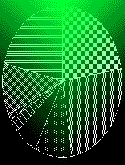
Effects of warmer and drier climate conditions on plant composition and biomass production in a Mediterranean shrubland community
iForest - Biogeosciences and Forestry, Volume 1, Issue 1, Pages 39-48 (2008)
doi: https://doi.org/10.3832/ifor0418-0010039
Published: Feb 28, 2008 - Copyright © 2008 SISEF
Research Articles
Abstract
The last IPCC report predicts warmer and drier conditions for the future European climate and the Mediterranean basin could be highly sensible to future climatic change. In order to investigate how the forecast more stressing factors could affect Mediterranean shrubland ecosystems, an appropriate manipulation of the microclimate was carried out in an area covered by Mediterranean maquis aimed at extending the drought period and increasing the night-time temperature. Soil cover, plant growth, litterfall, leaf water status, and leaf nutritional status were monitored over three growing seasons. The manipulation altered the microclimate according to common scenarios, increasing mean annual night-time air temperature by about 1 °C and mean annual temperature by about 0.5 °C, and decreasing precipitation between 6-46% of the total rainfall during the growing seasons. A general increase of vegetation cover was observed in the whole community during the three years of experimentation. This positive temporal pattern was mainly observed in control and warming treatment, whereas in the drought treatment it was less evident. At species-specific level, a clear negative effect of drought treatment was observed for C. monspeliensis percentage cover. Shoot elongation was not significantly affected by the warming treatment. A significant negative effect of drought treatment was noticed in the 2001-2002 and 2002-2003 growing seasons. An increase of N and P concentrations in the drought treatment in Cistus was observed and it can be explained by the reduced shoot growth induced by the water shortage that we had observed in the same treatment. The absence of a concentration effect on the other two species could be the signal of the different behaviour with regard to a drier climate, and therefore could be a symptom of future change in species composition. We underline the need of long-term observation, because of the different responses of plants in the short and long- term conditions.
Keywords
Mediterranean shrublands, Plant production, Night-time warming, Drought period extension
Introduction
The last IPCC report predicts warmer and drier conditions for the future European climate ([35]). In particular, the Southern part of the continent will experience an increasing average temperature (0.1-0.4 °C per decade), as a consequence of increased night-time temperature ([22]), and reduced precipitations, (from -1% to -5% per decade, [17], [16]). Both phenomena will be mostly evident in summer, hence drier climate conditions will be likely to occur.
The Mediterranean area might be highly sensible to future climate change ([27], [50], [51], [46], [11], [49], [36]). In this area shrublands ecosystems represent an important vegetation component, a widespread variety resulting from degradation of Mediterranean forests caused by anthropic activities (agriculture, pasture, wood, and recently tourism, etc.) carried out for thousands of years by native populations for their purpose needs. The Italian “gariga” is a shrubland community that represents the first step of soil covering process subsequent to disturbances (fire, grazing, deforestation. An example of gariga is the formation with Cistus monspeliensis and C. creticus subsp. eriocephalus, with Dorycnium pentaphyllum ([7]). The Cistus species are pyrophyte, i.e. pioneer plants spreading by seed and forming dense stands after fires ([64]). These stands represent the first step after the establishment of herbaceous plants and rustic suffrutices, such as Helichrysum italicum, that abound in poorer and more degraded edaphic conditions ([41]). Climatic variation can alter the conditions that allow the evolution of this type of vegetation towards more evolved aspects, such as those represented by maquis with holm oak. The predicted lower precipitations and higher temperature may cause a general degeneration of Mediterranean forests through direct effects on plants (increased transpiration, photodamage, lower photochemical efficiency, decreased water use efficiency, decrease of productivity) and indirect effects on the abiotic component (soil erosion, nutrient leaching, more frequent wildfires - [9], [34], [12], [23], [38], [45], [5], [32], [39], [21], [26], [47], [58], [41]). This would imply loss of habitats and species, with the migration of Southern species northwards, as reported in Skov & Svenning ([61]) and Hughes ([33]).
The aim of this work was to investigate how Mediterranean shrubland ecosystems could respond to longer drought period and increased night-time temperature, which were obtained by an appropriate microclimate manipulation in an area covered by garrigue. We supposed that the increased temperature and the lower water availability would have changed the community structure, inducing a lower growth for the “more evolved” species, such as C. monspeliensis, in favour of the more rustic one (H. italicum). In a 4-year study we monitored: species cover, plant growth by measuring woody biomass, litterfall and shoot elongation, water status by leaf water potential and nutritional status by leaf chemical analysis.
Materials and Methods
Description of the area
The experimental area (about 0.12 ha) is located in the peninsula of Capo Caccia (north-western Sardinia), within a Natural Reserve (40° 37’N, 8° 10’ E). Four different vegetation types can be easily identified in the area: a quite recent forested area of pine trees, the Mediterranean macchia, a Quercus ilex forest and the garrigue, located near the coast line and dominated by small bushes and tiny herbaceous plants growing between emerging rocks. The geologic substrate is Mesozoic limestone ([15]). The main soil type is Terra Rossa (Lithic Xerorthent and Typic Rhodoxeralfs - [68], [70], [52]). The soil is rocky and shallow (20-30 cm); the texture is sandy loam, with an ABC profile, and neutral pH.
The climate is semi-arid: the average rainfall amount is 640 mm yr-1; precipitation falls in autumn and spring with a long dry period from May to August, with about 60 mm. The mean annual temperature is 16.8 °C. The mean of minimum temperatures in the coldest month is 7 °C, the mean of the maximum temperatures in the hottest month is 28 °C (meteorological station of Fertilia Airport N 40°38’ E 8°17’; altitude 40 m a.s.l.; sea distance 4273 m; period of observation 1961-1990).
The experimental plots are located in a fire-break strip realized in 1973. The fire-break strip, nearly wide 125 x 10 m, slope 10%, had been managed by controlled fire until 1990, then mechanically in 1991 and 1992. Since 1993 no other intervention has been done and vegetation started to colonize the soil. The plots are covered by vegetation with a maximum height of 1.0 m mainly represented by Cistus monspeliensis, Helichrysum italicum and Dorycnium pentaphyllum. The presence of other shrubs (Pistacia lentiscus, C. creticus, Daphne gnidium, etc.) is more sporadic. Bare soil is about 20% of soil cover.
Microclimate manipulation and climate monitoring
The experimental manipulation of climate conditions at field scale was carried out employing a newly developed night-time warming technique and an automated covering system to extend summer drought ([6] - Fig. 1). Extended summer drought was induced by covering the plots with waterproof plastic curtains, transparent to infrared radiation, during rain events that occurred during two periods per year, in spring and in autumn (May 1st - June 13th 2002; August 27th - November 15th 2002; April 1st - May 31st 2003; October 1st - November 30th 2003; April 1st - May 31st 2004; October 1st - November 30th 2004). Passive night-time warming was induced throughout the year by covering vegetation and soil with aluminium curtains at night. This method enables the ecosystem to retain a portion of energy accumulated during the light period, simulating the mechanism of global warming. The curtains run along two rails placed on a light scaffolding 1.5 m tall. The curtains were coiled on a beam and connected to a motor. The movement of the curtains was controlled automatically by an electronic devise set to the following conditions:
Fig. 1 - The plot is delimited by light scaffolding, upon which two rails allow the curtains to run and mimic the climate change. For more details see the text.
- the warmed plots were automatically covered when light intensity at sunset was lower than 0.4 W m2. At sunrise the curtains were retracted to leave the plots open during the day;
- a rain sensor activated the curtains to cover the drought plots whenever it rained and to remove them when it stopped. The water collected by the curtains was removed from the area by gutters;
- to keep the hydrological conditions in the warmed plots unaffected, rain sensor activated the removal of the curtains in case of rain during the night (sensitivity < 0.3 mm). When the rain stopped, the curtains were automatically drawn over the vegetation again.
Twenty m2 plots (5x4 m) were manipulated by night-time warming and extending drought, and the responses to the treatments were compared to the control plots. Each type of manipulation was replicated 3 times. Pre-treatment measures were conducted to identify variability between sites or plots.
In each plot, air temperature and relative humidity at 20 cm height from soil (Igromer HP100A, Rotronic, CH), soil temperature at 10 cm depth and at 20 cm depth (LTN NR3, Tecno.El, IT) and soil water content (ECH2O probe model EC-20, Decagon Devices, Inc.) at 10 cm depth were continuously monitored. Moreover, a weather station measured air temperature and relative humidity (Igromer HP100A, Rotronic, CH), wind direction and speed (03002 Young, USA), global solar radiation (PYR SKS1110, Skye Instruments, UK) and precipitation (ARG199, Environmental Measurements, UK) at 2 m height. The opening and closing time of the curtains were recorded for each plot by sensors (Mac-I) installed along the rails. These sensors allow to check the good working of the curtains and, when compared to the time of raining, to calculate the amount of precipitation removed. All the sensors were acquired and stored in a data logger (CR10X Campbell Scientific, Inc., USA) and downloaded on a laptop every week nearly.
The average temperatures were calculated for the growing season, covering the months from September, when the autumn precipitations occur, till May, before the beginning of the summer dry period.
Plant cover
The pinpoint method ([66], [40]) was used in order to measure plant frequency, and to indirectly estimate plant cover. A sharpened pin was lowered through the vegetation along different transects. Each plant hit with the pin was counted and the plant species and type of contact recorded. 300 measurements for each plot were collected. Two different contact types were identified: vegetative parts (green and dead) and reproductive structures (flowers, inflorescences, and fruits). The total number of hits for each species (and for each part of plant) per transect was expressed as portion of all the pin per transect. Pre-treatment measurements were done in summer 2001 to identify variability between plots. The measurement frequency was yearly, during the first two weeks of May, at the peak of the growing season, except in 2001 when the campaign was done in June.
Plant biomass
Plant dimensions were calibrated against woody biomass using destructive sampling outside the plots. In winter 2001 inside an external plot (5x5 m) the maximum height (H) and the two orthogonal maximum crown diameters (Dc1 and Dc2) were taken for the following perennial plants (C. monspeliensis, H. italicum, D. pentaphyllum, P. lentiscus and C. creticus). The plants were then harvested. The collected material was dried at 70 °C up to constant weight and then weighed to measure the dry woody biomass (DWB). Given the projected crown area (Ac) equal to (eqn. 1):
The apparent volume (Va) of each plant was calculated as (eqn. 2):
Five different relationships for the i species (i= 5) between the Va and DWB were found (eqn. 3):
where a is the estimated coefficient. The apparent volume has been widely used to determine the aerial biomass in polycormic plants of the Mediterranean region ([62], [69]).
In early spring 2002 and 2004, H and Dc of the perennial species were measured inside the nine experimental plots, and the standing plant woody biomass was estimated by the eqn. 3.
The annual growth rate (AGR) was calculated as (eqn. 4):
where 2004 and 2002 represent the sampling years.
Litterfall
In each plot, 10 litterfall collectors were located under the canopy of plants according to their cover. Each collector was made of PVC tubes with a circular area of 385 cm2 and 20 cm high. The bottom of the collector was closed with a net (0.25 mm2) to prevent material loss. The material was collected, dried in oven and weighed up to constant weight almost monthly.
Plant shoots growth
Direct measurements of plant growth were carried out for the most dominant species (C. monspeliensis). At the beginning of the vegetative cycle (September), every year a sample of 10 shoots was labelled in each plot. The shoots were harvested in March when maximum elongation of terminal main shoots was reached and flower buds appeared at the apex of shoots. For each shoot the following parameters were measured: length of the main shoot, orthogonal basal diameters, number of leaves, and leaf area.
Leaf chemical composition
Leaves of upper-canopy were sampled every year to determine leaf chemical composition. C and N concentrations were determined by elemental analyzer (Carlo Erba model 1108EA, Milan, IT). P concentration was determined by colorimetry using the blu-molibdathe method described in Allen ([3]).
Predawn water potential
Xilematic pre-dawn water potential was measured from April 2002 to August 2004 every two months on C. monspeliensis by pressure chamber (model SKPM 1400, Skye Instruments LTD, UK). Before dawn, one shoot per plant was cut after enclosure in a plastic bag and measured in one minute. Three plants of for each plot were measured in each sampling date, for a total of 27 measurements.
Statistical analysis
The effect of warming and drought treatments on the air and soil temperatures was tested by a Wilcoxon signed rank test. We tested if the daily temperature differences (warming minus control and drought minus control, respectively) could be assumed to be higher than zero.
One-way ANOVAs with plant variables as dependent variable and treatment as independent factor was performed for each year (or date) to compare warming and drought treatments separately with control treatment. We considered the plot as the smallest independent unit; therefore we used the average value of each variable per plot (n = 3 per treatment), except for shoots. Significant differences between means were identified by Duncan’s multiple range test.
The plant cover results relative to the whole community and to the three dominant species (C. monspeliensis, H. italicum and D. pentaphyllum) were subjected to regression analysis in order to describe, for each treatment, the trend of this variable during the experiment. Significant differences between coefficient slope relative to control and each treatment were identified by Student’s test.
Leaf chemical composition differences were tested by a two-way ANOVA. The mean value per plot for each nutrient (n = 3 per treatment) was used as dependent variable. Nutrients and species were analyzed separately. Year (n = 4) and treatment (n = 3) were the independent variables.
Results and discussion
Effect of the treatments on microclimate
The warming treatment increased significantly soil and air temperature during the three growing seasons (Tab. 1). The effects on the minimum air temperatures were on average +0.9 °C, +0.6 °C and +0.4 °C, measured respectively at +20 cm (Ta), -10 cm (Ts10) and -20 cm (Ts20). As a consequence of the higher minimum temperatures, the daily mean temperatures were significantly affected as well: the strongest effect was on Ta (+0.3 °C); the effect on Ts10 was positive in 2001-2002, but in 2002-2003 and 2003-2004 it was almost null; Ts20 was warmer in 2001-2002 and 2003-2004, and it was colder in 2002-2003.
Tab. 1 - (a) Growing season (September-May) daily mean temperatures of air (Ta), soil at 10 cm depth (Ts10) and soil at 20 cm depth (Ts20); (b) Growing season (September-May) daily mean minimum temperatures of air (Ta), soil at 10 cm depth (Ts10) and soil at 20 cm depth (Ts20). The following symbols indicate significant differences between the warming treatment and the control: *** p < 0.001;[a] p < 0.06.
(a) Year |
Ta | Ts10 | Ts20 | ||||||
---|---|---|---|---|---|---|---|---|---|
Control (°C) |
Warming (°C) |
Effect (%) |
Control (°C) |
Warming (°C) |
Effect (%) |
Control (°C) |
Warming (°C) |
Effect (%) |
|
2001-2002 | 14 | 14 | +2.2*** | 14 | 15 | +3.9*** | 15 | 16 | +2.7*** |
2002-2003 | 13 | 14 | +1.9*** | 14 | 14 | -0.2[a] | 15 | 15 | -2.2*** |
2003-2004 | 13 | 13 | +3.6*** | 14 | 14 | +0.8*** | 14 | 15 | +1.4*** |
(b) Year |
Ta (min) |
Ts10 (min) |
Ts20 (min) |
||||||
Control (°C) |
Warming (°C) |
Effect (%) |
Control (°C) |
Warming (°C) |
Effect (%) |
Control (°C) |
Warming (°C) |
Effect (%) |
|
2001-2002 | 8 | 9 | +9.8*** | 13 | 14 | +6.7*** | 14 | 15 | +5.0*** |
2002-2003 | 6 | 7 | +14.7*** | 12 | 12 | +3.7*** | 13 | 13 | +1.5*** |
2003-2004 | 7 | 8 | +12.4*** | 12 | 13 | +4.1*** | 13 | 14 | +2.1*** |
The removed precipitation amounts (sum of the two periods - Tab. 2), expressed as percentage of the total precipitation fallen during the growing season, were 6%, 18% and 46% respectively in 2001-2002, 2002-2003 and 2003-2004. The system was not always effective in cutting rain, as occurred during the autumn of the first growing season and during the spring of the second growing season.
Tab. 2 - Mean annual biomass accumulation (g m-2 yr-1) calculated during the period 2002-2004. CM = Cistus monspeliensis; CC = C. creticus; PL = Pistacia lentiscus; HM = Helichrysum italicum; DP = Dorycnium pentaphyllum; TOT = above ground biomass growth of the total biomass. D.OFF = period with the Drought treatment turned off.
Growing season | Period | Day of Drought treatment |
Precipitation (mm) | Mean RWC (%) | ||||
---|---|---|---|---|---|---|---|---|
Control | Drought | Removed rain (%) |
Control | Drought | Effect (%) |
|||
2001-2002 | 1st period | 61 | 1 | 1 | 0 | 21 | 15 | -31 |
2nd period | 30 | 38 | 24 | -35 | 22 | 16 | -29 | |
D.OFF | - | 199 | 199 | - | 57 | 59 | 4 | |
annual | 91 | 238 | 224 | -6 | 45 | 44 | -2 | |
2002-2003 | 1st period | 76 | 152 | 80 | -47 | 26 | 16 | -38 |
2nd period | 54 | 9 | 8 | -7 | 18 | 21 | 14 | |
D.OFF | - | 252 | 252 | - | 71 | 65 | -8 | |
annual | 130 | 412 | 339 | -18 | 48 | 43 | -11 | |
2003-2004 | 1st period | 60 | 178 | 31 | -83 | 59 | 56 | -5 |
2nd period | 61 | 146 | 57 | -61 | 51 | 37 | -27 | |
D.OFF | - | 188 | 188 | - | 58 | 62 | 7 | |
annual | 121 | 513 | 276 | -46 | 57 | 55 | -3 |
The effect of reduced precipitations on soil water content was not always evident, because of the high heterogeneity of soil, and because of the low number of sampling points per plot ([42]). Mean soil relative water contents (normalized to the annual maximum soil water content) during the drought were decreased by 2% (t value = -1.5, p = 0.13), 8% (t value = -7.2, p < 0.001) and 3% (t value = -2.7, p < 0.01), respectively in 2001-2002, 2002-2003 and 2003-2004.
As previously reported in De Angelis et al. ([19]), the obtained change in microclimate was not of the same magnitude compared to other climate manipulative experiments ([31], [57], [56], [43]). Nevertheless, this was the tribute to pay to obtain one of the main objective of the used device, i.e. avoiding the secondary effects of devices as OTCs, electrical wires and infrared lamps ([55], [71], [67], [63], [59], [44], [29]), which included interaction with water cycle and undifferentiated increase in day- and night-time temperatures. On the other hand, our microclimate manipulation was realistic because it altered meanly night-time temperature and prolonged the summer dry period, that is what the instrumental records had revealed for the last decades ([25], [22]). Additionally, Kundzewicz et al. ([35]) report for the future an increase in air temperature by 0.1-0.4 °C per decade and a change in summer precipitations by -5% per decade, so that the simulated climate, obtained by the described devices, and the below reported plant responses, seem to mirror in a realistic way the situation in the short or medium-term of 10-50 years.
Change in plant cover
Pre-treatment measurements (late spring 2001) did not show significant differences between treatments in term of percentage of cover and reproductive effort. C. monspeliensis, H. italicum and D. pentaphyllum provided almost 60% of soil cover; about 21% of soil was covered by other species, the main of which was P. lentiscus and C. creticus; about one fifth of soil was classified as bare soil (Fig. 2).
Fig. 2 - Soil cover percentage by the different species at the pre-treatment (2001). The values are the average of the nine plots.
A general increase of vegetation cover was observed in the whole community during the three years of experimentation, even if the increase of this parameter in warming and drought treatment was almost null in 2003. This positive temporal pattern was mainly observed in control and warming treatment (p = 0.01 and 0.07 in control and warming respectively) whereas in the drought treatment it was less evident (p = 0.14 - Fig. 3A). No significant differences between regression slopes were observed.
Fig. 3 - Trend line of cover percentage observed: (a) for the whole community, and (b) for Cistus monspeliensis in treated and non-treated plots during the years of experimentation. Full circles with continuous line = Control; full triangles with dash line = Warming; open squares with dot line = Drought.
At species-specific level, a clear negative effect of drought treatment was observed for C. monspeliensis percentage cover. The trend line, in fact, showed a negative slope significantly different from the one of control, (p<0.05 - Fig. 3B). No particular effect of warming was observed on this species. On the contrary, H. italicum and D. pentaphyllum did not show any identifiable temporal trend either in treated or in non-treated plots (data not shown).
The positive temporal trend of the total cover observed in the control and warming treatment, except that in the drought ones (Fig. 3A) seems to indicate that the evolutionary process of plant community has not stopped after 12 years (after the last disturbance in 1993) since the fire-break strip has no longer been managed. This behaviour is similar to those reported by other studies ([10]), where plant cover had been recovered in 12-15 years after the disturbances. However, our climate and edaphic conditions could explain the quite slow evolution of the vegetation community.
The negative trend observed for the Cistus cover in the drought treatment (Fig. 3B) gives evidence to the negative effect of water shortage on the evolution of this community. This idea is corroborated by the fact that in 2001 (pre-treatment) we had observed an inverse relationship between plant cover of Cistus and Helichrysum in the nine plots; therefore it could be plausible to think of an exclusive use of space. Moreover, the carbon isotopic composition of leaves (as reported in [20]) had demonstrated that Cistus plants were negatively influenced in their stomatal response by the drought treatment, effect not observed in Helichrysum. If the negative trend in Cistus cover is confirmed in the future, we could assert that the evolutionary phases of this ecosystem will be blocked and, on the contrary, the less evolved steps will find enough space and resources to expand. Considering that the cycles of plant adaptation and recovery after disturbances are longer than the 4 years of the present study ([31], [24], [10], [65]), a longer-term experiment could give more clear responses about the behaviour of plants at specific and functional group level.
Effects on plant growth and litterfall
In 2002 the estimated above ground standing woody biomass inside the experimental plots varied between 328 and 545 g m-2. On average 72% of this amount was due to the sum of C. monspeliensis and H. italicum biomasses. D. pentaphyllum, C. creticus and P. lentiscus contributed on average with 19%, 6% and 4% respectively.
In the control treatment the annual growth rate (AGR; Fig. 4) for the period 2002-2004 was 27%; in the warming treatment it was 34% and in the drought treatment it was 27%, not significantly different between the treated and non-treated plots. In terms of biomass, this is equivalent to an increase of only about 60 g m-2 yr-1 of woody dry weight, averaged between the two years (Tab. 3).
Fig. 4 - Annual growth rate calculated in the period 2002-2004. Control = Black bars; Warming = Grey bars; Drought = White bars. CM = Cistus monspeliensis; CC = C. creticus; PL = Pistacia lentiscus; HM = Helichrysum italicum; DP = Dorycnium pentaphyllum; TOT = above ground biomass growth rate of the total biomass.
Tab. 3 - Parameters of the allometric regression DWBi = a i * Vai used to estimate the aerial biomass of the five mentioned species.
Species | a | n | r2 | p |
---|---|---|---|---|
C. monspeliensis | 1311.8 ± 76.2 | 12 | 0 | p<0.001 |
H. italicum | 2364.4 ± 243.7 | 24 | 0 | p<0.001 |
P. lentiscus | 2364.4 ± 243.7 | 4 | 0 | p<0.01 |
D. pentaphyllum | 577.6 ± 17.4 | 11 | 0 | p<0.01 |
C. incanus | 2969.1 ± 437.4 | 13 | 0 | p<0.01 |
Shoots basal diameter of C. monspeliensis was not significantly affected by the warming treatment, whereas the drought treatment inhibited significantly this parameter during the growing seasons 2001-2002 and 2003-2004 (-15% and -10% respectively, p < 0.05), but not in the 2002-2003 growing season (-6%, p = 0.4 - Tab. 4).
Tab. 4 - Annual biomass accumulation (g m-2 yr-1) calculated during the period 2002-2004. [a] = above ground biomass growth of the total biomass.
g m-2 yr-1 | Control | Warming | Drought |
---|---|---|---|
Cistus monspeliensis | 13.7 (± 5.3) | 21.5 (± 5.2) | 22.7 (± 16.5) |
C. creticus | 4.7 (± 3.1) | 0.7 (± 0.3) | 2.9 (± 1.1) |
Pistacia lentiscus | 0.6 (± 0.5) | 0.9 (± 0.4) | 5.0 (--) |
Helichrysum italicum | 36.3 (± 18.1) | 28.8 (± 17.1) | 21.3 (± 13.0) |
Dorycnium pentaphyllum | 6.0 (± 3.2) | 11.6 (± 6.1) | 8.0 (± 2.0) |
Total[a] | 61.2 (± 23.2) | 63.5 (± 7.4) | 56.6 (± 17.3) |
Shoot elongation was not significantly affected by the warming treatment. A significant negative effect of drought treatment was noticed in the 2001-2002 and 2002-2003 growing seasons (-21% in both the year; p < 0.05) but not in 2003-2004 (p = 0.7 - Tab. 4).
The warming treatment did not have effect on the other parameters in the 2002-2003 and 2003-2004 growing seasons. The drought treatment had a negative effect on the mean leaf area in the 2003-2004 growing season (p < 0.05 - Tab. 4).
The observed changes in the shoot growth were caused by a rainfall decrease and by the consequent change in soil water content. These results match with the experimental findings, that derive from studies carried on both in shrublands and maquis species and in conifer forests ([34], [50], [38], [46], [14], [53]) of the Mediterranean basin, which all claimed water availability as the limiting factor for plants of this area and showed that decreased precipitations could have a central role for plant productivity. This experiment, in addition, stress this characteristic, given that the experimentally drought was not a permanent stress, but it was carried out on only during the growing seasons. Moreover, different periods of the drought treatment brought different results on shoot elongation. In fact, the drought treatment in the autumn 2001 and 2002 (growing seasons 2001-2002 and 2003-2004 respectively) removed summer precipitations, because it run from September to October, causing a slightly recover of the soil water content and therefore a stronger effect on shoot elongation, that starts after the dry summer whenever the first rains fall; on the contrary, in the autumn 2003 (growing season 2003-2004) the drought treatment run later (October-November), when soil water recharge had already occurred and the shoot growth had already started. Considering the biomass growth at the community level, no significant effect was found. This is not in contrast with the change in shoot elongation, because shoot elongation is a more precise measure and moreover it is a more sensible parameter than biomass accumulation especially in the short period (in this study we are testing only three growing seasons).
Differently from the drought treatment, warmer temperatures did not bring any positive or negative effect on plant and shoot growth (Tab. 4). In temperate and boreal regions, warming experiments had induced higher plant growth ([54]). In the Mediterranean area, where temperature is already optimal, neither stimulation effect on photosynthesis or on vegetative period lengthening can be plausible ([50]). In this site, in fact, Cesaraccio et al. ([13]) did not find any significant elongation of the vegetative period. [20]) did not report, for the same site, positive effect of the warming treatment on litter decomposition process and on the soil CO2 efflux. In a parallel experiment on a shrubland community in Spain, Llorens et al. ([38]) did not report a significant change of net photosynthesis at higher temperatures.
The leaf litterfall amount calculation was based, as for the biomass, on the growing season, that is to say considering the litter fallen during the period from September of the year n until August of the year n+ 1. In the control treatment (Tab. 5), leaf litterfall was 51.1, 52.0 and 34.2 g m-2 period-1 respectively during the growing seasons 2001-2002, 2002-2003 and 2003-2004. C. monspeliensis produced most of the litter (70-80% of all the collected material). The warming treatment increased the litterfall production by +13%. +2% and +20% respectively in the 2001-2002, 2002-2003 and 2003-2004 growing seasons compared to the control. In the drought plots litterfall production was between 51.6 and 21.6 g m-2 period-1, lower than the control values by 23% 37% in the 2002-2003 and 2003-2004, but the difference was +1% in the 2001-2002. All these effects were never statistically significant. The maximum in the litterfall occurred in summer (June-July), as typical of the drought-avoiding plants with summer-deciduousness habit (Fig. 5), with no differences between the control and the warming and drought treatments. According to several authors ([48], [2], [60]), litter production is strongly controlled by precipitation, following a direct relationship (lower the precipitation, lower the litter production). Moreover, Pavón et al. ([48]) showed that temperature was negatively related to litterfall amount. The lack of treatment effect in the present study was unexpected, the high standard errors of the data are the results of the different percentage distributions of the species inside the plots. However, it is in accordance with the lack of treatments response in the biomass growth and in the number of leaves per shoot (Fig. 4, Tab. 6).
Tab. 5 - Shoot length (± s.e.) and diameter (± s.e.) of C. monspeliensis per year and treatment. Different letters mean significant differences among the treatments (p < 0.05); n.a. = not available.
Growing season |
Treatment | Shoot diameter (mm) |
Shoot length (cm) |
Total leaf area (cm2) |
# leaves shoot-1 |
Mean leaf area (cm2) |
---|---|---|---|---|---|---|
2001-2002 | Control | 2.0 ± 0.11 a | 6.9 ± 0.5 a | n.a. | n.a. | n.a. |
Warming | 1.8 ± 0.07 ab | 6.0 ± 0.3 ab | n.a. | n.a. | n.a. | |
Drought | 1.7 ± 0.05 b | 5.3 ± 0.3 b | n.a. | n.a. | n.a. | |
2002-2003 | Control | 1.8 ± 0.08 a | 7.8 ± 0.5 a | 32.8 ± 3.4 a | 42.6 ± 3.9 a | 0.77 ± 0.04 a |
Warming | 1.9 ± 0.04 a | 7.8 ± 0.3 a | 33.2 ± 3.0 a | 44.0 ± 4.0 a | 0.81 ± 0.07 a | |
Drought | 1.7 ± 0.07 a | 6.2 ± 0.5 b | 28.6 ± 3.3 a | 33.3 ± 2.9 a | 0.85 ± 0.05 a | |
2003-2004 | Control | 2.0 ± 0.06 a | 6.2 ± 0.4 a | 29.1 ± 3.3 ab | 54.7 ± 4.9 a | 0.54 ± 0.04 a |
Warming | 2.0 ± 0.07 a | 7.2 ± 0.5 a | 33.9 ± 2.9 a | 59.1 ± 4.3 a | 0.57 ± 0.02 a | |
Drought | 1.7 ± 0.05 b | 5.7 ± 0.5 a | 20.6 ± 2.1 b | 45.8 ± 4.4 a | 0.41 ± 0.02 a |
Fig. 5 - Monthly leaf litterfall of the entire community. In order to simplify, standard errors are not drawn. Control = full circles with continuous line; Warming = full triangles with long dash line; Drought = open squares with short dash.
Tab. 6 - Leaf litterfall production (± s.e.) during the three growing seasons, expressed as g m-2 period-1. Different letters indicate significant differences (p<0.05) among the treatments per each growing season.
g m-2 period-1 |
Control | Warming | Drought |
---|---|---|---|
2001-2002 | 51.1 (± 12.3) a | 57.7 (± 6.6) a | 51.6 (± 22.1) a |
2002-2003 | 52.0 (± 12.3) a | 52.8 (± 7.5) a | 40.0 (± 9.9) a |
2003-2004 | 34.2 (± 7.0) a | 41.0 (± 2.6) a | 21.6 (± 5.2) a |
Leaf chemical composition
Leaf C concentration was approximately 50% in the analyzed species (Fig. 6 a-b-c, Tab. 7). This value followed a slightly significant decreasing trend in the three species, but no effect due to the treatments was ever noticed.
Fig. 6 - Leaf chemical composition (Carbon, Nitrogen and Phosphorous) of the three main species Cistus monspeliensis (a-d-g), Helichrysum italicum (b-e-h) and Dorycnium pentaphyllum (c-f-i) for the years 2001-2004 (2001 is pre-treatment). Control = Black bars; Warming = Grey bars; Drought = White bars.
Tab. 7 - F-ratios and p-values summary table of the ANOVAs on leaf chemical composition and C/N ratios for the species C. monspeliensis, H. italicum and D. pentaphyllum.
Element | Species | Years | Treatment | Years*Treatment |
---|---|---|---|---|
Carbon | C. monspeliensis | F = 85.02; p<0.001 | F = 2.25; p = 0.13 | F = 1.16; p = 0.36 |
H. italicum | F = 8.88; p<0.01 | F = 0.51; p = 0.61 | F = 0.41; p = 0.86 | |
D. pentaphyllum | F = 5.71; p<0.01 | F = 1.08; p = 0.36 | F = 0.77; p = 0.60 | |
Nitrogen | C. monspeliensis | F = 7.80; p<0.01 | F = 5.82; p<0.01 | F = 0.67; p = 0.68 |
H. italicum | F = 33.10; p<0.001 | F = 0.63; p = 0.55 | F = 0.24; p = 0.96 | |
D. pentaphyllum | F = 83.49; p<0.001 | F = 0.87; p = 0.43 | F = 4.33; p<0.01 | |
Phosphorous | C. monspeliensis | F = 10.42; p<0.001 | F = 4.33; p<0.05 | F = 1.53; p = 0.22 |
H. italicum | F = 0.34; p = 0.79 | F = 1.47; p = 0.26 | F = 2.57; p = 0.06 | |
D. pentaphyllum | F = 2.83; p = 0.07 | F = 0.23; p = 0.80 | F = 0.19; p = 0.98 | |
C/N ratio | C. monspeliensis | F = 24.62; p<0.001 | F = 5.98; p = 0.008 | F = 0.78; p = 0.59 |
H. italicum | F = 60.7; p<0.001 | F = 0.61; p = 0.56 | F = 0.30; p = 0.93 | |
D. pentaphyllum | F = 45.79; p<0.001 | F = 0.57; p = 0.57 | F = 1.95; p = 0.12 |
Leaf N concentration at the pre-treatment was between 1.2-2.2%, with D. pentahyllum the richest species (Fig. 6 d-e-f, Tab. 7). In Cistus leaves a significant higher value of the mean N concentration in the drought than in the control treatment (about +10%) was found. The warming treatment did not alter significantly the leaf C content in all the species. During the study period, N concentration followed an increasing temporal trend in all the species.
P concentration varied among the species between 82 and 92 µg per g of leaf dry weight (Fig. 6 g-h-i, Tab. 7). In Cistus there was a significant higher concentration of P in the drought than in the control (about +60%). The warming treatment did not alter significantly the leaf P content in all the species. Along the years, we observed a decreasing trend of leaf P concentration in Cistus but not in Helichrysum and Dorycnium, independently of the treatments.
In the three species the C:N ratio followed a significant decreasing trend (Tab. 7), with a significant negative drought effect (about -5%) on the average value in Cistus.
Nutrient concentration is the result of the balance between uptake and growth. The observed increase of N and P concentrations in the drought treatment in Cistus can be explained by the reduced shoot growth induced by the water shortage that we had observed in the same treatment. The absence of a concentration effect on the other two species could be the signal of the different behaviour with regard to a drier climate, and therefore could be a symptom of future change in species composition, as also partially seen in plant cover (Fig. 3).
It is worth to say that over time foliar N tended to increase and foliar P to decrease (although strictly to Cistus) in the control treatment (that is to say in natural conditions). The resulting increase in the N:P ratio highlights a progressive importance of P limitation ([50]; [57]), and this pattern could influence its evolution. On the other hand, a decrease in the C:N ratio (more N available for decomposers) can lead to a better litter palatability and to a more rapid litter decomposition process ([18]), with positive consequences on nutrients’ cycles. The observed reduced C:N in the drier conditions can be an alarm because, if a quicker decomposition is not accompanied by an increased uptake (as it is likely to occur given the decrease in plant cover in the drought treatment) nutrient leaching can strongly limit this ecosystem ([12], [39]).
Predawn water potential
In the control treatment, predawn water potential (PWP) of Cistus shoots varied between -0.4 and -3.5 MPa (Fig. 7), similar to values found in another study investigating a different Cistus species ([8]). The lowest values occurred during summer period, except in October 2003, because of the dryness of that year. The warming treatment did not affect the PWP. The warming treatment did not affected the PWP, showing a -9% difference with the control, not statistically significant. The drought treatment negatively affected PWP at three sampling dates, when the drought treatment was running and during the season with enough precipitation, and not in the very dry spring 2003, when only about 50 mm of rain fell. On average, the percentage drought difference was -11%, not statistically significant from the control.
Fig. 7 - Comparison of: (a) control vs. warming and (b) control vs. drought predawn water potentials measured on shoots of Cistus monspeliensis in different dates. The symbol * indicates significant differences (p < 0.05) among the treatments. Control = full circle with continuous line; Warming = full triangles with long dash line; Drought =open squares with short dash. The darkened areas show the period with the drought treatment running.
These results point out that Cistus is stressed under drier conditions and therefore it can perform less C accumulation and growth ([14], [1], [37]), as also confirmed by the decreased elongation of its shoots. The drought treatment decreased PWP in the short term (when the treatment was active), while this effect was absent during the rest of the year. Therefore, Cistus plants were able to recover after the removal of the stress factor. This characteristic is due to its shallow rooting depth and to the persistence of its over-summer leaves which allow it to re-establish its water potentials on the occasion of rain events occurring after the dry season ([72], [28]).
Conclusion
Although not dramatically, the microclimate manipulation altered the microclimate according to common scenarios ([35], [16], [17]), giving a possible image of the climate in the short and medium term and the possible responses of this shrubland community.
The drought treatment influenced the changes of Cistus cover, differently from what happened to that of Helichrysum, in addition to the effects on shoot elongation in Cistus. This different behaviour could be the sign of a possible change in species composition with possible consequences on the evolution of this community. A new community structure could imply different processes at soil ([30]) and plant level ([4]). We underline the need of long term observation, because of the different responses of plants in the short- and long-term ([50]). The lack of a warming effect on the measured variables, could be the results of the low increase of temperature due to the manipulation, especially during the diurnal hours ([38]) but stresses also the central role of water availability for plants of the Mediterranean region.
Acknowledgments
The research was funded by the EU under the project VULCAN (contract No. EVK2-CT-2000-00094). We would like to thank Mr. Tullio Oro for the fieldwork when all the arrangements were installed; all the PhD students of the University of Sassari for the continuous monitoring of the area and a special thank to the Porto Conte staff.
References
Gscholar
Gscholar
Gscholar
Gscholar
Gscholar
Gscholar
Gscholar
Gscholar
Gscholar
Gscholar
Online | Gscholar
Gscholar
Gscholar
Gscholar
Gscholar
Gscholar
Gscholar
Gscholar
Authors’ Info
Authors’ Affiliation
P De Angelis
Department of Forest Environment and Resources, University of Tuscia, via S. Camillo De Lellis snc - 01100 Viterbo (Italy)
C Cesaraccio
P Duce
National Research Council Institute of Biometeorology, Via Funtana di Lu Colbu, 4/A - 07100 Sassari (Italy)
National Research Council Institute of Agro-Environmental and Forest Biology, c/o Area di Ricerca, Via Salaria km. 29.3 - c.p. 10 - 00016 Monterotondo Scalo, Roma (Italy)
Corresponding author
Paper Info
Citation
De Dato G, Pellizzaro G, Cesaraccio C, Sirca C, De Angelis P, Duce P, Spano D, Scarascia Mugnozza G (2008). Effects of warmer and drier climate conditions on plant composition and biomass production in a Mediterranean shrubland community. iForest 1: 39-48. - doi: 10.3832/ifor0418-0010039
Paper history
Received: Jun 29, 2006
Accepted: Nov 17, 2006
First online: Feb 28, 2008
Publication Date: Feb 28, 2008
Publication Time: 15.60 months
Copyright Information
© SISEF - The Italian Society of Silviculture and Forest Ecology 2008
Open Access
This article is distributed under the terms of the Creative Commons Attribution-Non Commercial 4.0 International (https://creativecommons.org/licenses/by-nc/4.0/), which permits unrestricted use, distribution, and reproduction in any medium, provided you give appropriate credit to the original author(s) and the source, provide a link to the Creative Commons license, and indicate if changes were made.
Web Metrics
Breakdown by View Type
Article Usage
Total Article Views: 60236
(from publication date up to now)
Breakdown by View Type
HTML Page Views: 49896
Abstract Page Views: 3465
PDF Downloads: 5887
Citation/Reference Downloads: 77
XML Downloads: 911
Web Metrics
Days since publication: 6111
Overall contacts: 60236
Avg. contacts per week: 69.00
Article Citations
Article citations are based on data periodically collected from the Clarivate Web of Science web site
(last update: Feb 2023)
Total number of cites (since 2008): 38
Average cites per year: 2.38
Publication Metrics
by Dimensions ©
Articles citing this article
List of the papers citing this article based on CrossRef Cited-by.
Related Contents
iForest Similar Articles
Review Papers
Linking patterns of forest dieback to triggering climatic and weather events: an overview on Mediterranean forests
vol. 17, pp. 309-316 (online: 30 September 2024)
Research Articles
Controlling soil total nitrogen factors across shrublands in the Three Rivers Source Region of the Tibetan Plateau
vol. 13, pp. 559-565 (online: 29 November 2020)
Review Papers
Wood anatomy of boreal species in a warming world: a review
vol. 13, pp. 130-138 (online: 09 April 2020)
Research Articles
Evergreen species response to Mediterranean climate stress factors
vol. 9, pp. 946-953 (online: 07 July 2016)
Research Articles
Perceptions of forest experts on climate change and fire management in European Mediterranean forests
vol. 7, pp. 33-41 (online: 14 October 2013)
Review Papers
Quantifying and modeling water availability in temperate forests: a review of drought and aridity indices
vol. 12, pp. 1-16 (online: 10 January 2019)
Review Papers
Climate change impacts on spatial distribution, tree-ring growth, and water use of stone pine (Pinus pinea L.) forests in the Mediterranean region and silvicultural practices to limit those impacts
vol. 14, pp. 104-112 (online: 01 March 2021)
Research Articles
Contrasting holm oak provenances show different field performance but similar resilience to drought events eight years after planting in a Mediterranean environment
vol. 11, pp. 259-266 (online: 29 March 2018)
Review Papers
SilvaCuore: a web-application to monitor the health status of Italian forests
vol. 17, pp. 317-322 (online: 30 September 2024)
Research Articles
Day and night respiration of three tree species in a temperate forest of northeastern China
vol. 8, pp. 25-32 (online: 26 May 2014)
iForest Database Search
Search By Author
Search By Keyword
Google Scholar Search
Citing Articles
Search By Author
Search By Keywords
PubMed Search
Search By Author
Search By Keyword