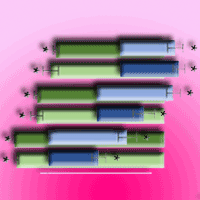
Chitosan exhibits variable effects on pine (Pinus sylvestris L.) and alder (Alnus glutinosa L.) growth and secondary metabolism
iForest - Biogeosciences and Forestry, Volume 17, Issue 4, Pages 236-244 (2024)
doi: https://doi.org/10.3832/ifor4404-017
Published: Aug 06, 2024 - Copyright © 2024 SISEF
Research Articles
Abstract
Chitosan is a naturally abundant biopolymer that has been studied as an alternative means to elicit beneficial responses in plants, such as enhanced plant growth and improved defense response. Most studies focus on agriculture and horticulture, but few have investigated forest trees. Based on chitosan’s success as a biostimulant, we hypothesized that trees such as pine (Pinus sylvestris L.) and alder (Alnus glutinosa L.) may also be positively affected by it. We evaluated vegetative growth parameters and secondary metabolites (phenolics, antioxidant indicators), pigments of seedlings grown in sterile in vitro and non-sterile ex vitro conditions to determine if various concentrations of chitosan solubilized in different solvents and stored in different conditions would significantly impact trees at various stages and conditions of growth. Results showed that in in vitro conditions, chitosan had a mainly positive effect on pine germination and growth, but a mainly negative effect on pine secondary metabolism (SM) indicators. In vitro grown alder’s SMs were positively affected in all measured parameters, but chitosan had no effect on growth. Overall, ex vitro results indicated that chitosan has no effect on pine growth and varied impact on its SM indicators. Similarly, the effect on growth parameters and SM indicators was varied in alder. Results on how chitosan storage can affect its impact on tree growth showed that storage conditions had a significant impact on both pine and alder. Based on our data, future studies must carefully evaluate all possible variables to optimize chitosan use in silviculture.
Keywords
Forest, Tree, Chitosan, Growth Improvement, Secondary Metabolites
Introduction
Chitosan is a biopolymer derived from chitin, a naturally occurring substance found in the exoskeletons of crustaceans, insects and fungi cell walls ([14], [17]). It has been studied for its potential use in agriculture and horticulture due to its various beneficial properties, such as its ability to enhance plant growth and improve plant defence mechanisms, helping combat pests and diseases ([22], [11], [20], [28], [14], [16]).
As chitosan is a natural and biodegradable substance, it can be used as an alternative to synthetic pesticides and fertilizers. Thus, its use can reduce the environmental impact of agricultural practices ([11], [14], [16]). Chitosan shows promise in this area as a sustainable means for enhancing plant growth and improving plant health in agriculture and horticulture ([11], [20]).
In the past chitosan has been shown to improve plant growth by stimulating root development and increasing nutrient uptake ([35], [39], [36]). This can lead to higher yields and better-quality crops. A study conducted on strawberry plants lead to the conclusion that chitosan application improved plant height, leaf number, fresh and dry weight; these parameters in groups treated with chitosan were significantly higher than the control treatment. Fruit quality also showed similar trends ([1]). Chitosan has also been found to enhance the defense mechanisms of plants helping fight back various pests and diseases, as well as improve fruit yield ([28]). Kuyyogsuy et al. ([19]) reported that chitosan enhanced rubber trees’ resistance to Phytophthora palmivora fungal pathogen by reducing disease severity. In addition, antioxidant enzyme (catalase, peroxidase, etc.) production was enhanced ([19]). Overall, chitosan use shows promise, however, there are fewer studies in regard to forestry and its use in forest tree growth improvement and protection ([35], [31]). For example, a study found that chitosan induces a biochemical response in pine when it is used as an elicitor, and that this response lowers the pinewood nematode population by sixfold after just 28 days. Authors linked this outcome with enhanced production of antioxidative chemicals, such as catalase, carotenoids, phenols, etc. ([23]). The activation of plants’ secondary metabolism and the production of phenols, flavonoids, carotenoids and other antioxidative biomolecules have long been linked with the enhancement of plants’ defensive systems ([41], [30]).
It is important to note that there are different types of chitosan that vary in their properties, including, most notably, degree of deacetylation and molecular weight. These intrinsic properties have been shown to affect on how chitosan interacts with plants ([22], [17], [37]). The degree of deacetylation refers to the extent to which chitin has been deacetylated to produce chitosan ([17]). The higher the degree of deacetylation, the more positively charged amino groups are present on the chitosan molecule. This can affect its solubility, antimicrobial activity, and ability to interact with other molecules. The molecular weight of chitosan refers to the size of the chitosan molecule, which can vary depending on the source material and production method. Chitosan of a certain molecular weight may be more effective in some applications, such as inducing plant defense mechanisms ([22]) or improving plant growth ([21]). Overall, since the properties of chitosan can impact its effectiveness in different applications, it is important to consider the specific application and desired outcomes when selecting a specific type of chitosan to use.
Based on the above evidence we chose chitosan derived from Tenebrio molitor L. mealworm for our studies. Beetle chitosan is sourced more sustainably than that of crustaceans and has fewer impurities. It has lower molecular weight and higher degree of deacetylation, which suggests that it may dissolve better in water, which is desirable in plant application ([17]). Considering the scarcity of studies using chitosan on forest trees, we hypothesized that chitosan derived from T. molitor solubilized in different solvents, and stored in different conditions may affect tree seedlings differently in terms of secondary metabolism and more importantly, as there are few if any studies about this, their vegetative growth parameters.
Materials and methods
Seed preparation
Pinus sylvestris L. and Alnus glutinosa L. seeds were used for this experiment. Both were gathered in a dedicated seed plantation in Lithuania. For in vitro experiments seed surface sterilization was done in a laminar flow hood under aseptic conditions.
Pine seeds were placed in a 20 ml sized syringe with a few drops of Tween 80 (polyoxyethylene sorbitan monooleate) detergent. The syringe was filled with 30% H2O2 to cover the seeds entirely. The seeds were incubated in the mixture for 3 minutes lightly shaking the syringe a few times. The mixture, except seeds, was expelled from the syringe and the syringe was filled with sterilized dH2O. The syringe was then shaken a few times and the water then expelled. The rinsing with water was repeated three more times. Afterward the seeds were placed in a sterile container.
Similarly, alder seeds were placed in a 20 ml syringe, however a few pine seeds were added first to fill the gap of the syringe, since alder seeds are smaller than the syringe opening. Ethanol (96%) was used to cover the seeds in the syringe and after 20 s, the ethanol was expelled. The seeds were then rinsed three times with dH2O as described previously. Then a few drops of Tween 80 were added and 30% H2O2 was used to cover the seeds completely. The mixture was incubated for 80 minutes, gently shaking the syringe every so often. Afterward, the seeds were rinsed with water five times as previously described. Then Tween 80 and 30% H2O2 were added again and again incubated for 80 minutes. Then the rinsing steps were repeated and after that the seeds were stored in a sterile container.
Preparation of chitosan
T. molitor larvae where defatted using hot oil screw press machine. Defatted larvae were boiled twice for 1 h in 1 M NaOH solution to remove proteins and remaining fat, then neutralized in 1 M HCl. Resulting chitin was washed twice with boiling water. Then chitin dried at 75 °C and was later deacetylated in 40% NaOH solution at 110 °C for 90 minutes and the reaction was quenched with ice. Solids were separated from the liquid using the oil screw press machine and washed in boiling water until pH 8.0 was reached. To increase bulk density of the end product it was dissolved in 1% acetic acid, evaporated to dryness at 75 °C and ground in a rotary grinder. This treatment increased bulk density of the product from 0.12 to 0.69 g cm-3. Deacetylation degree (DDA) of the chitosan was determined by transmission infrared spectroscopy using PerkinElmer Spectrum Two® FT-IR spectrometer and PerkinElmer Spectrum IR® software in KBr pellets as follows ([8] - eqn. 1):
where DDA% is a deacetylation degree of chitosan, and A1652 and A3450 are absorbances at specified wavenumber.
Preparation of chitosan solutions
During the experiment two concentrations of chitosan were used: 2 g l-1 and 10 g l-1. For the first and second experiments three solvents were utilized: distilled water, 1% acetic acid (VWR, USA) and neutralized 1% acetic acid ([26]). NaOH (1 M) was used for neutralization (Sigma Aldrich, USA). NaOH solution was slowly pipeted into the acetic acid solution until it reached the desired pH. Chitosan powder was mixed with the different solvents (Tab. 1). After shaking the bottle, the solution was put into a thermal shaker (Adolf Kühner AG, Switzerland) for 3-4 h, at 1 g and 35 °C.
Tab. 1 - Solutions used for the experiments one (in vitro) and two (ex vitro).
No. | Chitosan concentration (g l-1) |
Solvent used | pH |
---|---|---|---|
1 | 0 | dH2O - Control group | 5.85 |
2 | 2 | dH2O | 5.85 |
3 | 10 | dH2O | 5.85 |
4 | 0 | Acetic a. (C2H4O2), 1 % | 2.85 |
5 | 2 | Acetic a. (C2H4O2), 1 % | 2.85 |
6 | 10 | Acetic a. (C2H4O2), 1 % | 2.85 |
7 | 0 | Acetic a. (C2H4O2), 1 % + NaOH, 1 M (20:3 v/v) | 5.85 |
8 | 2 | Acetic a. (C2H4O2), 1 % + NaOH, 1 M (20:3 v/v) | 5.85 |
9 | 10 | Acetic a. (C2H4O2), 1 % + NaOH, 1 M (20:3 v/v) | 5.85 |
As for the third experiment, 3 different storing conditions for chitosan powder were used: (i) 23 °C and 35% humidity (room) for 4 months; (ii) 4 °C and 65% humidity (fridge) for 4 months; (iii) freshly prepared powder. Distilled water and 0.1% acetic acid (pH 3.85) were used as solvents. The same chitosan concentrations of 2 g l-1 and 10 g l-1 were tested.
Seedling growth conditions
Each group in the experiment consisted of 30 individual tree seedlings (3 independent experiments, 10 replicates per experiment). All in vitro trials were conducted on standard composition McCown Woody plant medium (Duchefa Biochemie, Netherlands) with 4 g l-1 of gelrite (Duchefa Biochemie) and 20 g l-1 of sucrose (Duchefa Biochemie) added. The pH was a consistent 5.6 ± 0.1. Temperature in the growth chamber was kept at 25/20 °C under a 16/8 h photoperiod (tube fluorescent lights - Osram, Germany, white light, irradiance 30 μmol m-2 s-2).
Sterile seedlings from in vitro conditions were adapted to non-sterile ex vitro conditions where trials were conducted in seedling trays with individuals seed chambers. Peat-based seed pellets (Jiffy, Estonia) were used for ex vitro trials. Otherwise, the ex vitro trials were conducted under the same conditions.
Experimental treatments
The seed treatments for the first experiment (in vitro) took place under sterile conditions in a laminar flow hood. Freshly prepared chitosan solutions were poured into Petri dishes and kept under UV light for 20 mins (Petri dishes uncovered). Then sterilized tree seeds were put into their respective solutions and soaked for 1 h, and then transferred into 20 mm diameter test tubes (1 seed per tube) and grown for 1 month.
As for the second experiment (ex vitro), untreated tree seeds were put into 20 mm diameter test tubes and grown for 1 month in vitro. Then Scots pine seedlings were extracted from the tubes and put into a jar filled with sterile distilled water and stratified in the fridge overnight. Black alder seedlings were extracted and only 20 mm of shoots without leaves were left (roots were cut off) that were transferred into jars with sterile distilled water and placed into the fridge overnight. The next day pine seedling roots and whole alder shoots were soaked in their respective freshly prepared chitosan solutions for 20 mins, then planted into peat pellets and grown for 6 weeks.
The third experiment (different chitosan storing conditions) was similar to the second one. First, untreated Scots pine seeds were planted in 20 mm diameter test tubes. After a month seedlings were extracted from the tubes and put into a jar filled with sterile dH2O and put into fridge overnight. Afterwards, seedlings were soaked in varied chitosan solutions and transferred into peat pellets to grow for one month.
Growth evaluation
After the studied period, the vegetative growth parameters for each individual seedling were evaluated. Root length and fresh weight, lateral root number, shoot length and fresh weight, largest leaf width for alder seedlings and largest needle length for pines were measured.
Secondary metabolite tests
Extract preparation
Out of each replicate (10 individuals) 1 sample (either leaves or needles) of 0.5 g was collected and processed as previously described ([34]). Three samples per pool of 30 plants were taken. Briefly, the samples were homogenized, and 75% methanol was used for extraction (thermal shaker, 24 h). Filtered samples were used for analyses of antioxidant activity, pigments and phenolics. Three technical replicates per each sample were measured for all SMs.
Total phenol content
Total phenol content (TPC) was determined by using a modified method ([33], [38]). Briefly, 100 µl of the methanol extract was mixed with 2.5 ml of dH2O and a 100 ml of Folin-Ciocalteu reagent and thereafter incubated for 6 minutes. Then 5 ml of Na2CO3 was added and incubated for 30 minutes in the dark. After this, the sample absorbance was measured and TPC was extrapolated based on previously published formulas ([38]).
Total flavonoid content
Total flavonoid content (TFC) was determined by using a method previously described by Striganavičiute et al. ([38]). Briefly, 1 ml of the methanol extract was mixed with 0.3 ml 5% NaNO2 and after a 5 min incubation 0.5 ml of 2% AlCl3 was added. The mixture was incubated for additional 6 minutes and then 0.5 ml 1 M NaOH was added. Afterwards, the sample absorbance was measured and TFC was extrapolated based on previously published formulas ([38]).
Antioxidant activity assays
Total free radical scavenging activity was estimated by using 2.2-Diphenyl-1-picryl-hydrazyl-hydrate (DPPH) following the methodology described by Sirgedaite-Šeziene et al. ([34]). Briefly, the reaction mixture containing 100 µl of the ethanol extract, an additional 400 µl of 75% methanol and 1 ml of 0.1 mM DPPH solution (in 100% methanol) was mixed and measured at 515 nm. Radical scavenging activity was calculated based on Trolox equivalent as thoroughly described by Sirgedaite-Šeziene et al. ([34]).
Similarly, free radical scavenging activity by using 2.2’-Azino-bis(3-ethylbenzothiazoline-6-sulfonic acid (ABTS) was estimated following the methodology previously described ([34]). Briefly, ABTS radical cation was prepared from ABTS stock solution (56 mg of ABTS in 50 ml of dH2O) and 200 µl of 70 nM K2S2O8 16 hours in advance. After 16 h the solution was diluted with dH2O until it reached 0.7 ± 0.2 absorbance at 734 nm. Then 50 µl of the methanol extract was mixed with 2 ml of the ABTS solution and incubated in the dark for 10 mins. Absorbance was measured and based on previously published formulas and models, radical scavenging activity was calculated ([34]).
Photosynthetic pigment content
The amount of chlorophyll a, b and carotenoids was determined based on a modified methodology first presented by Wettstein ([40], [38]). Briefly, samples were collected and homogenized with CaCO3 in 100% acetone. Filtered samples were immediately used for pigment analysis. The formulas and models used were thoroughly described previously ([38]).
Statistical analysis
Data from 3 independent experiments (i.e., 30 replicates) were pooled to make one group for the analysis. Means and standard errors were calculated using the software Microsoft Excel®. Data was further processed using SPSS® (IBM, version 28.0.1.1). Kruskal-Wallis H test for analysis of ranks was chosen as a non-parametric alternative to one-way ANOVA. Post-hoc Dunn’s test (α = 0.05) was conducted to determine pairwise differences ([18], [4]).
Results
In vitro experiments
Chitosan treatment impact on Scots pine vegetative growth parameters, germination percentage and radical scavenging activity are provided in Fig. S1 (Supplementary material). Results showed that acetic acid (1%) and neutralized acetic acid alone and with 2 g l-1 of dissolved chitosan increased pine germination rate by ~30% (Fig. S1a). Root parameters (Fig. S1b) were not significantly influenced by chitosan solutions compared to the control group. Shoot length was positively affected in pines treated with 2 g l-1 of chitosan dissolved in neutralized acid by ~10% (Fig. S1c). While needle length was positively affected by 10 g l-1 of chitosan in both 1% acetic acid and neutralized acid by up to ~10% (Fig. S1d). DPPH radical scavenging activity of extracts significantly increased in pines treated with 10 g l-1 of chitosan dissolved in dH2O and neutralized acid (by up to ~115%), while ABTS radical scavenging activity was not significantly affected by any treatments (Fig. S1e). As shown in Fig. 1, chitosan treatment had no positive effect on Scots pine seeds under in vitro conditions in terms of SMs and photosynthetic pigments. While slightly elevated SM levels were seen in groups with 1 % acetic acid and 2 g l-1 of chitosan solution, neutralized acetic acid and 10 g l-1 of chitosan, they were statistically non-significant. As for chlorophylls, results followed the same trend - chitosan treatment had no positive effect -, on the contrary it was negative.
Fig. 1 - Differences in the amount of total phenolic content (TPC) and total flavonoid content (TFC) (a), chlorophylls a (b), b (c) and carotenoids (d) of in vitro grown Pinus sylvestris seedlings from control group (seeds treated with water), % ± RSE (n = 30). Three technical replicates for each sample were done. Significance of differences was tested by Kruskal-Wallis H test for ranks and post-hoc Dunn’s test for pairs (*: p < 0.05).
As for total phenolic and total flavonoid content (Fig. 2a), chitosan powder dissolved in distilled water, gave the best results - TPC significantly increased by 60% and TFC increased by 75% when chitosan concentration was 2 g l-1. Overall, the optimal concentration for all 3 solvents (distilled water, 1% acetic acid and neutralized acetic acid) was 2 g l-1. It should be noted that 1% acetic acid solvent alone (without chitosan) also had a positive outcome - TPC increased by 42% and TFC by 31 %, compared to the control group. Antioxidant activity increased in all experimental groups with acetic acid as a solvent - DPPH radical scavenging activity of extracts increased by at least 80% in all groups and ABTS - by 70% (Fig. 2b). Once again, 2 g l-1 of chitosan dissolved in water gave the best results - DPPH radical scavenging activity increased by almost 250%, compared to the control group. Not many significant differences were seen in groups with neutralized acetic acid (except for DPPH radical scavenging activity with 2 g l-1 of chitosan, where values increased by ~85%). Concentration of chlorophyll a increased in three tested groups: 2 g l-1 of chitosan in water, acetic acid and neutralized acid (Fig. 2c). The amount of chlorophyll b significantly increased in all tested groups treated with chitosan by at least 25%, regardless of solvent used (Fig. 2d). Solvents alone like acetic acid and neutralized acetic acid increased concentration of carotenoids significantly as well (Fig. 2e). In vitro black alder growth was not notably affected by chitosan. Neither germination rate (Fig. S2a in Supplementary material), root, shoot nor leaf width were affected significantly (Fig. S2b, Fig. S2c, Fig. S2d).
Fig. 2 - Differences in the amount of total phenolic content (TPC) and total flavonoid content (TFC) (a), DPPH and ABTS radical scavenging activity of extracts (b), chlorophylls a (c), b (d) and carotenoids (e) of in vitro grown Alnus glutinosa from the control group, % ± RSE (n = 30). Three technical replicates for each sample were done. Significance of differences was tested by Kruskal-Wallis H test for ranks and post hoc Dunn’s test for pairs (*: p < 0.05).
Ex vitro experiments
ABTS radical scavenging activity increased significantly in 5 studied groups by at least 55%: 10 g l-1 of chitosan, dissolved in all three solvents, as well as in 2 g l-1 of chitosan, dissolved in acetic acid and neutralized acetic acid (Fig. 3). This may indicate that chitosan, regardless of solvent used, can increase radical scavenging activity (ABTS) in Scots pine seedlings after direct treatment to their roots. Meanwhile, in almost all groups the DPPH radical scavenging activity decreased. Significantly so in dH2O and 1% acetic acid with 10 g l-1 of chitosan, and with neutralized acetic acid alone. Fig. S3a (Supplementary material) shows that TPC significantly increased in three groups up to ~30%: 2 g l-1 of chitosan in 1% acetic acid, 2 g l-1 and 10 g l-1 of chitosan in neutralized acid, but TFC levels have significantly dropped in treatments with neutralized acid alone and chitosan dissolved in water (2 g l-1, ~30%). As for photosynthetic pigments (Fig. S3b, Fig. S3c, Fig. S3d in Supplementary material), no positive impact was observed, but chlorophyll b levels were negatively affected in two groups: 10 g l-1 of chitosan in water (~15%) and 2 g l-1 of chitosan in neutralized acid (~10% - Fig. S3c in Supplementary material). Similarly, in the group treated with 2 g l-1 of chitosan in neutralized acid, carotenoid content was also significantly negatively impacted (~10% - Fig. S3d).
Fig. 3 - Differences in the amount of DPPH and ABTS radical scavenging activity of extracts of ex vitro adapted Pinus sylvestris seedlings from the control group, % ± RSE (n = 30). Three technical replicates for each sample were done. Significance of differences was tested by Kruskal-Wallis H test for ranks and post-hoc Dunn’s test for pairs (*: p < 0.05).
Both shoot and root length were measured, as well as leaf width, and the total leaf number was counted. Results indicate that two solutions gave positive outcome for shoot length and leaf width - 10 g l-1 of chitosan, dissolved in water and in 1% acetic acid (Fig. 4a, Fig. 4c). However, only leaf width was significantly affected. Root length was affected negatively in three groups: 2 g l-1 of chitosan in water and neutralized acid, and 10 g l-1 of chitosan in neutralized acid (Fig. 4b). Total leaf count was negatively affected by 1% acetic acid as solvent with 2 g l-1 of chitosan; this negative impact decreased when 10 g l-1 of chitosan was present, likely showing that chitosan can mitigate negative impacts from other variables (Fig. 4d). TPC, TFC together with ABTS radical scavenging activity were negatively affected by both concentrations of chitosan, dissolved in dH2O (Fig. 4e, Fig. 4f). As for TPC and DPPH radical scavenging activity, their concentrations were almost alike, both improved by 1% acetic acid alone and with 2 g l-1 of chitosan. The best results were seen in a group with neutralized acetic acid and 10 g l-1 of chitosan (TPC increased by 80%, DPPH radical scavenging activity by ~130% - Fig. 4e, Fig. 4f). It is noteworthy that ABTS radical scavenging activity significantly increased in all groups (with and without chitosan) with 1% acetic acid. Chlorophyll a, b and carotenoid concentrations increased in trees treated with 2 g l-1 of chitosan dissolved in distilled water (Fig. S4 in Supplementary material). These 3 parameters significantly decreased by at least 20% both in 1% acetic acid alone and in trees treated with 10 g l-1 of chitosan mixed with neutralized acid.
Fig. 4 - Differences in shoot (a) and root (b) length, leaf width (c) and total leaf count (d), in the amount of total phenolic content (TPC) and total flavonoid content (TFC) (e), DPPH and ABTS radical scavenging activity of extracts (f) of ex vitro adapted Alnus glutinosa from the control group, % ± RSE (n = 30). Three technical replicates for each sample were done. Significance of differences was tested by Kruskal-Wallis H test for ranks and post-hoc Dunn’s test for pairs (*: p < 0.05).
Different chitosan storage conditions
Concentration of synthesized chlorophyll a increased significantly in two studied groups: 0.1% acetic acid with 2 g l-1 of chitosan, kept in a fridge, and dH2O with 10 g l-1 of freshly made chitosan (Fig. 5a). Chlorophyll b concentrations significantly increased in the same two groups, as well as in the group with dH2O and 10 g l-1 of chitosan stored in the fridge (Fig. 5b). Overall, chlorophyll a and carotenoids were affected more negatively than positively regardless of chitosan and solvents used (Fig. 5a, Fig. 5c). As for SMs (Fig. S5 in Supplementary material), both TPC and TFC increased by 40% or more in pines treated with 10 g l-1 of fresh chitosan powder dissolved in 0.1% acetic acid. TPC was also significantly positively affected in the other five studied groups up to ~60%: 2 g l-1 of chitosan, kept in the fridge and in the room, dissolved in 0.1% acetic acid, 10 g l-1 of chitosan in water and at both studied concentrations of fresh chitosan with water as a solvent.
Fig. 5 - Differences in the amount of chlorophylls a (a), b (b) and carotenoids (c) of Pinus sylvestris seedlings from the control group, % ± RSE (n = 30), depending on chitosan powder storage conditions and solvent used. Three technical replicates for each sample were done. Significance of differences was tested by Kruskal-Wallis H test for ranks and post-hoc Dunn’s test for pairs (*: p < 0.05).
The results show that for TPC and TFC the use of solvent had a huge impact: 0.1% acetic acid impacted SMs negatively, and dH2O had a positive or neutral impact, compared to the control group (Fig. 6a). This indicates that black alder (broadleaved tree) is more sensitive to acid used as a solvent than the Scots pine (conifer). As for photosynthetic pigments, results varied. Chlorophylls a and b were affected more negatively by dH2O with chitosan, stored both in a fridge and at room temperature, while freshly prepared chitosan had less of a negative impact on these parameters (Fig. 6b, Fig. 6c). Carotenoid concentration was negatively affected in groups with acetic acid as a solvent, and positively in groups with water as a solvent (Fig. 6d). Overall, chitosan storage conditions while showing some significant variations were not the main variable that determined chitosan effectiveness in improving the different parameters; instead, more attention must be paid to the solvent and chitosan concentration used.
Fig. 6 - Differences in the amount of total phenolic content (TPC) and total flavonoid content (TFC) (a), chlorophylls a (b), b (c) and carotenoids (d) of Alnus glutinosa seedlings from the control group, % ± RSE (n = 30), depending on chitosan powder storage conditions and solvent used. Three technical replicates for each sample were done. Significance of differences was tested by Kruskal-Wallis H test for ranks and post-hoc Dunn’s test for pairs (*: p < 0.05).
Discussion
A number of studies published in the last few decades showed the beneficial effect chitosan can have on plants ([1], [6], [43], [7], [24], [27]). Chitosan has been shown to affect growth in wheat in terms of increase in shoot and root length, and similar to our results with pine, chitosan-primed wheat seeds also exhibited better germination rate ([10]). Comparatively, more studies promote chitosan as an effective agent that could be used for induced resistance in plants. The researchers found that chitosan treatments increased the total phenol content and total flavonoid content in mint (Mentha piperita L. - [29]) and other secondary metabolites in Mexican mint ([37]). The previously mentioned study on wheat resulted in chitosan negatively affecting total phenol content, however vastly increasing the amounts of certain antioxidative enzymes, such as catalase, peroxidase and superoxide dismutase, among others ([10]).
Most plant-chitosan studies employ agriculturally important or fast-growing herbaceous species. However, the few studies with woody plants also reported similar beneficial trends due to chitosan application. For example, researchers applied chitosan to the Washington navel orange trees and found that it had an insignificant effect on growth parameters, but improved pigment, sugar content, phenolics, hormones, and varied micronutrients in the plants, overall positively affecting fruit quality ([15]). Our results also showed that chitosan had a much more prevalent impact on secondary metabolism than on growth. Another study investigated how chitosan can impact drought-affected Buddhist pine (Podocarpus macrophyllus [Thunb] Sweet), finding that chitosan application enhanced starch allocation to roots, where simultaneously, antioxidant activity was up-regulated ([13]). A recent study on Rosa roxburghii Tratt. revealed that chitosan-treated plants had up to ~92% efficacy in controlling powdery mildew - a pathogen causing yield losses up to 40%. Total phenol content, total flavonoid content and chlorophyll were also positively affected by chitosan application, as were the amounts of superoxide dismutase and polyphenol oxidase ([44]). The results of our study showed that alder grown in vitro hugely benefited from chitosan application. All measured secondary metabolites, TPC, TFC, chlorophyll and antioxidative activity indicators were positively affected, as compared to the study on Rosa roxburghii. For example, during our trials a ~250% increase in radical scavenging activity (DPPH radical scavenging activity assay) was observed. Comparatively, ex vitro results were far more ambiguous. Radical scavenging increased up to ~140% as measured by both DPPH and ABTS assays, and so did TPC, but different concentrations and the solvents used had a variable effect on pigments and TFC.
Pines (genus Pinus) are the most often investigated trees in relation to chitosan among woody plants. Several studies demonstrated the positive effects chitosan application can have on pine tree resistance to various pathogens and growth. Application of chitosan on Pinus radiata D. Don and Pinus patula Schiede trees induced resistance to Fusarium circinatum fungus. For P. radiata this resistance was dependent on the inoculum concentration, and in certain conditions chitosan application was shown to reduce disease incidence on seedlings by 60% ([25], [9]). Pinus sylvestris was also shown to benefit from chitosan under F. circinatum infection. The treatment significantly improved seedling survival rates and total phenol content as well as radical scavenging activity ([32]). In the current study, Scots pine was also significantly affected by chitosan. In vitro trials showed an overall negative effect of chitosan on pine’s secondary metabolism. While ex vitro experiments resulted in a varied response, i.e., TFC, chlorophyll b, carotenoids, and radical scavenging activity (DPPH) decreased, and TPC and antioxidant activity as measured by ABTS assay increased. In regard to growth parameters, it was previously reported that chitosan-treated P. sylvestris seedlings had enhanced shoot length, shoot mass and root collar diameter compared with non-treated plants ([35]). Another study also reported enhancements on P. sylvestris growth by using commercial chitosan-based products ([2]). Our research demonstrated that chitosan may also increase shoot height as well as needle length. However, this effect was only observed in vitro and only in pines, as opposed to alders. The effect on alder growth parameters was variable. Ex vitro shoot and root length, and leaf number were all negatively affected, while biomass and leaf width showed positive increases only in certain conditions. The only other study on Alnus glutinosa - chitosan interaction describes biofertilizer use that was beneficial to seedling shoot and root length and weight. While these results were promising, chitosan was only a part of the biofertilizer formula, thus the positive effect the biofertilizer cannot be exclusively attributed to chitosan alone ([5]).
Based on both the above evidences and our results, we can hypothesize that chitosan has two main mechanisms of action. One is linked with the plant’s defensive pathways while the other with direct growth promotion. Based on our findings, both mechanisms were not activated at the same time, and either one or the other was favoured. Overall, growth was not affected for the alders grown in vitro, though the secondary metabolic parameters have increased. Chakraborty et al. ([6]) noted this exact phenomenon and compiled a compelling list of examples in their review on the subject, enumerating several specific ways this may have taken place: osmotic pressure regulation, enzyme and plant hormone activation. All these lead to improved nutrient and water uptake, thus boosting growth ([6], [27] and references therein). The other mechanism is related to the plant defense, where chitosan may directly act as an antimicrobial molecule, eliciting various signals and regulatory processes, though the exact mechanism of action is still being debated.
In our experiment we tested how chitosan at different concentrations and solubilized in different solvents, as well as stored in different conditions can exhibit varied efficacy as a biostimulant. The impact of chitosan on plants can vary depending on the concentration of chitosan used as was noted with tomatoes ([3], [12]), peach ([28]) and strawberry ([1]). Our results also confirmed those reported previously. Interestingly, we noted that the way chitosan was stored and the solvent used for its preparation also had a significant impact on the tested tree seedlings. Likely, the choice of solvent can influence the molecular characteristics of chitosan ([42]). Indeed, we observed that neutralizing the acidic solvent was overall beneficial to plants.
Overall, it is important to carefully evaluate all the variables before using chitosan for growth promotion of tree seedlings. Based on our results, we can surmise that different tree species will likely react differently to chitosan application. Moreover, chitosan may also elicit different responses when applied at different stages of the tree’s development. Nonetheless, we recommend including chitosan as an effective biostimulant for enhancing the in vitro and ex vitro A. glutinosa secondary metabolism, specifically radical scavenging activity, as well as for improving P. sylvestris growth in vitro.
Competing interests
The authors have no competing interests to declare that are relevant to the content of this article.
Data availability
All data will be made available upon request.
Author contributions
MŠ, VSŠ designed and conducted the experiments. MKDS produced the chitosan and consulted on the experimental design. DV consulted on methodology and data analysis. MŠ, VSŠ and DV wrote and revised the manuscript. All authors read and approved the final manuscript.
Acknowledgements
No funding was received to assist with the preparation of this manuscript.
References
Gscholar
Gscholar
Authors’ Info
Authors’ Affiliation
Vaida Sirgedaite-Šežiene 0000-0002-1607-0551
Doroteja Vaitiekunaite 0000-0003-2775-9802
Lithuanian Research Centre for Agriculture and Forestry, Institute of Forestry, Liepu St. 1, Girionys, LT-53101 Kaunas dist. (Lithuania)
MKDS Innovation Co., Piliakalnio st. 68, Nemenčine, LT-15175 Vilniaus distr. (Lithuania)
Corresponding author
Paper Info
Citation
Šilanskiene M, Sirgedaite-Šežiene V, Vaitiekunaite D, Co. MKDS (2024). Chitosan exhibits variable effects on pine (Pinus sylvestris L.) and alder (Alnus glutinosa L.) growth and secondary metabolism. iForest 17: 236-244. - doi: 10.3832/ifor4404-017
Academic Editor
Claudia Cocozza
Paper history
Received: Jun 12, 2023
Accepted: Mar 31, 2024
First online: Aug 06, 2024
Publication Date: Aug 31, 2024
Publication Time: 4.27 months
Copyright Information
© SISEF - The Italian Society of Silviculture and Forest Ecology 2024
Open Access
This article is distributed under the terms of the Creative Commons Attribution-Non Commercial 4.0 International (https://creativecommons.org/licenses/by-nc/4.0/), which permits unrestricted use, distribution, and reproduction in any medium, provided you give appropriate credit to the original author(s) and the source, provide a link to the Creative Commons license, and indicate if changes were made.
Web Metrics
Breakdown by View Type
Article Usage
Total Article Views: 729
(from publication date up to now)
Breakdown by View Type
HTML Page Views: 491
Abstract Page Views: 94
PDF Downloads: 131
Citation/Reference Downloads: 0
XML Downloads: 13
Web Metrics
Days since publication: 18
Overall contacts: 729
Avg. contacts per week: 283.50
Article Citations
Article citations are based on data periodically collected from the Clarivate Web of Science web site
(last update: Feb 2023)
(No citations were found up to date. Please come back later)
Publication Metrics
by Dimensions ©
Articles citing this article
List of the papers citing this article based on CrossRef Cited-by.
Related Contents
iForest Similar Articles
Review Papers
Tectona grandis Linn. f. secondary metabolites and their bioactive potential: a review
vol. 15, pp. 112-120 (online: 26 March 2022)
Research Articles
Links between phenology and ecophysiology in a European beech forest
vol. 8, pp. 438-447 (online: 15 December 2014)
Short Communications
The effects of salicylic acid, oxalic acid and chitosan on damping-off control and growth in Scots pine in a forest nursery
vol. 13, pp. 441-446 (online: 24 September 2020)
Technical Notes
Effect of tree age on chemical compounds of ancient Anatolian black pine (Pinus nigra subsp. pallasiana) needles in Northwest Turkey
vol. 11, pp. 406-410 (online: 15 May 2018)
Research Articles
Secondary metabolites of six Siberian and Crimean Armillaria species and their in vitro phytotoxicity to pine, larch and poplar
vol. 15, pp. 38-46 (online: 04 February 2022)
Research Articles
Chitosan oligosaccharide addition affects current-year shoot of post-transplant Buddhist pine (Podocarpus macrophyllus) seedlings under contrasting photoperiods
vol. 10, pp. 715-721 (online: 27 July 2017)
Review Papers
Breeding and improvement of black locust (Robinia pseudoacacia L.) with a special focus on Hungary: a review
vol. 16, pp. 290-298 (online: 28 October 2023)
Research Articles
Intra-annual tree growth responds to micrometeorological variability in the central Amazon
vol. 14, pp. 242-249 (online: 21 May 2021)
Research Articles
Coping with spring frost-effects on polyamine metabolism of Scots pine seedlings
vol. 10, pp. 227-236 (online: 27 January 2017)
Research Articles
Genetic control of intra-annual height growth in 6-year-old Norway spruce progenies in Latvia
vol. 12, pp. 214-219 (online: 25 April 2019)
iForest Database Search
Search By Author
Search By Keyword
Google Scholar Search
Citing Articles
Search By Author
Search By Keywords
PubMed Search
Search By Author
Search By Keyword