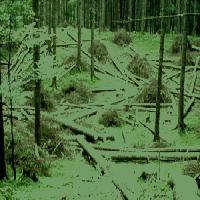
Forests and climate change - lessons from insects
iForest - Biogeosciences and Forestry, Volume 1, Issue 1, Pages 1-5 (2008)
doi: https://doi.org/10.3832/ifor0210-0010001
Published: Feb 28, 2008 - Copyright © 2008 SISEF
Review Papers
Abstract
The climate change may indirectly affects the forest ecosystems through the activity of phytophagous insects. The climate change has been claimed to be responsible of the range expansion northward and upward of several insect species of northern temperate forests, as well as of changes in the seasonal phenology. Several papers have dealt with the prediction of the most likely consequences of the climate change on the phytophagous insects, including some of the most important forest pests. Increased levels of CO2 in the atmosphere involve an increase of the C/N balance of the plant tissues, which in turn results in a lower food quality for many defoliating insects. Some insects respond by increasing the level of leaf consumption and consequently the damage to the tree, whereas others show higher mortality and lower performance. The level of plant chemical defences may also be affected by a change of CO2. The temperature is affecting either the survival of the insects which are active during the cold period, such as the pine processionary moth, or the synchronization mechanism between the host and the herbivores, as in the case of the larch bud moth. An increase of temperature may alter the mechanism by which the insects adjust their cycles to the local climate (diapause), resulting in faster development and higher feeding rate, as in the case of the spruce web-spinning sawfly outbreaks in the Southern Alps.
Keywords
Climate change and insect distribution
The effects of global warming on living organisms have now been recognized from the level of individual species to communities, most notably in the form of temperature-related range shifts ([53], [41], [24]). As the number of insects per unit area is inversely related to latitude and elevation ([49]), we may assume that the increase of temperature would allow the spreading of insect species northward and upward, especially for those species that have wide ranges, as many forest pests have. This assumption is supported by fossil data related to the forest insect response to climatic changes of the past. Higher damage and insect diversity was recorded during the global warming which occurred during the Paleocene - Eocene transition, relative to other periods ([54]).
With mean global temperatures increasing over the past 100 years by about 0.8 °C and projected to continue ([48]), widespread climate-related changes in the biosphere can be expected. There are various ways by which the insects may react to climate change ([55], [1], [22], [2], [37]), and it seems reasonable to assume that an increase of temperature within the vital limits of a species implies a faster development. The species ready to expand are those characterized by high growth potential, multivoltinism and absence of diapause, whereas those that could be restricted show slow development rate and long cycles. The reduction of the period of time spent as a larva or pupa may improve survival, as these are the stages more subjected to predation and other mortality factors ([9]). The increase in population density may in turn promote a further expansion of the range. Some species would be simply limited in their survival at the southern edge of their range and would shift the range northward. Switching to new hosts may occur among non-specialist herbivores, and can be the first consequence of the strong selection on colonizers ([22], [50]).
Parmesan & Yohe ([38]) have provided a quantitative assessment of the biological impact of climatic change, using data from different types of organisms, including insects. This analysis concerned the spatial (range shift) and phenological (advancement of spring events) data, averaging 6.1 km/decade and 2.3 days/decade, respectively. Eighty percent of the studied species (n = 434) showed a consistent range shift and 87% an advancement of spring events, such as flowering or migration.
However, the response of insects to climatic change may not always be linear ([2], [20]). For example, the developmental stages of the insects can be differentially affected by the climate change, i.e., the growth can be accelerated by higher temperature, but at the same time the length of diapause may be extended.
Those insects developing without winter diapause, which are active during this season and are protected from the low temperature, are the best candidates for range expansion if the winter temperature maintain the current increasing trend ([46]). The increase in winter temperature is a key factor for the survival of the lepidopteran Atalopedes campestris in the new colonization areas ([16]). A good example concerning a forest pest is the case of the pine processionary moth Thaumetopoea pityocampa (Box 1, Fig. 1), reported also in the last IPCC report ([42]).
Fig. 1 - Egg batch of the pine processionary moth Thaumetopoea pityocampa on a twig of Pinus sylvestris in the expansion outbreak area of Mont Avic (Aosta), at the unusual elevation for an alpine population of 1450 m. The insect shows high performance and almost no mortality due to the absence of the main natural enemies; a genetic analysis has shown that the population originated from different sources, suggesting that female moths are flying over bigger distances than previously believed ([45]).
However, most forest insects of temperate regions have a winter diapause, which in some cases can last several years. Temperature plays a major role in the induction and maintenance of this diapause. An increase of the temperature would modify the induction and maintenance of the diapause, involving changes, which could affect the development of the insect, making predictions about population dynamics quite unreliable. Two examples are reported here, which illustrate how high temperature during the larval development has caused lower diapause rate and higher damage by the spruce webspinning sawfly Cephalcia arvensis (Box 2), and how high winter temperature has disrupted the maintenance and termination of the egg diapause in the larch bud moth Zeiraphera diniana (Box 3), causing a poor synchronization with the host and the absence of an expected outbreak.
A different situation is presented by species that are already adapted to the cold environments, such as Aglais urticae. These would probably undergo a restriction of the range if they become limited at their southern boundary by increasing temperature ([13]).
For insects that are heavily dependent on a favourable synchronization between bud breaking and hatching, such as the winter moth Operophtera brumata, it appears that there may be a compensation between a faster egg spring development and a delayed pupation in autumn, both triggered by an increase of temperature ([15]). Therefore, phenology is not affected, allowing Bale et al. ([2]) to conclude that the effects of the global warming would not be so evident in some species.
Finally, the natural enemies of forest insects may be affected by the temperature change in different directions or extent. The expansion of the host may not be promptly followed by that of its enemies, as in the case of the pine processionary moth ([57]), or the synchronization between host and parasitoid may not be maintained under new temperature conditions. This seems to be the case of a parasitoid of the winter moth Operophtera brumata, which is effective at low elevation but that is almost absent at high elevation ([32]).
All the examples cited above illustrate how insects may react to the climate change, however they also have a great potential to develop physiological and behavioural adaptations, which may improve their fitness under new conditions. This would ultimately lead to the formation of genetically differentiated populations and possibly new species, especially when the climatic change is associated with range expansion and host switch.
Climate change and host-insect interactions
The capacity of an herbivore insect to complete its development depends on the adaptation to both, the environmental conditions and the host plant. The plant may respond to changes in temperature by varying its growth rate, as it is commonly observed along latitudinal or altitudinal gradients, when the conditions become progressively less favorable and the herbivores are more limited in the host’s exploitation. An example is given by the willow psyllid (Cacopsylla spp.), which is restricted to feed on a low number of species or types of plant tissues as the range edge is approached ([27], [25]).
The change of temperature, which promotes the expansion of the insect’s range, may also involve a new association between a herbivore and its host, as it has been shown by the pine processionary moth attacking the mountain pine (Pinus mugo) in the southern Alps. The large outbreaks observed in the expansion areas on the new hosts may be explained either by the high susceptibility of the hosts or by the inability of natural enemies to locate the moth larvae on an unusual hosts or environment ([26], [50]).
An elevated concentration of CO2 may affect the performance of phytophagous insects through the modification of the nutritional properties of the host plant ([31], [29], [30]). As CO2 is the main carbon source for photosynthesis, its increase could alter the carbon/nutrient balance of plants, increasing the C/N ratio and thus diluting the nitrogen content of the tissues. However, the response of plants to increased CO2 varies among species. A high concentration causes an increase of tannins in the leaves of birch, poplar and maple, but not in the eastern white pine (Pinus strobus - [43]).
The first reaction expected from herbivores to the increase of the C/N ratio is compensatory feeding, in other words they should eat more to accumulate enough nitrogen for their development. Thus, plant damage may increase, but plant biomass could remain stable if we assume that the plants exposed to high CO2 grow more. Phytophagous insects may also develop adaptations to overcome higher C/N ratios, such as the pine sawfly Neodiprion lecontei, which shows an increase in the efficiency of nitrogen utilization when reared on plants treated with high CO2 concentration ([56]). However, other insect species seem unable to compensate the lower nutritional quality of the plants by increasing the efficiency of nutrient utilization ([12], [51]). The experiments of Lindroth et al. ([33]), on three species of saturnid moths, show that the performance of the caterpillars is only marginally affected when the nitrogen content of the leaves is reduced by 23% and the C/N ratio increased by 13-28%.
Experiments combining different concentrations of both nitrogen and carbon dioxide supplied to Norway spruce showed that a high nitrogen level may compensate the effects of CO2 on the concentration of nutrients and defence compounds in the shoots, limiting the negative effects on the test insect Lymantria monacha ([21]).
The effects of a modified atmosphere on herbivore insects could also involve the third trophic level, i.e., their parasitoids and predators. As we are expecting a delay in the developmental time of the herbivores after exposure to high CO2 ([19], [33], [47]), the probability of parasitism and predation should increase as well. Experimental evidence of such a hypothesis is contradictory, as Roth & Lindroth ([44]) did not find higher parasitism by the hymenopteran Cotesia melanoscela on the larvae of Lymantria dispar raised at high CO2, whereas Stiling et al. ([51]) found higher mortality of oak leaf miners by parasitoids on two species of oak (Quercus myrtifolia, Q. geminata) grown at high CO2 level.
Laboratory or greenhouse experiments provide valuable data, but it is difficult to derive conclusions applicable to the natural environment. For example, high CO2 levels are known to increase the temperature and, indirectly may affect the host-herbivore interaction.
Dury et al. ([18]) showed that an increase of 3°C of the temperature might lead to the same effects of an increase of CO2 (decrease of nitrogen, increased of condensed tannins) on oak leaves. However, an increase of temperature may enhance the feeding of the herbivore and thus compensate for the negative effects of a lower food quality. An experiment that tested simultaneously the effects of different levels of CO2, nitrogen and temperature on the monoterpene production of Pseudotsuga menziesii ([34]), indicated that the synthesis of these defence compounds was more affected by individual tree variability than by the treatments.
The response of herbivore insects to increased CO2 may also differ among the feeding guilds, as suggested by Bezemer & Jones ([10]). Defoliators are generally expected to increase leaf consumption by about 30%, but leaf miners showed a much lower rate. Phloem-sucking insects appear to take the greatest advantage from increased CO2, as they grow bigger and in a shorter time. In a FACE (Free Air Carbon Enrichment) experiment carried out in Wisconsin ([39]), the activity of all guilds of herbivores, combined with the effect of increased ozone, may be compensated by the beneficial consequences of enriched CO2 on growth of Populus tremuloides.
More research is clearly needed to make reliable predictions about the effects of climate change on the relationships between the forest trees and phytophagous insects. Good conceptual frameworks, such as the carbon/nutrient balance ([13]) and the growth/differentiation balance ([23]) theories are available to interpret experimental results and to formulate new hypotheses. Hopefully, this understanding of the effects of climate change on forest pests will enable us to take the necessary measures to counteract or mitigate the possible negative consequences on the forest ecosystems.
Box 1 - The pine processionary moth Thaumetopoea pityocampa
The winter pine processionary moth, Thaumetopoea pityocampa offers a possibility to test for the effects of global warming on an insect population over a wide area of the Mediterranean basin and southern parts of Europe, where it is the most important pest of pine forests (Pinus spp.). Its geographic range lies within precise limits of elevation and latitude ([17]), primarily as a function of the average winter temperatures. Because the larvae are oligophagous, potentially feeding on all Pinus spp., but also on Cedrus spp. and the introduced Pseudotsuga menziesii, host plant distribution does not restrict the present range of the insect; many usual or potential host species grow in areas where the insect is absent. Consequently, if the climatic conditions become favourable in higher latitudes or at higher elevations, the insect may expand its range to these areas, often coupled with host switching ([7], [40]). This relative importance of temperature over biotic factors in defining the geographic distribution makes the moth a particularly suitable model to study the range shift in relation to global warming ([14]).
An important forest pest in many areas, the moth has shown in the last decades a substantial expansion of the outbreak area both northward and upward ([28], [26], [7]), aggravated by extreme climatic events such as the summer of 2003 ([8]). This has resulted in high attack rates in areas previously largely unaffected by the insect ([50]). The case deserves special interest for the implications it may have on the management of European forests and plantations, as well as on ornamental trees.
Box 2 - The spruce webspinning sawfly Cephalcia arvensis
The outbreaks of the spruce web-spinning sawfly Cephalcia arvensis in the Southern Alps are a good example of what may happen when favourable climatic conditions interfere with the mechanism of the induction of extended diapause, allowing an exponential growth of the population and consequent damage to trees. This species, as many others in this genus, is monophagous on Picea and endemic to the spruce range in Eurasia, where outbreaks have been seldom recorded ([36]). Cephalcia species generally show low fecundity and spreading of the cohort over many years by mean of an extended diapause, which is induced by low temperature at pupation time ([5]). However, in the period 1985-1992 there was a sudden outbreak in the Southern Alps (Asiago and Cansiglio Plateaux - Fig. 2), during which the populations developed an annual life cycle and grew exponentially, causing repeated defoliations, which ultimately caused tree death over hundreds of hectares ([6]). The most likely reasons for such a change in the life cycle of the insect have been explored through an analysis of the local climate, which showed that the years preceding the outbreak were characterized by an abnormally warm and dry weather during the feeding period of the larvae. We hypothesized that favourable conditions promoted the survival and speeded up the development, making it possible to pupate when soil temperature was high enough to start pupation immediately, skipping in this way from the extended diapause. Later, an experiment showed that the soil threshold temperature for the induction of the extended diapause was about 12°C ([5]), well below the values recorded in the forest at the beginning of the outbreak. The sudden increase of the population density was not quickly followed by that of natural enemies, which were unable to limit population growth ([6]). It seems likely that the increase of the temperature in June and July of 1983-85 is the major factor promoting the outbreaks, as they occurred simultaneously at two sites at a distance of about 100 km, through switching the insects to an annual generation.
Fig. 2 - Salvation logging after repeated defoliation of the spruce web-spinning sawfly Cephalcia arvensis in Norway spruce stands of Cansiglio Plateau (Southern Alps). This was the first outbreak of this insect ever recorded in the Alps, where it is endemic.
Box 3 - The larch bud moth Zeiraphera diniana
The larch bud moth Zeiraphera diniana is likely the best example of a forest insect showing population cycles in Europe, where it has been defoliating large patches of larch stands in the Alps every 8-10 years for centuries ([4]). This insect has an annual life cycle and spends the winter as an egg on the larch branches, starting to feed on the needles as soon as the bud breaks. Multiple factors seem to be involved in triggering the cycles, such as the host plant quality, the natural enemies, and the migration of the moths ([4], [11], [52]). However, a disturbance probably related to climate change has caused the collapse of an outbreak, which was started in 1989 in the traditional occurrence area in Upper Engadine Valley ([3]). In the following years the larval density decreased drastically and Baltensweiler ([3]) was able to demonstrate that it was due to unusually high egg mortality, caused by repeated unfavourable weather conditions in winter and spring 1989-91. In fact, it appears that weather is a critical factor for a good synchronization between bud burst and hatching of the larvae. Since these conditions occurred in three successive generations, population growth was effectively reduced and the cycle collapsed prematurely. In a recent analysis of European temperature trend since 1500 ([35]), it appears that “the winter 1989/1990 (ΔT=+2.4°C) and the decade 1989 to 1998 (ΔT=+1.2°C) were the warmest since 1500. The period 1989 to 1998 was almost two standard errors warmer than the second warmest (non-overlapping) decade (1733 to 1742, ΔT=+0.45°C), thus was very likely (95% confidence level) warmer than any other decade since 1500”. The abnormally high temperature recorded in that period is possibly the reason for the disruption of the coincidence between the larch and the bud moth, probably through the induction of high egg mortality (Fig. 3).
Fig. 3 - Larch stand at timberline in the Eastern Dolomites, where the cyclic outbreaks of the larch bud moth Zeiraphera diniana are less regular and of variable intensity, fitting the model of the easterly directional population waves created by Bjørnstad et al. ([11]), based on the 35-year dataset of Baltensweiler & Rubli ([4]).
Acknowledgements
This work has been supported by the EU 5th FP QLK5-CT-2002-00852 project “Promoth” (Global change and pine processionary moth: a new challenge for integrated pest management) and by the project “Forest Monitoring” of Regione del Veneto. I thank Michael Stastny and Edoardo Petrucco Toffolo for the useful discussions and for the help in screening the literature.
References
CrossRef | Gscholar
Gscholar
Gscholar
Gscholar
Gscholar
Gscholar
Gscholar
CrossRef | Gscholar
Gscholar
Gscholar
Authors’ Info
Authors’ Affiliation
Dipartimento Agronomia Ambientale e Produzioni Vegetali, Università di Padova, Via Università 16 - Agripolis, I-35020 Legnaro, Padova, Italy
Corresponding author
Paper Info
Citation
Battisti A (2008). Forests and climate change - lessons from insects. iForest 1: 1-5. - doi: 10.3832/ifor0210-0010001
Paper history
Received: Oct 18, 2007
Accepted: Oct 24, 2007
First online: Feb 28, 2008
Publication Date: Feb 28, 2008
Publication Time: 4.23 months
Copyright Information
© SISEF - The Italian Society of Silviculture and Forest Ecology 2008
Open Access
This article is distributed under the terms of the Creative Commons Attribution-Non Commercial 4.0 International (https://creativecommons.org/licenses/by-nc/4.0/), which permits unrestricted use, distribution, and reproduction in any medium, provided you give appropriate credit to the original author(s) and the source, provide a link to the Creative Commons license, and indicate if changes were made.
Web Metrics
Breakdown by View Type
Article Usage
Total Article Views: 66088
(from publication date up to now)
Breakdown by View Type
HTML Page Views: 54667
Abstract Page Views: 3348
PDF Downloads: 6907
Citation/Reference Downloads: 126
XML Downloads: 1040
Web Metrics
Days since publication: 5994
Overall contacts: 66088
Avg. contacts per week: 77.18
Article Citations
Article citations are based on data periodically collected from the Clarivate Web of Science web site
(last update: Feb 2023)
Total number of cites (since 2008): 38
Average cites per year: 2.38
Publication Metrics
by Dimensions ©
Articles citing this article
List of the papers citing this article based on CrossRef Cited-by.
Related Contents
iForest Similar Articles
Research Articles
Performances of an expanding insect under elevated CO2 and snow cover in the Alps
vol. 1, pp. 126-131 (online: 27 August 2008)
Research Articles
Estimating the potential threat of increasing temperature to the forests of Turkey: a focus on two invasive alien insect pests
vol. 15, pp. 444-450 (online: 03 November 2022)
Research Articles
Predicting phenology of European beech in forest habitats
vol. 11, pp. 41-47 (online: 09 January 2018)
Research Articles
Potential impacts of regional climate change on site productivity of Larix olgensis plantations in northeast China
vol. 8, pp. 642-651 (online: 02 March 2015)
Research Articles
Links between phenology and ecophysiology in a European beech forest
vol. 8, pp. 438-447 (online: 15 December 2014)
Research Articles
Impact of climate change on radial growth of Siberian spruce and Scots pine in North-western Russia
vol. 1, pp. 13-21 (online: 28 February 2008)
Research Articles
Multiscale characteristics of the early spring temperature and response to climate indices over the past 179 years in the Qinling Mountains
vol. 15, pp. 491-499 (online: 01 December 2022)
Research Articles
Influence of climate on tree health evaluated by defoliation in the ICP level I network (Romania)
vol. 10, pp. 554-560 (online: 05 May 2017)
Research Articles
Effects of artificial defoliation and simulated insect damage on the growth of Betula pendula saplings
vol. 9, pp. 95-100 (online: 15 July 2015)
Research Articles
Species-specific climate response of oaks (Quercus spp.) under identical environmental conditions
vol. 7, pp. 61-69 (online: 18 November 2013)
iForest Database Search
Search By Author
Search By Keyword
Google Scholar Search
Citing Articles
Search By Author
Search By Keywords