a robust confirmation from our gas exchange measurements. In fact, we did not observe variation in ci between D and W over the two years of the experiment. Furthermore, direct measurements of gas exchange revealed a reduction of both Amax and gs with increasing water stress (from W to D). The information deduced from the carbon and oxygen stable isotopes reflects the long-term integrated information of Amax and gs (because organic matter accumulates over some time) and are consistent with short-term gas exchange measurements on a different time scale.
The down regulation of g s may have induced a parallel decrease in A max, suggesting that stomata strongly limit carbon assimilation. However, a similar c i, found in this experiment in D and W plots, indicates that photosynthesis may have been down-regulated and that non-stomatal limitations may have played an important role ([22]). Through a study of quantitative limitation analysis carried out in July 2004 on the same experimental site, it was shown that non-stomatal limitations largely affected A max (Grassi et al., unpublished data), accounting for 35% of total limitations (9% biochemical - carboxylation and electron transport rate - and 26% resistance to CO2 from intercellular spaces to carboxylation sites). Several studies have shown evidence that this is far from negligible, and it is often the most important factor under moderate water stress (e.g., [25], [37], [15]).
Although the dual isotope approach has been shown to be a reliable tool to infer the relationship between gs and Amax, it is not able to explain in details the underlying mechanism involved. In fact, under Mediterranean conditions, it is often necessary to assess the contribution of stomatal and non-stomatal limitations in driving changes in Amax and gs and their weight in this ratio that, in turn, affects the WUE.
As a result of the parallel decrease in gs and Amax, we observed little or no differences in either WUEinst and integrated WUE as assessed by δ13C. This result contrasts with most findings in Mediterranean areas, where a significant increase in WUE has been found ([27], [24], etc.). These results derive from a more intense stomatal control of water loss than inhibition of photosynthesis.
The large fluctuations in WUEinst over the two seasons appear to be mainly related to changes in VPD. Thus, VPD is considered an important parameter in driving gas exchanges in Mediterranean environment ([26]) and in influencing the productivity of forest ecosystems ([21]).
Conclusions
The combination of δ13C-δ18O, in a semi-quantitative conceptual model, proved to be a valid tool for investigating the time integrated gs-Amax relationship under Mediterranean conditions. In fact, a constant δ13C and an increase of δ18O isotopes in response to reduced air and soil water availability were consistent with a parallel decline of either gs and Amax, as assessed by gas exchange. The good correlations found between δ13C and ci or between δ18O and gs confirm this result. Furthermore, either instantaneous (from gas exchanges) and integrated WUE (from δ13C isotopes) data are in agreement in showing that, as a consequence of a parallel decrease of either gs and Amax, soil water restriction had no or slight influence on WUE. VPD was shown to have a larger impact on WUE than SWC. Such a response should result in a negative feature under climate change scenarios that may further reduce the carbon sequestration and the productivity of Mediterranean forests.
Acknowledgments
This research was supported by the EU Project n. EKV2-CT-2002-00158 MIND “Mediterranean terrestrial ecosystem and Increasing Drought” and the MIUR-PRIN Project prot. 003073315_003 “Drought and Mediterranean forests: stomatal mechanisms in the regulation of plant gas exchanges”. The authors would like to thank the coordinator of the MIND project, Dr. Franco Miglietta, and the Principal Investigator of the research group, Prof. Marco Borghetti. The authors also express their gratitude to Raddi S, Nolè A, Lapolla A, Anichini M, Cantoni L and Vicinelli E for helpful support in field measurements.
References
Gscholar
Gscholar
Gscholar
Gscholar
Gscholar
Gscholar
Gscholar
Gscholar
Authors’ Info
Authors’ Affiliation
MR Guerrieri
R Guarini
Dept. of Crop System, Forestry and Environmental Sciences, University of Basilicata (Italy)
R Siegwolf
M Jäggi
Paul Scherrer Institut, Laboratory of Atmospheric Chemistry, Stable Isotope and Ecosystem Fluxes (Switzerland)
Dept. of Plant Cultivation, University of Bologna (Italy)
Corresponding author
Paper Info
Citation
Ripullone F, Guerrieri MR, Saurer M, Siegwolf R, Jäggi M, Guarini R, Magnani F (2009). Testing a dual isotope model to track carbon and water gas exchanges in a Mediterranean forest. iForest 2: 59-66. - doi: 10.3832/ifor0491-002
Academic Editor
Roberto Tognetti
Paper history
Received: Oct 13, 2008
Accepted: Feb 20, 2009
First online: Mar 18, 2009
Publication Date: Mar 18, 2009
Publication Time: 0.87 months
Copyright Information
© SISEF - The Italian Society of Silviculture and Forest Ecology 2009
Open Access
This article is distributed under the terms of the Creative Commons Attribution-Non Commercial 4.0 International (https://creativecommons.org/licenses/by-nc/4.0/), which permits unrestricted use, distribution, and reproduction in any medium, provided you give appropriate credit to the original author(s) and the source, provide a link to the Creative Commons license, and indicate if changes were made.
Web Metrics
Breakdown by View Type
Article Usage
Total Article Views: 54596
(from publication date up to now)
Breakdown by View Type
HTML Page Views: 46904
Abstract Page Views: 2930
PDF Downloads: 4083
Citation/Reference Downloads: 63
XML Downloads: 616
Web Metrics
Days since publication: 5610
Overall contacts: 54596
Avg. contacts per week: 68.12
Article Citations
Article citations are based on data periodically collected from the Clarivate Web of Science web site
(last update: Feb 2023)
Total number of cites (since 2009): 18
Average cites per year: 1.20
Publication Metrics
by Dimensions ©
Articles citing this article
List of the papers citing this article based on CrossRef Cited-by.
Related Contents
iForest Similar Articles
Research Articles
Total RNA extraction from strawberry tree (Arbutus unedo) and several other woody-plants
vol. 1, pp. 122-125 (online: 27 August 2008)
Research Articles
Drought tolerance in cork oak is associated with low leaf stomatal and hydraulic conductances
vol. 11, pp. 728-733 (online: 06 November 2018)
Research Articles
Developing stand transpiration model relating canopy conductance to stand sapwood area in a Korean pine plantation
vol. 14, pp. 186-194 (online: 14 April 2021)
Research Articles
Use of δ13C as water stress indicator and potential silvicultural decision support tool in Pinus radiata stand management in South Africa
vol. 12, pp. 51-60 (online: 24 January 2019)
Research Articles
Role of photosynthesis and stomatal conductance on the long-term rising of intrinsic water use efficiency in dominant trees in three old-growth forests in Bosnia-Herzegovina and Montenegro
vol. 14, pp. 53-60 (online: 28 January 2021)
Research Articles
Gas exchange, biomass allocation and water-use efficiency in response to elevated CO2 and drought in andiroba (Carapa surinamensis, Meliaceae)
vol. 12, pp. 61-68 (online: 24 January 2019)
Research Articles
Sap flow, leaf-level gas exchange and spectral responses to drought in Pinus sylvestris, Pinus pinea and Pinus halepensis
vol. 10, pp. 204-214 (online: 01 November 2016)
Research Articles
Magnolia grandiflora L. shows better responses to drought than Magnolia × soulangeana in urban environment
vol. 13, pp. 575-583 (online: 07 December 2020)
Research Articles
Light acclimation of leaf gas exchange in two Tunisian cork oak populations from contrasting environmental conditions
vol. 8, pp. 700-706 (online: 08 January 2015)
Research Articles
A comparison between stomatal ozone uptake and AOT40 of deciduous trees in Japan
vol. 4, pp. 128-135 (online: 01 June 2011)
iForest Database Search
Search By Author
Search By Keyword
Google Scholar Search
Citing Articles
Search By Author
Search By Keywords
PubMed Search
Search By Author
Search By Keyword