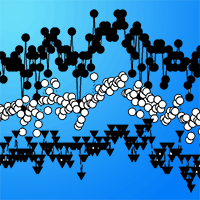
Variations in the performance of hybrid poplars subjected to the inoculation of a microbial consortium and water restriction
iForest - Biogeosciences and Forestry, Volume 16, Issue 6, Pages 352-360 (2023)
doi: https://doi.org/10.3832/ifor4378-016
Published: Dec 13, 2023 - Copyright © 2023 SISEF
Research Articles
Abstract
There is an increasing interest in using plant growth-promoting rhizobacteria (PGPR) to improve hybrid poplar performance under water stress conditions. We assessed the growth and leaf-level physiological responses of different hybrid poplar clones to the inoculation of a microbial consortium and subjected to moderate water shortage. In a nursery experiment, growth, leaf gas exchange, and biomass partitioning traits were assessed during one growing season on twenty hybrid clones from interspecific crosses of Populus trichocarpa × P.deltoides and (P. trichocarpa × P. deltoides) × P. deltoides, which were submitted to two treatments of PGPM inoculation (Inoculated, Inoc_1 vs. non-inoculated, Inoc_0) and two irrigation treatments (full water vs. water restriction). The water restriction decreased shoot growth, photosynthetic rate (Asat), and stomatal conductance (gs); increased intrinsic water use efficiency (WUEint) of the hybrid clones but it did not change the root-to-shoot ratio. Unlike our expectations, by the end of the study, treatment Inoc_1 slightly decreased basal diameter (D) and height (H) relative to Inoc_0 (5.8% and 5.2 %, respectively). Moreover, seven clones significantly decreased the root biomass by 37% to 62% in the Inoc_1 relative to Inoc_0 treatment, while the other clones showed no response to the inoculation. Oppositely, while most of the clones showed no response to the Inoc_1 treatment on leaf-physiological traits compared to Inoc_0, some of them exhibited an increase of Asat of 15% to 39%. Overall, the consortium applied did not improve the responses to the water restriction, and responses to the inoculation were more associated with a deleterious than a growth-promoting effect, which is discussed in the context of nutrient immobilization, application method, and timing.
Keywords
Biomass Partitioning, Leaf-physiological Traits, PGPM Inoculation, Poplar Clones, Water Stress
Introduction
There are concerns about how the decrease in precipitation and the increase in temperature due to climate change ([4]) will affect the productivity of crops and forests in Mediterranean-type climate zones ([11]). Since the green revolution, high gains in plant productivity have been achieved by applying intensive cropping systems using agrochemical and genetic manipulation ([20], [5]). However, the future climate scenario may limit further gains in productivity. Some of the adaptation strategies to a changing climate are the incorporation of more plastic genotypes into production and matching them with more sustainable cropping and silvicultural systems to optimize productivity ([11]). Managing the soil microbiome (i.e., plant growth-promoting rhizobacteria, PGPR) is recognized as being part of these strategies ([41]). The microbiome of the rhizosphere is responsible for essential processes affecting plant life and health ([3]). Because most studies on plant growth-promoting rhizobacteria (PGPR) are carried out in controlled laboratory conditions and using pure culture isolates, there is still little knowledge about the factors that could limit their efficacy in operational conditions (i.e., nursery, field), which depends on complex interactions of PGPR with specific crops, plant genotypes, soils, and climate.
Currently, PGPR genera such as Bacillus, Pseudomonas, Rhizobia, Glomu, Azospirillum, Azotobacter, among others, can be found in commercial products ([5]). These can be applied as individual strains, but sometimes greater effects have been reported when a bacterial mixture (i.e., consortium) is used ([28], [9]). The beneficial microbiome associated with roots and plant components alleviates environmental stress by a variety of mechanisms ([42]). Among them, PGPR can directly enhance nutrients uptake, and increase growth by changing the concentration of growth regulators and producing antibiotics that trigger resistance to pathogens ([25], [5], [26]). It has also been reported to improve the ability to counteract drought stress, inducing a higher antioxidant activity, chlorophyll content, osmolyte production ([5], [32]), water use efficiency, stomatal control ([10]), and improving soil hydraulic properties by producing exopolysaccharides ([49]), and enhance lateral roots and roots hairs development ([42]).
Some evidence shows that the complexity of hosts by microbiome interaction overpasses the plant species level, and goes to the specific genotype level (i.e., genotypic variation in the responses to PGPR). This type of interaction has been shown in many crops, for example, soybean (Glicine max [L.] Merr. - [43]), Boechera stricta ([44]), cotton lines ([35]), maize inbred lines ([34]), potato (Solanum tuberosum L. - [40]), wheat (Triticum aestivum L.), and some forest species such as hybrid poplars ([14], [18], [22]). This specificity is mainùly associated with the quality and composition of exudates released by different individual genotypes ([1], [38]). Other studies have shown some transient growth reduction in the crop plant due to N depletion by PGPR ([6], [2]), which may also contribute to the differential responses of specific genotypes to the inoculation of PGPR.
Poplar species and their hybrids are planted in about 70 countries, adapting to a wide variety of edaphoclimatic conditions ([47]), including Mediterranean-type zones such as central Chile ([46]). Moreover, commercial hybrid poplars (Populus spp.) might be a promising source of raw material for the wood-processing industry and biomass production in the country, and there have been some efforts to broaden the low genetic diversity of hybrid poplars ([48], [46]). However, the lower precipitation projected under the climate change scenario may compromise the productivity and profitability of these plantations. Thus, incorporating more sustainable practices to reduce the use of fertilizers and increase plant tolerance to drought are needed. The objective of this study was to assess the growth and leaf-level physiological acclimation of different hybrid poplar clones subjected to the inoculation of a microbial consortium and under water restriction in nursery conditions. We hypothesized that (i) the growth and physiological performance of hybrid poplars under water restriction is improved by the inoculation of the PGPR consortium, and (ii) those responses are genotype-specific. Understanding the success or failure of applying PGPR on the growth of specific hybrid poplar clones may guide the use of this biotechnology in the development of genotype-specific management prescriptions.
Materials and methods
Plant material and study design
The study was conducted at the nursery facilities of the Centro Tecnológico del Álamo (CTA) at the Universidad de Talca, Chile (35° 24′ S, 71° 38′ W, 112 m a.s.l.). In winter 2018, plant material of ten poplar hybrids for each of the two interspecific crosses, (P. trichocarpa × P. deltoides) × P. deltoides and P. trichocarpa × P. deltoides, were obtained from the CTA’s clonal bank. Clones under study were generated by the hybridization program led by the former Poplar Molecular Genetic Cooperative (PMGC) in Seattle, WA, USA ([48]), and introduced in Chile in 2002. The plant material used in this study corresponds to a subset of experimental clones that have shown high growth potential in selection trials in Chile, which was propagated using 15-cm unrooted cuttings with diameters of 1 to 2.5 cm. The nursery experiment was established in October 2018 under outdoor conditions of light and temperature. Cuttings were planted in 10 L black plastic bags containing a substrate with a mixture of DSM2 peat (Kekkilä Professional Inc., Vantaa, Finland), coconut fiber (Golden Grow by Projar, Valencia, Spain), and perlite (particle size 0.5-3 mm) in a volume proportion of 50%, 35% and 15%, respectively. The peat is commercialized with a small amount of fertilizer NPK (15-12-29) plus microelements in a dose of 1 kg m-3. The substrate analysis showed a NPK concentration of 1.06%, 1.35%, and 0.9%, respectively, organic matter 85%, pH 6.7, and C:N ratio of 42.7.
The experimental design was a split-split plot design, arranged as a complete randomized design (CRD), with five replicates. Two irrigation treatments were the whole plot (full water vs. water restriction), two treatments of bacteria inoculation were the split-plot (Inoculated Inoc_1 vs. non-inoculated Inoc_0), and 20 clonal hybrid poplars were the split-split plot (5 replicates × 2 irrigation treatments × 2 inoculation treatments × 20 hybrid poplars = 400 plants). The treatment of water restriction was exposed at the end of February 2019 (peak of maximum temperatures during summer 2019 - Fig. 1a). We referred water restriction level as mild, as predawn water potential at the end of the treatment application reached -0.63 Mpa, as it is described below. Fig. 2 summarizes the timeline of the nursery experiment. The average substrate moisture percentage achieved for the full water and water-restricted treatments was 42% and 23%, respectively (Fig. 1b). The soil moisture was monitored with a Thetaprobe™ soil moisture sensor (Delta-T Devices Ltd., Cambridge, UK) on two pots per clone and irrigation treatment. To further characterize the water stress condition, at the end of March, we randomly selected 5 plants per watering treatment and measured predawn water potential using a pressure chamber (PMS instrument Co., Albany, OR, USA). Daily irrigation was provided and controlled by an automatic-drip irrigation system and controlled according to soil moisture monitoring. The irrigation rate during the study period varied from 0.3 to 0.6 L day-1 plant-1.
Fig. 1 - Daily maximum, minimum, and mean air temperature (a), and substrate moisture (%) for the full (Full water) and restricted (Water restriction) water treatments (b) for the duration of the experiment.
Fig. 2 - Timeline for the study period (October 2018 to April 2019). Dots show measurement dates, while continuous and discontinuous arrows represent the beginning of the inoculation and irrigation treatments, respectively. The day scale shows the days since planting.
The inoculation treatment (Inoc_1) consisted of applying a soil living microbial consortium, which is commercialized as a lyophilized product with the name of OIKO-BAC 174 (Oikos Chile Ltda., Santiago, Chile). Its composition, expressed in colony-forming units (CFU g-1), included Bacillus subtilis (3.5×108), Bacillus licheniformis (5×107), Bacillus megaterium (3.5×108), Bacillus polymyxa (3.5×108), Bacillus macerans (3.5×108), Pseudomonas fluorescens (3.75×108), Pseudomonas putida (3.75×108), Nocardia corallina (5×108), Saccharomyces cerevisiae (5×108), and Trichoderma viride (2×105). We prepared a solution containing 200 gr of OIKO-BAC 174, 2 k of sugar cane molasses, and 100 L of water according to the manufacturer’s guidelines. The solution was further spread on the substrate at a dose of 400 ml per plant using a graduated glass. Treatment application was carried out before dawn to avoid potential damage of bacteria by light. The first application was made 65 days after planting to ensure root development and increase the rhizosphere zone. At that time, plants had an average height of 40 cm and most adventitious roots had over 5 cm length, which was verified by sampling 5 plants. The application was repeated three times during the growing period, every three weeks (December 2018 to February 2019 - Fig. 2). On the same dates of inoculation, plants in the control treatment (Inoc_0) also received an additional dose of 400 ml of water.
Growth, leaf-level physiology, stem and root biomass
Growth and leaf-level physiological measurements were obtained on five instances during the study period (November 28, 2018; December 12, 2018; January 15, 2019; March 14, 2019; April 4, 2019). At each date, growth traits were measured in all the replicates, whereas the leaf physiological and biomass traits were measured in only 3 of the 5 replicates per treatment combination. Plant basal diameter (D) and height (H) were measured above 3 cm of the cutting insertion, using a caliper and a metric tape, respectively. Net saturated photosynthesis rate (Asat, μmol m-2 s-1), stomatal conductance (gs, mmol m-2 s-1) and intrinsic water use efficiency (WUEint, μmol mmol-1) were measured for one fully expanded leaf in the upper part of the plant, using a portable gas exchange system LI 6800® (LICOR Inc., Lincoln, NE, USA). Initial chamber conditions were set up at ambient conditions during measurements, with a temperature of 20 °C, CO2 concentration of 400 ppm, relative humidity of 50%, and PAR of 1800 µmol m-2 s-1. Measurements were performed between 09:00 and 12:00 a.m. local time. At the end of the experiment, the dry biomass for stems (SDW) and roots (RDW) was determined by harvesting the plants, detaching the components, and drying them in an oven at 65 °C for 48 hours. Additionally, we calculated the roots-to-shoot ratio (RSR). Once the plants were harvested, three samples of substrate were obtained per each combination of irrigation and inoculation treatment for further analysis of microbial activity. The samples were sent to the Soil Laboratory of the Universidad de Concepción, and the soil microbial activity was determined by fluorescein diacetate (FDA) hydrolysis, as described by Mutumba et al. ([30]). Microbial activity was expressed in µg Fluorescein g-1 dry soil.
Statistical analysis
Data analyses were made with the ASReml 4 package implemented in the R software (ASReml-R, VSN, UK). For growth and leaf-physiological traits, all the explanatory variables (irrigation treatment, inoculation treatment, hybrid clone, date, and the interactions among factors) were considered fixed effects. We constrained the model to third-order interactions, whereas the fourth-order interaction (Irrigation × Inoculation × Clone × Date) was pooled in the error term. Because measurements were unequally spaced over time, we used an exponential power structure to model the variance-covariance matrix of correlated residuals effects. This structure was previously selected over the unstructured based on the Akaike information criteria. For biomass traits, which were measured once at the end of the experiment, the same analysis was run, excluding the effect of date and its interaction with the other factors. The FDA data was analyzed as a factorial design with irrigation and inoculation treatment considered fixed. We correlated the clonal means for growth and biomass traits obtained at the end of the experiment (i.e., cumulated growth) with means for leaf-physiological traits over the study period, using the Pearson’s coefficient of correlation. Significant differences were considered at a probability level of 0.05.
Results
Variability in growth and biomass partitioning
Differences in D and H among the poplar clones varied by date (Date×Clone interaction, P<0.05), and were detected since the first measurement date (51 d after planting - Tab. 1, Fig. 3a, Fig. 3b). Differences among clones with time were greater for H than D (Fig. 3a, Fig. 3b). Particularly, two clones (T×D 1 and TD×D 1) exhibited a remarkable fast-growing in H. By the end of the study period, the fast-growing clone showed higher D (14%) and H (69%) than the slow-growing clone. As expected, the growth on H slowed down toward April (by the end of the growing season). However, low growth in D was observed from January to March (midsummer in the Southern Hemisphere), with a slight increase toward April (Fig. 3a, Fig. 3b).
Tab. 1 - P-values on the analysis of variance for basal diameter (D), total height (H), root to shoot ratio (RSR), shoot dry weight (SDW), root dry weight (RDW), light-saturated photosynthetic rate (Asat), stomatal conductance (gs), intrinsic water use efficiency (WUEint). The effects are irrigation treatment (Irrig), inoculation treatment (Inoc), clone and date.
Source of variation | Stem growth | Biomass partitioning | Leaf-level physiology | |||||
---|---|---|---|---|---|---|---|---|
D | H | RSR | SDW | RDW | A sat | g s | WUEint | |
Irrig | 0.6938 | 0.8798 | 0.9222 | 0.0490 | 0.0006 | 0.7744 | 0.2787 | 0.2250 |
Inoc | 0.0027 | 0.0232 | 0.1541 | 0.0015 | <0.0001 | 0.0489 | 0.4035 | 0.8303 |
Irrig×Inoc | 0.6197 | 0.5299 | 0.6800 | 0.5870 | 0.2080 | 0.6294 | 0.6275 | 0.4269 |
Clone | <0.0001 | <0.0001 | <0.0001 | <0.0001 | <0.0001 | <0.0001 | <0.0001 | <0.0001 |
Clone×Irrig | 0.2408 | 0.3470 | 0.1583 | 0.6184 | 0.5017 | 0.0136 | 0.3984 | 0.9571 |
Clone×Inoc | 0.1802 | 0.6092 | 0.0526 | 0.5243 | 0.0046 | 0.0473 | 0.8759 | 0.9161 |
Clone×Inoc×Irrig | 0.5930 | 0.9371 | 0.3923 | 0.7986 | 0.4530 | 0.5642 | 0.4834 | 0.3729 |
Date | <0.0001 | <0.0001 | - | - | - | <0.0001 | <0.0001 | <0.0001 |
Irrig×Date | <0.0001 | 0.3937 | - | - | - | 0.0012 | 0.0027 | 0.0080 |
Inoc×Date | 0.0071 | 0.0001 | - | - | - | 0.1747 | 0.0571 | 0.2144 |
Date×Clone | 0.0015 | 0.0000 | - | - | - | 0.0001 | 0.0373 | <0.0001 |
Irrig×Inoc×Date | 0.5630 | 0.4567 | - | - | - | 0.1691 | 0.0655 | 0.0745 |
Date×Clone×Irrig | 0.9389 | 0.1256 | - | - | - | 0.8934 | 0.9878 | 0.9480 |
Date×Clone×Inoc | 0.9687 | 0.8667 | - | - | - | 0.5859 | 0.8332 | 0.7644 |
Fig. 3 - Means for basal diameter (D) (a) and height (H) (b) over time per poplar clone. D (c) and H (d) over time per inoculation treatment (control Inoc_0 and inoculated Inoc_1). D (f) and H (g) over time per irrigation level (full water and water restriction). (*): significant differences (p<0.05) between inoculation treatments at a specific date. The continuous and discontinuous arrows represent the beginning of the inoculation and water restriction treatments, respectively.
The first application of the microbial consortium started 65 d after planting, and the varying response of D and H to the inoculation treatments and date (Inoc×Date interaction, P<0.05) were detected since the third measurement date (34 d after inoculation - Fig. 3c, Fig. 3d). Contrarily with our expectations, the inoculated treatment (Inoc_1) had a slight but significant decrease in D and H relative to the control treatment (Inoc_0). By the end of the study period, Inoc_1 decreased D and H by 5.8% and 5.2% relative to Inoc_0. On the other hand, the water stress treatment was imposed toward the end of summer (164 d after planting and 77 d after applying the inoculation treatment). Overall, the water restriction had a small effect on the growth traits, decreasing D by 4.9%, but with no effect on H (Fig. 3e, Fig. 3f). The fact that the clone interacted with neither the inoculation nor the irrigation treatment implies that the clonal responses for growth were fairly stable at this stage.
Significant differences among the poplar clones were found for biomass components, which did not depend on the irrigation treatment (Tab. 1, Fig. 4a, Fig. 4b). Overall, both interspecific crosses (T×D and TD×D) showed a similar range of variation for RSR at the clone level (Fig. 4). The lowest and highest RSR was 1.4 for clone T×D 1 and 3.6 for clone T×D 2, respectively (157% higher in T×D 2 - Fig. 4a). Similarly, significant differences among clones were found in SDW, with values ranging from 4.7 g (TD×7) to 12.6 g (T×D 1). This trait was also affected by inoculation and irrigation treatments (Tab. 1). The treatment Inoc_1 significantly decreased SDW by 15% relative to Inoc_0 (Tab. 1), with mean values for Inoc_0 and Inoc_1 of 8.1 g and 6.9 g, respectively. The water restriction treatment significantly decreased SDW by 18% relative to the full water treatment, with mean values for the full and restricted water treatment of 8.2 and 6.8 g, respectively.
Fig. 4 - Means for root to shoot ratio (RSR) (a) and shoot dry weight (SDW) (b) per hybrid clone, and root dry weight (RDW) (c) per clone and inoculation treatment (control Inoc_0 and inoculated Inoc_1). Clones in the graphs were ordered by interspecific crosses and ascending values of RSR. (*): significant differences (p<0.05) between inoculation treatments at a specific date.
Particularly, the differences in RDW among clones varied by inoculation treatment (Clone×Inoc interaction - Fig. 4c). Seven clones (T×D: 5, 6, 8, 9 and TD×D: 6, 7, 8) significantly decreased RDW by 37% to 62% in the Inoc_1 relative to the Inoc_0 treatment, while the other clones showed no response to the microbial consortium inoculation (Fig. 4c). Those seven clones corresponded to the ones that had the highest RDW in the control treatment Inoc_0. The water restriction treatment significantly decreased RDW by 16% relative to the full water treatment, with mean values for the full and restricted water treatment of 17.9 and 15.1 g, respectively.
Variability in leaf-level physiological parameters
All the gas exchange parameters significantly varied by clone and time (Date× Clone interaction, P<0.05 - Tab. 1, Fig. 5a-c). Differences among clones were detected since the first measurement date for gas exchange parameters (65 d after planting). The clonal responses of the physiological parameters over time were diverse (Fig. 5a to Fig. 5c). For instance, in summer (between December and March) some clones maintained Asat constant, while others increased or decreased their value within the period. Meanwhile, at the end of the experiment (April), on average, all clones decreased Asat by 20% relative to the March measurement (Fig. 5a). Similarly, during the study period, both gs and WUEint fluctuated more for some clones than others. WUEint peaked in January for some clones, while a few ones peaked in March. Some clones considerably decreased gs from December to January, which explained the peak of WUEint in January for these clones, whereas other clones were fairly stable throughout the study period.
Fig. 5 - Means for saturated photosynthetic rate (Asat) (a), stomatal conductance (gs) (b), and intrinsic water use efficiency (WUEint) (c) over time per poplar clone and Asat (d), gs (e) and WUEint (f) over time per irrigation level (full water and water restriction treatment). (*): significant differences (p<0.05) between inoculation treatments at a specific date. The continuous and discontinuous arrows represent the beginning of the inoculation and water restriction treatments, respectively.
The water restriction treatment imposed at the end of February had a transient influence on the gas exchange parameters in March but not in the April measurement (Irrig×Date interaction, P<0.05 - Tab. 1, Fig. 5d-f). Mean predawn water potential for the full water and water-restricted treatment were -0.28 MPa and -0.63 MPa, respectively. In March, the water restriction treatment decreased Asat and gs and by 12% and 30%, and increased WUEint by 26% relative to the full water treatment, respectively. On the other, hand, the clone response on Asat differed by inoculation treatment (Tab. 1, Fig. 6). Four clones significantly increased Asat from 15% to 39% in the inoculated treatment.
Fig. 6 - Means for saturated photosynthetic rate (Asat) across the study period by poplar clone and inoculation treatment (control Inoc_0 and inoculated Inoc_1). Clones in the graphs were ordered by interspecific cross and ascending values of Asat in the full irrigation treatment. (*): significant differences (p<0.05) between inoculation treatments at a specific date.
Measurements of microbial activity on the substrate
The microbial activity measured in the substrate by the end of the experiment showed no significant differences between the inoculation treatments (P=0.3806). FDA means (± standard errors) for the Inoc_0 and Inoc_1 treatment were 16.0 ± 1.78 and 17.8 ± 1.64 µg g soil-1 h-1, respectively. However, there was a significant increase in the FDA due to the water restriction treatment relative to the full irrigation treatment (P=0.0379). FDA means and standard errors for the full irrigated and water restriction treatment were 14.5 ± 1.92 and 19.3 ± 0.42 µg g soil-1 h-1, respectively.
Discussion
The use of PGPM has been recognized as a sustainable management tool that could improve the productivity of different crops and plant species, especially when they face biotic and abiotic stresses ([28], [5]). Contrary to our expectations and initial hypothesis, the PGPR inoculation did not improve the clones’ growth under the mild water restriction imposed. In this study, there were no significant interactions between the hybrid poplars with neither the inoculation nor the irrigation treatment on the growth traits. This implies that all clones responded in a similar direction and relative magnitude to those treatments (i.e., co-directional response). The tested hybrid clones belong to interspecific crosses with great growth potential in Chile, and with high clonal variation within the crosses ([46]). Differences in growth among clones appeared early after planting, and the fastest-growing clone had 69% higher H than the slowest-growing clone. Overall, plants’ growth in the trial was considered low, but it was in the range of other similar studies ([22]). During the experiment, we provided water daily. Thus, the low growth observed in the trial was likely due to stresses by the high temperatures during the study period (Fig. 1a), and by potential nutrient deficiency, as discussed below. The growth rate for H increased from November to March and decreased toward April (the end of the growing season). However, the growth in D stagnated from January to March and then recovered toward April. Growth rate for stem diameter might decrease during the growing season, while plants face adverse climate conditions and recover later ([33]), which coincides with our findings.
Several studies have reported the beneficial effects of applying PGPR on different plant species ([25], [28], [5], [22], [26]). Nevertheless, in this study, the aboveground growth and biomass were slightly but significantly decreased over the study period by the inoculation with the microbial consortium, which disagreed with our first hypothesis. Several reasons may explain a transitory reduction of growth due to the PGPR. First, at the end of the study period, the C:N ratio in our study was 37, which was quite high due to the nature of the substrate used. It has been documented that C:N ratio over 25 makes that soil microbes may deplete N in the solution and compete with plants for this element (i.e., immobilization), as it might have occurred in this study ([45]). Growing mediums based on peat and coconut fiber are common in horticulture but not in forest nurseries. Although the blend of those media may improve the environment (i.e., moisture, aeration, porosity, and pH) for microbial communities, the microbes may face extreme nutrient imbalances due to the higher C:N ratios in the substrate relative to the ones on microbes ([16]), with the consequent negative effect on poplar growth. Some studies have shown little microbial respiration in peat ([7]), whereas both peat and coconut are high in lignin content, and thus highly recalcitrants ([16]). As long the decay of organic matter proceeds, the C:N ratio decreases, and mineralization increases, which varies in time depending on the recalcitrance of the organic matter ([45]). Other studies highlight that the effectiveness of applying PGPR on plant growth may not be achieved if nutrient limitations are present ([13], [27]).
A second reason may be related to the short experimental period in our study, which likely covered the phase of slow decomposition rate and depletion of N in the growing media by microbes. For instance, in western red cedar (Thuja plicata Donn.), the inoculation with an endophytic bacteria (Paenibacillus polymyza strain) reduced growth of 9-month old seedling relative to the control non-inoculated, but this was reversed when seedlings were 13-month old ([2]). The authors claimed that this effect was due to the low N in the growth medium, which was not enough to trigger the biological fixation of N, depleting N, and restraining seedling growth. On the other hand, although plant N concentration might increase due to biological fixation, it might not imply an immediate effect on plant growth. Bal & Chanway ([6]) found that after seven months, three diazotrophic bacteria increased the foliar N on western red cedar compared to the non-inoculated control. However, one bacteria strain decreased seedling dry weight relative to the control, while the other showed no differences. In hybrid poplar clones of Populus euroamericana and Populus deltoides × P. nigra, Jang et al. ([22]) found that clonal responses in D and H to inoculation with a strain of Bacillus subtilis varied from 1 to 5 months. Compared to our study, we argue that the faster effect of the inoculation found in the study by Jang et al. ([22]) was due to the use of autoclaved soil (organic matter 2% and N 0.15%) instead of a substrate with high C:N ratio, and to the higher frequency of applications of the inoculant (12 times per month). On the other hand, rhizobacteria may act as PGPR or as deleterious rhizobacteria (DRB) depending on the environmental conditions ([31]). Ciccillo et al. ([8]) found that the effect of a strain of Burkholderia ambifaria on the growth of maize plants depended on the application method acting as PGPR when the inoculum was applied to the seed and DRB when this was applied to the soil, which would also explain our results. The authors mentioned that the effect of B. ambifaria on the preexistence microflora varied with the application method. Thus, maybe the direct application of the consortium to the poplar cuttings at pre-planting might turn out more favorable results, which need further research.
The clonal effect predominated on SDW and RSR, and the considerable variation among clones on RSR suggests large differences in carbon partitioning (Fig. 4). Nevertheless, for RDW we found a significant interaction between the clone and inoculation treatment. Although most of the clones showed a decrease in RDW due to the microbial consortium inoculation, this effect was significant only in seven clones (T×D: 5, 6, 8, 9 and TD×D: 6, 7, 8), with reductions in a range of 37% to 62%. This reduction was much higher than for the growth trait H and D (less than 6%). Our results indicate that the microbial inoculation had higher effects on below than aboveground growth at this early stage. These results partially support our second hypothesis since the responses of hybrid poplars to the inoculation with the consortium were genotype-specific, but more associated with a DRB than PGPR effect as mentioned. Moreover, it highlights the complex interaction that might exist between different plant genotypes and microbial strains, as has been shown in other species such as Triticum aestivum L. ([30]), and hybrid poplar clones ([22]). In a similar experiment on blueberry (Vaccinium corymbosum L.), Schoebitz et al. ([37]) tested the same microbial consortium as in our study (OIKO BAC 174) both alone and in a mixture with humic substances. Their results cannot completely elucidate the effect of the microbial consortium, as it increased RDW and SDW relative to the control non-inoculated but had no effect on D or H, being the mixture the most effective treatment.
The water potential in the water-restricted treatment was moderated and comparable to that reported in other studies ([15]). Because poplar species are recognized to be sensitive to water stress, this mild water restriction was enough to decrease some growth, biomass components, and gas exchange traits. However, we did not observe genotype-specific responses as have been found in other studies ([29]). In our study, the photosynthetic rate was in the range of other studies using hybrid poplars in nursery conditions and grown in pots (from 6 to 12 µmol m-2 s-1 - [22]) but was lower than values obtained in field trials (around 20 µmol m-2 s-1 - [29], [12]). However, when comparing WUEint, the values were much lower than the study by Jang et al. ([22]) but in the range reported by Monclus et al. ([29]). Similar to growth, clones significantly differed in gas-exchange parameters and fluctuated throughout the study period. Fluctuations of WUEint across the period were more related to the fluctuations of Asat than gs
While most clones did not respond to the microbial consortium inoculation on gas-exchange parameters, a few of them significantly increased their photosynthetic rate, which partially agrees with the second hypothesis. Nonetheless, our study cannot clarify the fate of the excess of C assimilated. We expected that an excess of C assimilated would be expressed in more biomass, which was not the case. Unlike, two clones increased the photosynthetic rate but decreased the root biomass due to the inoculation treatment. Bal & Chanway ([6]) explained that the excess of C supply (i.e., sugars, amino acids and organic compounds, dead root-cap cells) from plant to bacteria to drive N fixation might be an expensive process, which may also explain a temporary reduction in growth. Root exudation may explain 10% to 44% of the C assimilated by photosynthesis ([17]). A genetic control on root exudates has been reported, which highlights that the association between plants and PGPM might be genotype-specific ([24]), which needs further research.
Several studies mention the positive effects of PGPR in alleviating drought stress ([42], [21]), a result not observed in our study. The water restriction imposed on plants was expressed on all gas-exchange parameters in March but not in April, which suggests that the mild water restriction did not seriously damage the photosynthetic apparatus, and its effect was likely a transient acclimation. In a study on poplar hybrids of Populus balsamifera × P. trichocarpa Torr. & Gray and P. balsamifera × P. maximowiczii A., established in a growing chamber and using a peat-based substrate, Larchevêque et al. ([23]) found that gs and Asat decreased when the substrate moisture content was lower than 20%. Our experiment was over this threshold, suggesting that the overall response of clones to water restriction in March was due to the high water demand from the atmosphere on those days in which the maximum temperature was over 35 °C. Moreover, there were likely collateral effects of the higher air temperatures on the substrate temperature, affecting water acquisition by roots.
The use of a consortium of microorganisms might be more effective than the use of single bacterial strains ([28]). Although we used a commercial product that contains a consortium of well-known bacteria and fungi, whose benefits for plant growth have been broadly revised in different species ([36], [19], [39]), these consortia did not exert any positive effect on poplar growth. The bacterial activity, measured by the FDA, also did not show differences between the inoculation treatments. This study is not conclusive at all in this respect, and we argue that the microbial starvation for N explained above also impeded the colony to prosper. Mutumba et al. ([30]) studied the effect of Bacillus and Pseudomonas strains applied individually and as a consortium on wheat genotypes under two irrigation treatments. Their results showed that although the consortium had the greatest effect on plant biomass, the microbial activity, measured by both FDA and as microbial respiration, differed from each other and was not entirely consistent with the values for biomass, which highlights the complexities and challenges to be addressed in the study of PGPR. Regarding the multiple factors affecting the PGPR and plant interaction, it is imperative to validate the enormous amount of information of laboratory studies in field conditions. Future short-term studies like ours must consider the use of substrate that minimize nutrient immobilization. Moreover, since effects may be small, it would be recommendable to increase the statistical power (i.e., higher replication) when experiments are run under less controlled conditions. Incorporation less instantaneous traits such as the carbon isotope discrimination and non-structural carbon to address water stress effects may be encouraged.
Conclusions
This study showed significant differences in the growth, biomass, and physiological traits among the poplar hybrid clones, implying a high clonal phenotypic variation. However, contrary to our expectations, the inoculation with the microbial consortium did not improve the hybrid poplar’s performance under water restriction conditions, and both effects were not interacting. Contrarily, the consortium inoculum tended to slightly decrease the aboveground growth traits, and the root biomass in some clones. Thus, the consortium inoculation was associated more with a deleterious (DRB) than a growth-promoting rhizobacteria (PGRB) effect. The water restriction was moderated, but enough to express a decline in growth, gas exchange, and biomass component traits, which suggests no potential differences in the susceptibility to water restriction among these hybrid clones. We also observed that the inoculation increased the photosynthetic rate but decreased the root biomass in some clones, whereas other clones showed no response. These complex interactions observed in this study may be driven by the differential response of the clones to nutrient immobilization and the quality of the exudates, which needs further research. Since poplar production systems must be optimized to produce high yields despite the stresses imposed by climate change, a better understanding of genotype by soil microorganism interactions may play a key role in adapting plants to a water-limited environment.
Conflict of interest
The authors declare that there is no conflict of interest.
Acknowledgements
This research was supported by a post-doctoral grant of the Dirección de Investigación of Universidad de Talca, Chile. Equipment, nursery and laboratory for this were provided by the Poplar Technology Center at the University of Talca. We want to acknowledge the forest engineer, Mr. Javier Urzua, who was in charge of the field campaigns and data management. We also thank Mrs. Lorena Sepúlveda and Patricio Diaz from the company Oikos Chile Ltda., who provided the microbial inoculum and support to installing the experiment.
References
CrossRef | Gscholar
Gscholar
CrossRef | Gscholar
Gscholar
Gscholar
Authors’ Info
Authors’ Affiliation
Carlos Magni 0000-0002-9939-0381
Eduardo Martínez-Herrera
CESAF, Facultad de Ciencias Forestales y de la Conservación de la Naturaleza, Universidad de Chile, Avenida Santa Rosa 11365, La Pintana (Chile)
Departamento de Ciencias Forestales, Facultad de Ciencias Agrarias y Forestales, Universidad Católica del Maule, Avenida San Miguel 3605, Talca (Chile)
Departamento de Silvicultura y Conservación de la Naturaleza, Universidad de Chile, Av. Santa Rosa 11315, Santiago (Chile)
Corresponding author
Paper Info
Citation
Yáñez MA, Espinoza S, Ovalle J, Magni C, Martínez-Herrera E (2023). Variations in the performance of hybrid poplars subjected to the inoculation of a microbial consortium and water restriction. iForest 16: 352-360. - doi: 10.3832/ifor4378-016
Academic Editor
Claudia Cocozza
Paper history
Received: May 11, 2023
Accepted: Sep 30, 2023
First online: Dec 13, 2023
Publication Date: Dec 31, 2023
Publication Time: 2.47 months
Copyright Information
© SISEF - The Italian Society of Silviculture and Forest Ecology 2023
Open Access
This article is distributed under the terms of the Creative Commons Attribution-Non Commercial 4.0 International (https://creativecommons.org/licenses/by-nc/4.0/), which permits unrestricted use, distribution, and reproduction in any medium, provided you give appropriate credit to the original author(s) and the source, provide a link to the Creative Commons license, and indicate if changes were made.
Web Metrics
Breakdown by View Type
Article Usage
Total Article Views: 12826
(from publication date up to now)
Breakdown by View Type
HTML Page Views: 10856
Abstract Page Views: 1007
PDF Downloads: 724
Citation/Reference Downloads: 0
XML Downloads: 239
Web Metrics
Days since publication: 565
Overall contacts: 12826
Avg. contacts per week: 158.91
Article Citations
Article citations are based on data periodically collected from the Clarivate Web of Science web site
(last update: Mar 2025)
(No citations were found up to date. Please come back later)
Publication Metrics
by Dimensions ©
Articles citing this article
List of the papers citing this article based on CrossRef Cited-by.
Related Contents
iForest Similar Articles
Research Articles
Revealing the physiological basis of forester’s choice of poplar clones (Populus spp.)
vol. 17, pp. 156-164 (online: 14 June 2024)
Research Articles
A physiological approach for pre-selection of Eucalyptus clones resistant to drought
vol. 13, pp. 16-23 (online: 15 January 2020)
Research Articles
Climate-wise models of biomass productivity for hybrid poplar clones in Europe
vol. 16, pp. 188-194 (online: 30 June 2023)
Research Articles
Energy production of poplar clones and their energy use efficiency
vol. 7, pp. 150-155 (online: 23 January 2014)
Short Communications
Stomata morphological traits in two different genotypes of Populus nigra L.
vol. 8, pp. 547-551 (online: 16 September 2014)
Research Articles
Growth performance and nitrogen use efficiency of two Populus hybrid clones (P. nigra × P. maximowiczii and P. trichocarpa × P. maximowiczii) in relation to soil depth in a young plantation
vol. 9, pp. 847-854 (online: 22 September 2016)
Research Articles
Variability of tolerance of Wild cherry clones to PEG-induced osmotic stress in vitro
vol. 15, pp. 265-272 (online: 25 July 2022)
Research Articles
Links between phenology and ecophysiology in a European beech forest
vol. 8, pp. 438-447 (online: 15 December 2014)
Research Articles
Soil nutrient status, nutrient return and retranslocation in poplar species and clones in northern Iran
vol. 6, pp. 336-341 (online: 29 August 2013)
Research Articles
Salinity strongly drives the survival, growth, leaf demography, and nutrient partitioning in seedlings of Xylocarpus granatum J. König
vol. 10, pp. 851-856 (online: 26 October 2017)
iForest Database Search
Search By Author
Search By Keyword
Google Scholar Search
Citing Articles
Search By Author
Search By Keywords
PubMed Search
Search By Author
Search By Keyword