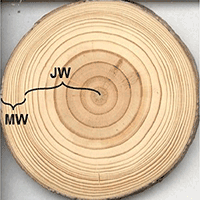
Wood anatomy of boreal species in a warming world: a review
iForest - Biogeosciences and Forestry, Volume 13, Issue 2, Pages 130-138 (2020)
doi: https://doi.org/10.3832/ifor3230-013
Published: Apr 09, 2020 - Copyright © 2020 SISEF
Review Papers
Abstract
Global warming is affecting tree growth and forest productivity, especially in the Northern boreal ecosystems. Wood quality, which is largely determined by anatomical traits of wood, is vital for the forest industry and global carbon sequestration. Cambium activity, wood density, fiber length and microfibril angle are the anatomical traits that determine wood quality, depending on market demands. Within the global warming scenario, a comprehensive understanding of these traits is still lacking and urgently required for both the forest industries and ecological researches. In this review, we identify that large proportions of mature wood, high wood density, longer fiber or tracheid length and low microfibril angles are the anatomical traits closely related with high wood quality. Higher temperatures could trigger onset and ending of cambial cell division, thus affecting wood quality by modulating duration of the growing season. Climate warming could also affect wood quality by impacting earlywood and latewood formation, as well as changing wood density, fiber length and microfibril angle depending on different species and growing conditions. In addition, this review indicates that the anatomical traits involved in wood quality are diverse and depend on the intended use. Improving our knowledge about the underlying mechanisms of how the wood anatomical traits respond to a changing environment with extreme climate events is thus still a crucial topic in the forest sciences. Selection of species and provenances best adapted to climate warming will be necessary to improve quality without sacrificing volume. Studies on wood traits and their relation to climate should therefore focus on a multitude of aspects including the physiology and genetics of boreal tree species.
Keywords
Climate Warming, Earlywood, Fiber Length, Latewood, Microfibril Angle, Radial Growth, Wood Formation
Introduction
Global mean surface temperature has risen by 0.85 °C during the last century (1880-2012) and is predicted to increase further in the next decades ([53]). Forests in the boreal zone, where climate change has the strongest effects over the world, are one of the ecosystems most responsive to temperature ([53]). In response to recent climate warming, boreal trees have shown advances in primary growth, such as earlier shooting, leafing, and flowering ([57], [98]), as well as the corresponding secondary growth, such as earlier xylem cell production and differentiation ([81], [10]). By the end of this century, boreal trees are expected to still benefit from future warming to enhance their primary and secondary growth, as shown by recent modeling studies ([67]), but reduced water availability may reverse this beneficial effect ([24]). Overall climate warming influences the quality and quantity of boreal forest wood supply by impacting growth and mortality and by creating shifts in species composition ([12]) Exogenous (e.g., climate and disturbances) and genetic factors determine primary and secondary tree growth. When combined with species competition, these two factors determine the form of a tree, and ultimately the quality of its wood.
The boreal forest, which is distributed over high latitude regions of Asia, Europe and North America, and mostly in Russia, Canada, the state of Alaska (USA), Finland, and Sweden, encompasses more than 25% of the global forest area (i.e., almost 1 billion hectares - [44]). It is dominated by coniferous species accompanied by varying proportions of angiosperms ([44]). Wood that is utilized in the forest industries in these countries is mainly extracted from boreal forests. For instance, more than 33% of the lumber and 25% of paper on the export market are generated from the boreal forests ([13]). The boreal forest also plays multiple important roles in regulating climate, biogenic carbon, and carbon sequestration ([11], [86]). Therefore, the fate of wood in the boreal forest under a warming scenario is an urgent global concern.
Wood quality is not an absolute measure in the literature because it is largely dependent on the intended practical application, such as structural board and lumber or paper making. Here, we define wood quality according to the timber resources needed to meet the requirements of forest industries and the end product based on tree morphological (e.g., knots and their size and distribution), wood anatomical (e.g., ring growth, earlywood and latewood, density, fiber or tracheid length, microfibril angle), chemical (e.g., cellulose, hemicellulose, lignin), and physical (e.g., stiffness, strength) properties ([50]). Wood quality is an important issue for the boreal forest industry because the traditional target of wood volume maximization can be achieved only occasionally. Boreal conifers typically have a short growing season, live in harsh conditions, and need more time than temperate and tropical trees to reach maturity and similar volumes of wood. Management decisions based on an increased awareness of biodiversity conservation may also limit the quantity of harvested wood.
In addition, increasingly competitive market pressure is pushing forest industries to shift their focus from volume to quality of wood ([95]). Therefore, high quality wood is now one of the main aims to satisfy both market demands and sustainable forest development. From the perspective of wood anatomy, high quality wood is characterized by large proportions of mature wood, associated with high wood gravity or density, long fiber or tracheid length, thick secondary wall with low microfibril angles ([73], [50]). From an ecological point of view, high wood quality means that more carbon is sequestered in trees because the above-ground carbon assessment is mainly based on both xylem volume and density and their variations over time and space ([20], [38]). It is critical to strengthen the role of the boreal forest in sequestering carbon and to mitigate the increased atmospheric CO2 because less carbon seems to be accumulated than expected by the available models ([64]).
The anatomical traits that determine wood quality are controlled by a synergic effect of genetics and environment ([33], [32]). A considerable number of studies on wood properties and quality have been conducted in the boreal forest. Overall, they can be summarized into the following three aspects: (i) investigations on tree morphological, wood anatomical, physical/ mechanical properties, and their interactions ([9], [52]); (ii) breeding programs for tree selection and improvement ([99]); (iii) silvicultural practices such as thinning, spacing, and fertilization ([16], [76]). The effect of climate change on wood quality is known and studied only partially, due to several reasons. Wood market demands and forest industries require a better understanding of basic wood properties such as juvenile and mature wood, average density, earlywood and latewood proportion, fiber/tracheid length and microfibril angle. The study of the response of these traits to climate change is often neglected because the emphasis is on quantity and quality improvement of trees through management and breeding practices ([48], [82]). Many studies focus on tree genetics and wood traits ([9], [78]), because wood properties are partly controlled by genetics ([58]). In addition, evaluating the effects of climate change on wood quality is a long-term effort, which can hardly be integrated into the current rapid, effective tree growth improvement programs through silviculture and breeding, thus reducing interest in exploring effects of climate on wood quality ([30]). However, it is obvious that climate is affecting wood quality directly or indirectly by influencing primary- and secondary-growth as well as other wood quality-related factors like insect disturbances and competition ([52]). We expect that these effects of climate on wood quality will be intensified with the ongoing global warming.
For sustainable forest development, it is critical to address why and how climate affects wood quality in the boreal forest. In this paper, we aim to answer these questions by reviewing the literature on the mechanisms behind the effect of climate warming on wood traits. We focus on boreal softwood forest and studies using phenology, dendrochronology and wood anatomy in relation to wood properties and quality. This review includes (i) the anatomical traits affecting wood quality, (ii) the effect of warming on these traits, and (iii) the perspectives and proposals for future research.
Study area and species
This literature review focuses on boreal softwood species such as jack pine, black spruce, white spruce, larch and balsam fir. We include studies on these species in their natural environment or in controlled experiments.
Selection of anatomical traits that determine wood quality
Wood anatomical traits related to wood quality mainly include radial growth, wood density, fiber or tracheid length, and microfibril angle ([8]). These anatomical traits are the basis for the mechanical properties: bending, stiffness (modulus of elasticity, MOE), strength (modulus of rupture, MOR), and shrinkage behavior, which are the most important physical features to describe wood quality ([7], [49]). For example, theoretical ([19]) and experimental ([35], [49]) evidences have shown that anatomical traits such as density and microfibril angle are the main determinants for wood stiffness and strength. These anatomical traits are generally believed to be directly or indirectly affected by climate ([51]). Therefore, wood anatomical traits can be regarded as the bridge between wood quality and climate, and it is necessary to address these properties to cast light on the mechanisms behind the effect of climate change on wood quality. The anatomical traits considered in this review are radial growth, wood density, fiber length and microfibril angle.
Wood anatomy and climate warming
The anatomical traits that determine wood quality are regulated by environmental factors that alter the final expression of genes ([57]). Climate warming, which is the most critical ongoing environmental change, is impacting carbon sequestration and wood production in the forest industry by affecting these anatomical traits in trees. Therefore, the effect of warming on anatomical traits urgently needs to be evaluated and discussed.
Radial growth
Radial growth in terms of stem volume achieved over a rotation is often considered as one of the important wood quality traits by commercial forestry ([32]). The average growth increment in diameter, represented by the annual tree-ring, is defined as rate of radial growth, representing the volume of wood, which is the objective of the forest industry ([75]).
Wood, the result of the production of xylem cells by cambium, is divided into juvenile (generally including the xylem formed in the first 15-20 years) and mature (formed later) wood, which refer to the regions that are close to and further from the pith, respectively ([73], see also [54] for more details about juvenile and mature-wood distribution). The quality of juvenile and mature wood and their proportions in a tree stem largely represent and determine the wood quality. Compared with mature wood, juvenile wood often has lower density and larger microfibril angle, which could cause inferior quality (e.g., low tensile and tear strength - [73]). From the perspective of the forestry industry, a large proportion of mature wood in a stem usually indicates good quality and can meet varied wood demands. Wood quality is therefore largely determined by the radial growth represented by growth rate and the proportions of juvenile and mature wood.
Radial growth is the result of both endogenous (e.g., physiological, genetic factors) and exogenous factors such as climate, of which environmental factors are recognized to play a more important role than genetics ([33], [58] - Fig. 1). For instance, traits related to the annual diameter growth of white spruce, including ring width and the proportion of earlywood and latewood were reported to be strongly controlled by environmental rather than genetic influences ([58]). The theoretical basis of tree-ring research summarized by Fritts ([40]) assumes that ring growth contains climatic information of both the previous and current growing season. Radial growth has thus been used as a reliable proxy to explore historical climate variability and change in dendroclimatology. However, it is less clear how traits of wood quality such as earlywood and latewood width, and the corresponding density are associated with temperature. In this section, we further discuss this issue from the perspective of xylem formation process.
Fig. 1 - Schematic diagram of factors (physiological and environmental) influencing wood formation, and annual diameter growth. JW, MW, EW and LW denote juvenile wood, mature wood, earlywood and latewood, respectively.
From dormancy to the onset of cambial cell division
It is well known that trees in temperate and boreal regions have annual pulses of activity and dormancy. In late autumn, trees undergo dormancy, including a rest phase that is controlled by internal factors, and a quiescence phase that is maintained by unfavorable environmental conditions ([41], [81]). Throughout this period, low temperatures play a vital role in regulating tree physiological activities through chilling (exposure to cold), which is necessary for trees to enter the quiescence phase. Throughout this phase, trees could regain the ability to respond to growth-promoting conditions. Then, exposure to rising temperatures, associated to a lengthening of photoperiod, make trees capable of starting cambial cell differentiation in spring ([37]). Photoperiod is also involved in driving the rate of cambial activity. In cold climates, cell production mostly occurs close to the summer solstice, and reduces under decreasing day length ([80], [23]). Thus, photoperiod could play an important role in combination with other endogenous and environmental factors in triggering the end of growth ([63], [85]). However, while temperature is expected to change under future climate, photoperiod remains the stable astronomical signal for plants. In spring, a threshold temperature might be needed to trigger the onset of cambial cell division ([81]) because this thermal threshold may affect the cambial sensitivity to auxin (indole-3-acetic acid, IAA). Therefore, changing temperatures could impact wood formation and thus the quality of wood by modulating the onset of cambium cell division.
Cytokinins, supplied by root apices ([2]) and produced only under favorable environmental conditions in spring, are the central regulators of cambial activity and may interact with the cambial responses to auxin ([3]). Therefore, the earlier the threshold temperature is reached in spring, the earlier the initiation of xylem cell division starts. Consequently, under warmer springs, a longer growing season could be expected for radial growth and wood formation ([28], [98]). Although it is acknowledged that a threshold temperature in spring may trigger the onset of stem cambial cell division, there is still a lack of consensus in the literature. This might be due to different species and sites. Recent wood anatomical studies using a weekly sampling to monitor xylem formation found that a temperature threshold in spring is critical for the onset of xylem formation in boreal trees ([27], [77]). However, this threshold temperature varies across studies. For instance, Schmitt et al. ([83]) documented that growth at the tree-line occurred when the daily temperature was above 5 °C and Rossi et al. ([81]) reported that xylogenesis was active when the mean daily air temperature was 5.6-8.5 °C. In northwestern Quebec, Zhai et al. ([98]) found that weekly mean minimum air temperature for the onset of new xylem cell production of trembling aspen and white birch ranged between 8.2 and 10.4 °C.
The process of xylem cell formation
Xylogenesis is initiated by cell division in the vascular cambium, followed by differentiation of cambial derivatives ([73]). Overall, cell division resulting in xylem formation in temperate and boreal trees is characterized by an S-shaped curve (see [51] for more details about S-shaped curve), which involves a slow initiation period, a fast-growing period followed by a slow termination period ([81], [51], [98]). This S-shaped curve is the result of an interaction of physiological and environmental factors. It describes the dynamics of wood formation in the growing season. During wood formation, number and size of xylem cells determine tree-ring width and earlywood and latewood proportions, and, together with cell wall thickness, wood density ([5]). Thus, any temperature-induced change of wood formation dynamics could influence wood quality in a tree ([88]).
The wood structure, originating from xylem formation, is a long-term adaptation strategy of trees to the local environment, particularly their water transport capacity ([36]). Xylem structure is generally believed to reflect the functional balance between efficient water transport to achieve optimal growth, minimum investment of construction costs to secure the xylem plumbing system, mechanical support of the assimilating leaves, and storage of water and nonstructural carbohydrates for defense and resistance to stress ([92]). A recent wood anatomical study showed that during the xylem formation process, water is the first constraint in modulating the production of xylem by the cambial cells in the growing season, while carbon is secondary because xylem cell expansion is a turgor-pressure driven process ([26]). Even when trees are submitted to water stress, mechanical factors may drastically impact secondary growth ([70]). Under water deficit, cambium stops dividing to limit the number of cells locked in differentiation without enough water ([26]). This may explain the widely observed growth reduction of trees reported during recent decades that was attributed to a warming-induced drought ([72], [61]).
Cell wall thickness is determined by availability of non-structural carbon (NSC) deposited in the secondary cell walls, which are composed and synthesized by cellulose, lignin, hemicellulose and protein during the secondary wall thickening ([73], [62]). It is believed that cell wall thickness is strongly affected by environmental conditions during the growing season ([58]). Under favorable climate, trees could reserve more current-year NSCs for cell wall thickening ([26]). The conifers commonly found in dry regions are thought to avoid hydraulic failure through an adaptive strategy, such as reduced conduit size and enhanced cell wall, and thus resist higher negative water pressure ([29]). Cell coarseness (µg µm-1), which is defined as the ratio of cell wall mass to cell unit length, is proposed to be used to measure paper quality and found to be tightly associated with drought resilience in the theoretical models ([43]). Overall, climate warming-induced drought may affect cambial cell division, in turn xylem cell number, size and wall thickness, thereby affecting the whole structure and ultimately wood quality.
Earlywood and climate
Earlywood production has been widely shown to be directly related to climate ([69]). More earlywood cells were observed at the black spruce tree-line in warmer years ([93]). Deslauriers et al. ([27]) found longer durations of earlywood production in balsam fir in the warmer 1999 (9-10 weeks) compared to 1998 and 2000 (6-7 weeks). By comparing xylem formation of black spruce at three sites along a latitudinal gradient in Canada, Huang et al. ([51]) showed that trees produced more earlywood cells at southern sites and in a warmer year than those at northern sites and a cold year, respectively. Average earlywood vessel area of Quercus robur L. trees was used to reconstruct minimum winter temperature during the past two centuries in northern Poland ([74]). This suggests that earlywood production is closely related to the climate in the previous or current year depending on the species.
Latewood and climate
Latewood formation is a result of carbohydrates accumulation produced by photosynthesis, which is very sensitive to climatic factors, such as temperature and water stress ([87]). Studies on wood anatomy have shown that onset and termination of latewood cell production in the boreal forest usually range from the end of June to mid-August, varying by species ([27], [51], [98]). Compared to the warm summer of 2005, Huang et al. ([51]) detected a higher proportion of latewood in black spruce in the cold summer of 2006. Their research also found the cold summer temperatures of 2006 resulted in an earlier start and termination of latewood cell production, and consequently fewer total xylem cells were produced in the colder year. Over the spatial scale, they also observed higher ratios of latewood cells to total cells for northern trees than southern trees in both years, which might be due to the limiting effect of colder summer temperatures in the north ([51]). In a boreal forest of eastern Finland, Miina ([66]) found a negative relationship between the proportion of latewood and June temperature, however, the proportion of latewood in Scots pine increased with the temperatures of spring and late summer. In a dry Mediterranean area in Portugal, Campelo et al. ([17]) found a negative effect of temperature in August on latewood formation of Pinus pinea L., probably due to the reduced photosynthesis induced by high temperature.
Overall, we conclude that the whole xylem formation process, including earlywood and latewood, might be mostly affected by the climate during the current growing season. In particular, temperature may influence the timing of earlywood and latewood formation during the xylem formation process according to the physiological status of the trees.
Wood density
Basic wood density (kg m-3) is the ratio of oven-dry mass to green volume and is determined by cell wall thickness, tracheid size, and the ratio of latewood to earlywood and juvenile wood to mature wood ([90]). Wood density is a quality trait that is easily measured and relevant to many product performances (e.g., timber stiffness, pulp, paper productivity - [97]). Therefore, wood density can provide a simple measure of the total amount of biomass present in a piece of wood ([54]) and is one of the most excellent predictors and determinants of wood quality such as stiffness, strength, and machinability ([54], [97]).
Within a tree, wood density can vary between earlywood and latewood, and between juvenile and mature wood, with earlywood and juvenile wood generally having lower density values ([65]). On a transverse section, juvenile and mature wood densities are usually used to describe wood density variation from the pith to bark. Hardwood species have a much higher wood density (e.g., 590-930 kg m-3 for oak) than softwood species (e.g., 380 kg m-3 for western red cedar - [90]). Most commercial softwoods have oven-dry densities of 400-600 kg m-3 ([50]). Besides, wood density could play a vital role in hydraulic efficiency of plants, provide mechanical support and protect from cavitation during drought ([90]). All wood density features (including early/late and juvenile/mature wood density) are critical and widely used to determine wood mechanical properties such as strength, stiffness, elasticity, rupture and pulp yield ([33]), and thereby to describe wood quality.
Radial growth rate of boreal conifers has usually been shown to be negatively correlated with wood density in many species ([42], [5]), and consequently influences wood quality (e.g., gravity and stiffness). For instance, compared with slow-growing trees, fast-growing ones generally produce a larger volume of wood with lower density ([60]). However, there are also some exceptional cases where a higher wood density occurred with higher growth rate ([68], [79]).
As stated in the previous sections, cell number, size and cell wall thickness together determine wood density ([21]). These anatomical traits are reported to be strongly affected by the current and previous growing season climate, such as for white spruce ([58]). Wood density has been widely shown to be positively associated with drought tolerance and survival and an important trait reflecting hydraulic conductance ([91]). In eastern white pine, Larocque et al. ([56]) found that the genetic influence on wood density and growth rate, i.e., tree-ring width, is low, thus indicating that they are strongly environmentally correlated. In boreal and temperate conifer forests, maximum wood density is usually correlated with summer temperature ([89]). This positive relationship is probably due to a thermal-induced enhancement of tracheid lignification in the growing season ([45]). For instance, Camarero & Gutiérrez ([15]) found a decrease in maximum wood density related with cold weather in the late growing season, probably due to a reduction of lignification and thickening rates of latewood tracheids in cold conditions. In addition, species with high wood density were often found in dry or cold environments ([20], [15]). For example, a wood density study at the tree-line of the boreal forest in Quebec by Wang et al. ([93]) showed fewer xylem cells and higher latewood density of black spruce in cold than in warm years. A geographical analysis of 2456 tree species in Central and South America showed that wood density in trees from wet forests is lower than in trees from dry forests ([20]).
Fiber length
Fiber length is the length of wood cells along the longitudinal axis, which is called tracheid length in softwood ([90]). Fiber length is an important wood quality attribute for timber products since longer fibers result in greater resistance of wooden beams to buckling ([90]) as well as for the pulp and paper industry because of the positive relationship between fiber length and paper strength ([54]). Fiber length has been found to be closely correlated with microfibril angle within a tree, with the smaller angles being related to the longer fiber lengths ([84]). The decrease in microfibril angle from the pith outwards to the bark contributes to the higher strength and stiffness of mature wood compared to juvenile wood ([65]). Studies by Zobel & Buijtenen ([101]) showed that the heritability of fiber length varies across species, ranging from weak to high genetic control. For example, fiber length in black spruce [Picea mariana Mill. (BSP)] and slash pine (Pinus elliottii Engelm) was found to be highly genetically controlled, whereas this was not the case for loblolly pine (Pinus taeda L.).
Fiber length is shown to be largely impacted by changing temperature. It is generally believed that trees form large diameter, thin-walled fibers during the early part of the growing season, and smaller diameter, thick-walled fibers in the latter part ([54]). Kilpeläinen et al. ([55]) found fiber length in Scots pine was increased by high temperature. Watt et al. ([94]) revealed that mean air temperature is the strongest environmental determinant of fiber length in Pinus radiata D. Don. In addition to temperature, drought is also shown to be an important factor in influencing fiber length, although with different effects according to season. For instance, in a drought-treated poplar study, Arend & Fromm ([4]) reported that early-summer drought significantly reduced fiber length, whereas no effect was found in late summer. In conifers, the length of tracheids, i.e., fiber length, is generally shaped by the amount of water available during their formation because of its basic function of transporting water ([92]). Xylem anatomy and fiber length define how much water can be transported by a conduit ([6]). Xylem hydraulic architecture is therefore also a useful indicator for climate reconstructions.
Microfibril angle
Microfibril angle (MFA) refers to the angle of cellulose microfibrils in the secondary cell wall of fibers to the tracheid axis ([97], [7]). MFA can strongly influence the strength and elasticity of wood (e.g., shrinkage behavior), which affects its quality ([50], [31]). MFA varies within the stem from pith to bark ([90], see also [32] for more details about MFA). Both vertical and radial variations of MFA are observed in conifers with smaller angles in mature wood than juvenile wood, leading to higher stiffness and elasticity in mature wood ([31]). There are three layers in the secondary cell wall of xylem cell: an outer S1 with transversely oriented microfibrils, a thick S2 layer with axially oriented microfibrils, and an inner S3 with transversely oriented microfibrils ([50], [31] - Fig. 1). MFA varies among cell wall layers, which consequently show diverging mechanical properties ([90], see also [32] for more details). S1 and S3 are relatively thin and play a key role in strengthening the cell against deformation by water tension force, and contribute to the lateral hardness and crushing strength of timber ([31]). Of the three layers, S2 is the thickest one accounting for 60-90% of the volume of the whole cell wall ([50], [31]). As a consequence, S2 plays a dominant role in cell wall physical and chemical properties and is the most important layer for wood quality.
For softwoods, Wimmer et al. ([97]) and Donaldson ([31]) reported higher MFA in earlywood and juvenile wood, and lower in latewood and mature wood. In contrast, MFA is generally below 20° in hardwoods without clear decreasing trends in juvenile and mature wood ([31]). MFA is higher at the stem base, then declines exponentially acropetally, is constant beyond about 7 m, and then increases near the stem top ([31]). For example, Barnett & Bonham ([7]) reported that MFA in Betula pendula Roth declines from 19° at the pith to 12° at the bark at 1 meter height, with slightly lower MFA towards the top. As a result, cell wall stiffness increases from the pith to bark as the MFA decreases. The increasing MFA results in an increasing non-linear longitudinal shrinkage ([7]).
Varied MFA within and along the stem is primarily controlled by heritability ([31]). However, it is also influenced by environmental factors such as temperature, water availability and wind speed ([96], [34]). Donaldson ([31]) stated that the environmental effect on MFA increased with tree age, and the environmentally-induced variation of MFA is less than that associated with position along the stem radius.
The variation of MFA is subjected to climatic influences with a response similar to that of growth rate and ring width ([34]). Temperature has proved to be a significant influencing factor for MFA. For instance, Drew et al. ([34]) reported that while the minimum MFA correlated with summer temperature, maximum MFA best correlated with spring temperature. In addition, water availability has significant effect on MFA. It was shown that MFA increases with irrigation, release of water stress, and rainfall, and decreases with drought ([96], [34]). Wind speed is another factor that directly influences MFA as a result of flexural stress inducing low MFA ([96]).
Conclusions
Climate warming is becoming more and more apparent on the forest ecosystems of high latitudes. Dramatic impacts on tree growth and the anatomical traits of xylem cells are expected, particularly for wood quality. Because of the intrinsic connections between macroscopic (wood) and microscopic (xylem) characteristics of the secondary growth, the study of wood quality is strictly related to the anatomical traits and growth dynamics of xylem. Under a global warming scenario, a comprehensive and deeper understanding of these traits involved with warming conditions is urgently required for the sectors basing their economy on forest products, specifically forest companies, and the private forest owners. Indeed, properties associated with high wood quality vary for different end uses ([48]).
Under climate warming, trees must adjust the anatomical traits of xylem cells to adapt to the increasing temperatures. In general, warming conditions advance the onset of xylem formation in boreal trees by triggering the activity of cambial cells in spring ([81], [51]). Therefore, this advancement of xylem formation might have a possibility to extend growing season and thus increase wood volume ([10]). However, under warming condition, winter chilling, which is also the key factor for triggering growth resumption, is reduced, leading to a delayed growth reactivation ([25]). Timing and duration of the growing season affects cell size ([14]). In addition, photoperiod also acts on the timing of tracheid production, but this factor is not expected to be impacted by climate warming. As a consequence, wood production and quality which are mainly determined by the timing of the growing season is a complex issue influenced by many factors and needs to be studied further.
The wood formation dynamics and tree-ring reflect the interaction of physiological and environmental factors. Thus, any change in xylem anatomy induced by warming, including number, size and wall thickness of cells, would influence wood quality. For boreal softwoods, the proportion of latewood, which mostly determines wood density, is correlated negatively with warming conditions. However, fiber length increases with temperature. Microfibril angle is primarily controlled by heritability but is also affected by temperature in various ways. Our literature review on wood quality concludes that wood with a large proportion of mature wood, high density, longer fiber length combined with smaller microfibril angle would be optimal for the wood industries ([54]). Wood anatomy depends on a large range of elements that all should be taken into consideration when studying the effect of climate warming.
Perspectives for future research
Forest management practices including silvicultural techniques to manage tree growth and wood production can be used to improve wood quality. For instance, cutting and thinning has often been performed to obtain a good wood quality ([48], [39]), but these techniques should be adapted as environmental conditions change. One of the means to increase productivity is to promote artificial over natural regeneration. Careful selection and provenance of breeding material is primordial to achieve this goal ([85]). In addition, using molecular and genetic techniques to improve wood quality can be used ([78]). For example, marker genomic selection is expected to sufficiently improve wood quality by considerably reducing breeding cycles and increasing selection accuracy ([9]). With the development of molecular and genetic techniques and a deeper understanding of the xylem cell structure along with the processes of xylem formation, trees with high quality wood for future needs could be fostered. These trees could also be expected to cope with climate extremes, such as the severe climate warming. Future research should focus on the species or provenances more adapted to warming and drying conditions, which may allow to improve wood traits without sacrificing volume. Forest managers and ecologists should aim to combine production in volume (i.e., large tree-ring width or high growth rates) and quality. Private forest owners from the southern boundary of the boreal area may focus on particular wood characteristics depending on specific demands and convert their stands towards an intensive forestry based on more lucrative hardwood species.
The anatomical traits can be hardly optimized simultaneously because of the trade-off between growth maximization and environmental adaptation ([71]). For example, trees with a high growth rate often produce low density wood ([42], [5]). Juvenile wood that is produced by a high growth rate in the early years of a tree’s life span usually has a low density, short fiber length and large microfibril angle ([54]). However, poplar clones with higher growth rates also show better water use under drought conditions and quicker recovery ([46]). Therefore, studies to find an optimal balance between these anatomical traits should aim to enhance wood quality.
In a natural environment, extreme conditions usually induce physiological stress in trees. For example, in most cases, warm conditions with high temperatures induce drought stress ([1]). Therefore, wood quality studies should be conducted in an interactive environment to clearly investigate changes in wood quality under realistic climate change. Multi-disciplinary physiological and biological researches, including molecular studies of wood formation processes, are also needed to reveal the underlying mechanisms of wood traits responses to climate change. Lastly, not only global warming will not affect wood formation and wood quality ([53]). Increasing CO2 and N deposition will accompany the rising temperature and changed precipitation patterns, and consequently impact wood formation ([24]). A more comprehensive understanding of the response of wood traits to climate change is therefore necessary. Thus, improving our mechanistic understanding of the responses of wood formation to interacting factors of climate change will be crucial to deal with and predict changes in required quality wood production.
Understanding of mechanisms and dynamics of wood formation in boreal regions progressed quickly during the last 20 years. A lot of literature is available on wood formation, anatomical traits and quality ([80], [22], [82]). However, there is still limited effort being made to explore the mechanisms and quantify the relationships between wood quality and cell anatomical traits.
There is a large quantity of tree-ring data, including ring width, wood density, and isotopic data already archived in the international tree-ring database, as well as wood formation data collected during recent years using the micro-sampling approach ([23], [25]) or confocal laser scanning microscopy ([59], [47], [100]). Using this data, a mechanistic or process-based model should be developed ([18]) to predict wood quality within the context of global change. Therefore, understanding of the underlying mechanisms of anatomical traits that are related with wood quality and climate change is still considered a crucial research topic. This must be developed at a multidisciplinary level, including silviculture, wood sciences, physiological ecology as well as molecular biology and genomics.
Acknowledgments
JGH: conceived the idea. SZ and JGH: reviewed articles and wrote the manuscript. SR and EB: contributed to the writing of the manuscript, the literature review and revision of the final draft. HR: contributed to the revision of the manuscript, and reviewed its final version. All authors have read and approved the final manuscript.
This project was funded by the National Natural Science Foundation of China (Grant No. 41661144007, 41861124001, 31570584, and 31901166), Natural Science Foundation of Guangdong Province (2019B121202007), China Postdoctoral Science Foundation (Grant No. 2018M643227), Key Special Project for Introduced Talents Team of Southern Marine Science and Engineering Guangdong Laboratory (Guangzhou) (GML2019ZD0408), CAS/SAFEA International Partnership Program for Creative Research Teams, the Natural Sciences and Engineering Research Council of Canada and the Fonds de Recherche Nature et Technologies du Québec (FRQNT). The authors thank P. G. Comeau and S. A. Alam for their suggestions on the manuscript and A. Garside for checking the English text.
Conflicts of interest
The authors declare that the research was conducted in the absence of any commercial or financial relationships that could be construed as a potential conflict of interest.
References
CrossRef | Gscholar
CrossRef | Gscholar
Online | Gscholar
CrossRef | Gscholar
CrossRef | Gscholar
CrossRef | Gscholar
Gscholar
Gscholar
CrossRef | Gscholar
CrossRef | Gscholar
CrossRef | Gscholar
Gscholar
Authors’ Info
Authors’ Affiliation
Hai Ren 0000-0002-3744-8007
Sergio Rossi 0000-0002-9919-0494
Jian-Guo Huang
Key Laboratory of Vegetation Restoration and Management of Degraded Ecosystems, South China Botanical Garden, Chinese Academy of Sciences, Guangzhou 510650 (China)
Hai Ren 0000-0002-3744-8007
Sergio Rossi 0000-0002-9919-0494
Jian-Guo Huang
Guangdong Provincial Key Laboratory of Applied Botany, South China Botanical Garden, Chinese Academy of Sciences, Guangzhou 510650 (China)
Sergio Rossi 0000-0002-9919-0494
Département des Sciences Fondamentales, Université du Québec à Chicoutimi, Chicoutimi (QC) G7H2B1 (Canada)
Corresponding author
Paper Info
Citation
Zhang S, Belien E, Ren H, Rossi S, Huang J-G (2020). Wood anatomy of boreal species in a warming world: a review. iForest 13: 130-138. - doi: 10.3832/ifor3230-013
Academic Editor
Rodolfo Picchio
Paper history
Received: Sep 05, 2019
Accepted: Jan 26, 2020
First online: Apr 09, 2020
Publication Date: Apr 30, 2020
Publication Time: 2.47 months
Copyright Information
© SISEF - The Italian Society of Silviculture and Forest Ecology 2020
Open Access
This article is distributed under the terms of the Creative Commons Attribution-Non Commercial 4.0 International (https://creativecommons.org/licenses/by-nc/4.0/), which permits unrestricted use, distribution, and reproduction in any medium, provided you give appropriate credit to the original author(s) and the source, provide a link to the Creative Commons license, and indicate if changes were made.
Web Metrics
Breakdown by View Type
Article Usage
Total Article Views: 40770
(from publication date up to now)
Breakdown by View Type
HTML Page Views: 33155
Abstract Page Views: 4056
PDF Downloads: 3002
Citation/Reference Downloads: 22
XML Downloads: 535
Web Metrics
Days since publication: 1687
Overall contacts: 40770
Avg. contacts per week: 169.17
Article Citations
Article citations are based on data periodically collected from the Clarivate Web of Science web site
(last update: Feb 2023)
Total number of cites (since 2020): 7
Average cites per year: 1.75
Publication Metrics
by Dimensions ©
Articles citing this article
List of the papers citing this article based on CrossRef Cited-by.
Related Contents
iForest Similar Articles
Research Articles
Earlywood vessel features in Quercus faginea: relationship between ring width and wood density at two sites in Portugal
vol. 8, pp. 866-873 (online: 27 April 2015)
Research Articles
Impact of rotation length of Eucalyptus globulus Labill. on wood production, kraft pulping, and forest value
vol. 15, pp. 433-443 (online: 20 October 2022)
Research Articles
Impact of climate change on radial growth of Siberian spruce and Scots pine in North-western Russia
vol. 1, pp. 13-21 (online: 28 February 2008)
Short Communications
Effect of intensive planting density on tree growth, wood density and fiber properties of maple (Acer velutinum Boiss.)
vol. 9, pp. 325-329 (online: 22 October 2015)
Research Articles
Preliminary study on genetic variation of growth traits and wood properties and superior clones selection of Populus ussuriensis Kom.
vol. 12, pp. 459-466 (online: 29 September 2019)
Research Articles
Auxin (IAA) and soluble carbohydrate seasonal dynamics monitored during xylogenesis and phloemogenesis in Scots pine
vol. 11, pp. 553-562 (online: 01 September 2018)
Research Articles
Physical, chemical and mechanical properties of Pinus sylvestris wood at five sites in Portugal
vol. 10, pp. 669-679 (online: 11 July 2017)
Short Communications
Climate effects on growth differ according to height and diameter along the stem in Pinus pinaster Ait.
vol. 11, pp. 237-242 (online: 12 March 2018)
Research Articles
Climate impacts on tree growth in a Neotropical high mountain forest of the Peruvian Andes
vol. 13, pp. 194-201 (online: 19 May 2020)
Review Papers
Impacts of climate change on the establishment, distribution, growth and mortality of Swiss stone pine (Pinus cembra L.)
vol. 3, pp. 82-85 (online: 15 July 2010)
iForest Database Search
Search By Author
Search By Keyword
Google Scholar Search
Citing Articles
Search By Author
Search By Keywords
PubMed Search
Search By Author
Search By Keyword