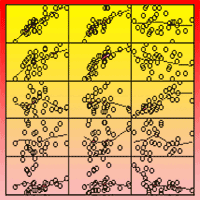
Adaptability and interspecific variability in growth and leaf traits of eucalypt
iForest - Biogeosciences and Forestry, Volume 14, Issue 6, Pages 560-568 (2021)
doi: https://doi.org/10.3832/ifor3660-014
Published: Dec 09, 2021 - Copyright © 2021 SISEF
Research Articles
Abstract
Effective adaptability of plants to new environments can be analysed in terms of survival rate. Analysing the traits that favour adaptation to environmental changes provides a more in-depth understanding of the mechanisms involved. Local adaptation occurs because different environmental factors exert selective pressure across habitats. Understanding the leaf mechanisms underlying plant survival and growth is crucial to determine why local adaptation involves trade-offs. A comparative provenance test on 29 eucalyptus species was conducted to improve our understanding of species adaptation strategies on coastal plains of Pointe-Noire, Republic of the Congo. We studied the different functional traits to determine how plants function and to highlight the different species’ adaptive strategies. For each species, survival, growth traits and leaf traits were measured, and the climatic factors of the origin area for each species was taken into account. Cluster analysis was performed on groups of species with a similar growth strategy. The results revealed general trends that explain the physiological mechanisms involved in the species’ local adaptation. Indeed, species have survived to current environmental changes by adjusting their specific leaf area plasticity. The 32 provenances of eucalyptus were subdivided into four groups by cluster analysis. The first cluster included two species (E. pilularis and E. peltata) that are totally unsuited to the local conditions in Pointe-Noire, with the slowest growth rate and smallest specific leaf area. The second cluster contained species that showed a wide variety of growing strategies, allowing them to adapt to local conditions. The third cluster included a species that is specialised in obtaining large quantities of resources, while investing very little in growth. The fourth cluster included species that acquired and used resources at a slow rate. Leaf anatomy was quite responsive to climatic conditions. We evaluated the different strategies and found that eucalyptus species had very diverse functional traits, which may explain their broad ecological range.
Keywords
Adaptability, Eucalyptus, Foliar Traits, Growth Strategies, Clustering Analysis
Introduction
The predictions suggest that Africa will be severely impacted by climate change. There is a significant risk that many forest ecosystems will not have the adaptive capacity to supply vital goods and services ([12]). To face global climate changes, species must adapt to new environments ([20], [13]). Thus, although this is a daunting task, understanding the capacity of species to adapt is increasingly important for conservation and biodiversity management ([29], [8]). Such task entails the study of morphological, physiological and phenological characteristics involved in the ecological strategies adopted in response to environmental factors ([35]).
The study of functional traits, especially in plants, has a long tradition in ecological research ([45]). Leaf traits may reflect adaptation mechanisms of plants to the environment; in particular, leaf morphological traits like specific leaf area, leaf thickness and leaf density may better reflect the changes in environmental factors ([2]) such as temperature, light intensity and water status. Conversely, environmental changes can led to unprecedented changes in ecosystems including plant growth strategies.
The trade-offs between functional traits depends on how plants acquire, use and conserve the resources ([37]). The plasticity in functional traits is responsible for much of the phenotypic variation observed in non-native populations, and allows introduced species to grow successfully in new conditions, despite the limited genetic variation ([9]). Genetic diversity within and among stands is the linchpin for current and future forest development, and is crucial for the survival and adaptation of species ([22]).
The current production context which involves unsustainable land use changes, as well as future climate change scenarios, call for more detailed studies on local varietal adaptation in order to reduce the use of land and optimize future timber production. In this context, the eucalyptus improvement programme in the Congo should move towards the selection of plastic genotypes with a more efficient use of resources.
Adapting to different environmental conditions entails costly fitness trade-offs ([46]). Assisted migration involving individuals or populations of a species is potentially an important strategy to respond to climate change, though this approach is not widely used ([11]). In general, several Eucalyptus species show remarkable plasticity, which allows adaptation to hostile environments, especially nutrient-poor soils ([5]) and hot arid areas ([38]). Although certain species are known to better perform on poor soils ([23]), few studies focused on the physiological basis of this ecological feature.
In the Congo, the first attempts to introduce eucalyptus date back to 1953. Subsequently, several species and provenance trials have been conducted. Several studies on their productivity revealed that only some species were adapted to local conditions ([16]). However, leaf relationships explaining the species’ responses have not been studied in the Congo, especially in Pointe Noire, where eucalyptus plantations have been established.
This study aims to understand the local adaptation mechanisms of 29 eucalyptus species using leaf traits in the Republic of Congo. We asked three research questions: (i) Do functional traits vary among eucalyptus species at different ages? (ii) What are the trade-offs among functional traits? (iii) Can the potential adaptation of eucalyptus species in the Pointe-Noire conditions be determined by examining the relationships between growth and functional traits?
Material and methods
Study site and sampling
This study is based on data from a provenance trial conducted at the Kissoko forestry station (04° 45′ 51″ S, 11° 59′ 21″ E) in the south of Republic of the Congo. Mean annual rainfall is approximately 1200 mm; daily temperature is 25-26 °C in the rainy season (October to May) and 22-23 °C in the dry season (June to September). The average monthly rainfall during the rainy season ranges from 83 to 92 mm, and during the dry season from 1 to 20 mm ([19]). Relative humidity is high (85%) with low seasonal variability (2%). The soil is ferralitic and highly desaturated in bases ([32]).
We conducted the present study in a Eucalyptus provenance trial which included 32 provenances belonging to 29 species (Tab. 1). The seeds used for seedling production were collected from different parts of Australia (North, North East, East and South East coasts) and Indonesia (Fig. 1).
Tab. 1 - List of studied species and their subgenera.
Species code |
Subgenus | Species | Provenance |
---|---|---|---|
1 | Alveolata | E. microcorys | Beerburrum (Australia) |
2 | Blakella | E. tesselaris | Mareeba (Australia) |
3 | Corymbia | E. citriodora | Ord Irvinbanck (Australia) |
4 | E. maculata | Rockhampton (Australia) | |
5 | E. nesophila | Jimmy’s Creek, Coboutg Peninsula (Australia) | |
6 | E. peltata | Mantuan (Australia) | |
7 | E. polycarpa | Est Gue Goyder river (Australia) | |
8 | E. torelliana | Flaggy Creek, QLD (Australia) | |
9 | Eucalyptus | E. acmenoides | Wild Cattle (Australia) |
10 | E. phaeotricha | Noosa Heads (Australia) | |
11 | E. pilularis | Moleton (Australia) | |
12 | E. umbra | Elliot river (Australia) | |
13 | Eudesmia | E. tetrodonta | SE Maningrida (Australia) |
14 | Idiogenes | E. cloeziana | Gympie (Australia) |
15 | Minutifructus | E. raveretiana | Exe Creek, Ouest Mckay (Australia) |
16 | Symphyomyrtus | E. alba | Sud Cooktown (Australia) |
17 | E. alba | Natar Bora (Timor) | |
18 | E. apodophylla | Ouest Pentecost river, Gibb River (Australia) | |
19 | E. bigalerita | S-O Katherine Kununurra river (Australia) | |
20 | E. brassiana | Cape York peninsula, Coen (Australia) | |
21 | E. exserta | Mckay Rockhampton (Australia) | |
22 | E. grandis | Est Atherton, QLD (Australia) | |
23 | E. paniculata | Nord Raymond Terrasse (Australia) | |
24 | E. pellita | Pinnacle (Australia) | |
25 | E. propinqua | Clouds Creek SF Grafton (Australia) | |
26 | E. punctata | Barakula SFNW Chinchilla (Australia) | |
27 | E. resinifera | 10km Ravenshoe (Australia) | |
28 | E. robusta | Noosa (Australia) | |
29 | E. tereticornis | Helenvale (Australia) | |
30 | E. urophylla | Mte Lewotobi (Indonesia) | |
31 | E. urophylla | Flores Arbau Ulu (Indonesia) | |
32 | E. urophylla | Arafalaca, Ouest Alor (Timor) |
Tree measurements
The experimental design consisted of 4 blocks of 128 plots (32 per block); each plot contains 9 trees representing a single provenance. The plots were randomly distributed into the blocks. Originally, each plot was planted with spacing of 4.70 × 2.65 m (about 800 trees ha-1). Trees were measured at two dates: after 15 months (1.2 years) since their establishment (in February during the short dry season) and after 54 months (4.5 years, in April during the main rainy season).
We first evaluated the survival rate (SR, %) of the trees at each age at the plot level, and the difference in survival between 54 and 15 months (eqn. 1):
where nlive is the number of live trees at the plot level and ntot is the total number of tree established in the plot. On each tree, we performed non-destructive quantitative measurements including collar circumference, total height and leaf measurements. Collar circumference (CC, cm) was measured with a tape around the trunk base. Total tree height (HT, m) was measured with a Vertex® IV (Haglöf, Långsele, Sweden) hypsometer at a distance approximately equivalent to tree height. Leaf variables, including specific leaf area (SLA), leaf thickness (LT, mm) and leaf density (LD, kg m-3) were measured for 10 leaves per tree harvested from the upper and lower crown. The 10 adult leaves were chosen with a fully expanded blade (juvenile and senescent leaves were excluded) and free of damage. Leaf thickness was measured immediately after leaf removal with a digital micrometer (Mitutoyo, Kawasaki, Japan) at a point mid-way along the length of the leaf and mid-way between the median and leaf edge. Leaf area was measured in the laboratory using scans and the software MatLab® (MathWorks Corp., Natick, MA, USA) for image processing.
Leaves were then dried at 65 °C to constant weight. The dry weight was used in conjunction with the area measurements to calculate specific leaf area (SLA, m² kg-1) using the following formula (eqn. 2):
where LA and LM are respectively the area and the dry mass of 10 sampled leaves. The leaf density (LD, kg m-3) was calculated using SLA and LT ([41]) as follows (eqn. 3):
Climate data
We used data from the meteorological stations (⇒ http://www.bom.gov.au/) at each site where we collected the seeds of eucalyptus species in Australia and Indonesia. The following 18 climatic variables were considered (Tab. S1 in Supplementary material): 1- average annual rainfall (AAR); 2- maximum annual rainfall (MAR); 3- minimum annual rainfall (mAR); 4- maximum monthly average rainfall (MMAR); 5- minimum monthly average rainfall (mMAR); 6- number of months of rainfall less than 50 mm (NR50); 7- average annual temperature (AAT); 8- maximum monthly temperature (MMT); 9- minimum average temperature (mMT); 10- maximum monthly average temperature (MMAT); 11- minimum monthly average temperature (mMAT); 12- number of days below 40 °C (ND40); 13- number of freezing days (NFD); 14- annual potential evapotranspiration (PE); 15- annual solar radiation (SR); 16- altitude (A); 17- longitude (Long); 18- latitude (Lat). Data on the same 18 climatic variables were also collected at the planting site (Pointe-Noire).
Statistical analysis
The following linear mixed model was used to perform ANOVA for survival rate (at the plot level), functional traits and growth (at tree level - eqn. 4):
where y is the vector of the response variable; µ is the overall mean; X and Z are the design matrix connecting the fixed and random effects to the data, respectively; b is a vector of fixed effects due to the blocks; sb ~ N(0, σ²sbId) is a vector of the random subgenus effect; σ²sb is the variance related to the subgenus effect; Id is the identity matrix; sp ~ N(0, σ²spId) is a vector of the random species effect; σ²sp is the variance related to the species effect; ε ~N(0, σ²εId) is a vector of the residual effect. Before performing ANOVA on survival rate, we calculated an angular arc sinus transformation (eqn. 5)
where p′ is the transformed value of survival rate and p is the original value of survival rate for each plot.
To detect covariations among functional traits, we used pairwise Pearson’s multiple correlation tests to analyse bivariate and multivariate relationships. To analyse the multivariate relationships, a principal component analysis (PCA) was performed in order to identify the similarities between the climatic characteristics of the area of origin of the species and the area where the species were introduced. At provenances level, functional traits were estimated from the tree measurements at 15 and 54 months (Tab. S2 in Supplementary material). The k-means partitioning method was performed to assign observations to clusters relating to growth and functional traits at the age of 54 months.
All statistical analyses were performed using the open-source software R v. 4.0.3.
Results
Variation of functional traits between species
Survival rate
The survival rate was significantly different between subgenera at 15 (P = 0.0003) and 54 months (P<0.001). Likewise, a similar effect was observed among species at 15 months (P<0.001) and at 54 months (P<0.001). At both ages, the subgenera Blakella and Idiogenes had the highest survival rates of 91% and 87%, respectively, whereas the subgenera Eucalyptus and Eudesmia had the lowest survival rates of 35% and 13%, respectively (Fig. 2). The species E. tetrodonta (17%), E. peltata (12%) and E. pilularis (0%) had the lowest survival rates at 54 months, while E. tereticornis (96%) and E. tesselaris (97%) had the highest survival rates at the same age (Fig. 2). The survival rate of E. robusta and E. alba_Australia remained the same between 15 and 54 months. That of species like E. tereticornis, E. alba_Timor, E. urophylla, E. maculata, E. citriodora, E. exserta, E. resinifera and E. pellita did not change considerably, while for E. pilularis, E. acmenoides, E. peltata, E. tetrodonta, E. torelliana and E. apodophylla, the change of survival rate over time is very marked (Fig. 3).
Fig. 2 - Mean values of survival rate by subgenera and species/provenances. Different letters above the bars indicate significant differences (p<0.05) between group means after Tukey’s test.
Fig. 3 - Difference in survival rate (%) of eucalyptus species/provenances between 54 and 15 months after tree establishment.
Growth and leaf traits
Growth traits increased according to ages from 15 months to 54 months. The ANOVA results showed significant differences between species of the same subgenus and between subgenera for all traits (Tab. 2). Leaf thickness (LT) remained almost stable, while specific leaf area (SLA) increased and leaf density (LD) decreased from 15 to 54 months. The subgenera Symphyomyrtus, Idiogenes, Eucalyptus and Corymbia showed greater variations in LT, whereas their SLA values remained low. In contrast, the subgenera Minutifructus, Eudesmia, Blakella and Alveolata had high SLA and low LT values. The Flores provenance of E. urophylla showed one of the best growth rates and a high SLA value.
Tab. 2 - Descriptive statistics and Anova results of variables studied at 15 and 54 months. (SR): survival rate (%); (HT): tree height (m); (SLA): specific leaf area (m2 kg-1); (LT): leaf thickness (mm); (LD): leaf density (kg m-3); (SD): standard deviation; (Max): maximum; (Min): minimum. 15 and 54 indicate the age tree (in months).
Traits | Unit | Mean | SD | Max | Min | (Pr > F) | ||
---|---|---|---|---|---|---|---|---|
Block | Sub-genus | Species/ Provenance |
||||||
SR15 | % | 68.93 | 21.07 | 100.00 | 17.85 | 0.273 | 0.0003 | <0.0001 |
SR54 | % | 61.24 | 23.40 | 91.83 | 0.00 | 0.177 | <0.0001 | <0.0001 |
HT15 | m | 2.02 | 0.87 | 5.55 | 0.55 | 0.195 | 0.023 | 0.003 |
HT54 | m | 7.99 | 3.26 | 18.60 | 1.50 | <0.0001 | <0.0001 | <0.0001 |
CC15 | cm | 7.27 | 3.87 | 26.12 | 0.94 | 0.188 | 0.068 | 0.003 |
CC54 | cm | 29.98 | 12.84 | 72.80 | 1.50 | <0.0001 | <0.0001 | <0.0001 |
SLA15 | m2 kg-1 | 80.43 | 16.79 | 148.84 | 45.76 | 0.392 | <0.0001 | <0.0001 |
SLA54 | m2 kg-1 | 259.73 | 102.00 | 529.46 | 38.36 | <0.0001 | <0.0001 | <0.0001 |
LT15 | mm | 0.28 | 0.05 | 0.46 | 0.14 | 0.0002 | <0.0001 | <0.0001 |
LT54 | mm | 0.27 | 0.05 | 0.42 | 0.16 | <0.0001 | <0.0001 | <0.0001 |
LD15 | kg m-3 | 0.46 | 0.06 | 0.60 | 0.32 | <0.0001 | <0.0001 | <0.0001 |
LD54 | kg m-3 | 0.18 | 0.11 | 1.15 | 0.08 | <0.0001 | <0.0001 | <0.0001 |
Correlations between traits
The juvenile-adult correlations (Fig. 4) indicated close ranking at different ages for LT and height. In contrast, the LD ranking changed completely from 15 to 54 months. There was a strong and positive correlation between height and collar circumference at 15 months (Pearson’s r = 0.79) and 54 months (Pearson’s r = 0.84), and a moderate and positive correlation between growth and survival (Pearson’s r = 0.41 to 0.57). Between 15 and 54 months, the correlation of individual SLA values was moderate (Pearson’s r = 0.49). Further, there was a weak correlation between growth and SLA (Pearson’s r = 0.10 to 0.28). We found a negative correlation between SLA and LD (Pearson’s r = -0.59 to -0.50), as well as between SLA and LT (Pearson’s r = -0.67 to -0.42), and also between LD and LT (Pearson’s r = -0.50 to -0.17) from 15 to 54 months.
Fig. 4 - Correlations between juvenile and adult traits. (HT): total tree height (m); (CC): collar circumference (cm); (LT): leaf thickness (mm); (SLA): specific leaf area (m2 kg-1); (LD): leaf density (kg m-3); 15 and 54 indicate the age of tree (in months).
PCA and clustering analysis
The PCA results indicated that the main components (two first axes) account for 66% of the total variation (Fig. 5). The two main components were associated with temperature and solar radiation (41.16%) and rainfall (24.84%), respectively. The results showed that species like E. tereticornis (from Halenvale), E. nesophilla (Coboutg peninsula), E. tetrodonta (South Est Maningrida), E. polycarpa (Est Gue Goyder river), E. brassiana (Cape York peninsula) and E. alba (Timor) were found in areas where the climatic conditions are similar to the Congolese coastal savannas (Fig. 5). On the contrary, E. punctata, E. maculata, E. acmenoides and E. resinifera were originated from areas whose climatic conditions differed from the area of introduction.
Fig. 5 - Principal component analysis biplot. Numbers refer to the different eucalypt species/provenances. (1): E. microcorys; (2): E. tesselaris; (3): E. citriodora; (4): E. maculata; (5): E. nesophila; (6): E. peltata; (7): E. polycarpa; (8): E. torelliana; (9): E .acmenoides; (10): E. phaeotricha; (11): E. pilularis; (12): E. umbra; (13): E. tetrodonta; (14): E. cloeziana; (15): E. raveretiana; (16): E. alba_Australia; (17): E. alba_Timor; (18): E. apodophylla; (19): E .bigalerita; (20): E. brassiana; (21): E. exserta; (22): E. grandis; (23): E. paniculata; (24): E. pellita; (25): E. propinqua; (26): E. punctata; (27): E. resinifera; (28): E. robusta; (29): E. tereticornis; (30): E. urophylla_Mte Lewotobi; (31): E. urophylla_Flores; (32): E. urophylla_Timor; (33): Pointe-noire (planting site).
The results of the cluster analysis suggest that the 32 provenances analyzed can be subdivided into four groups (Fig. 6). The first cluster included E. pilularis and E. peltata, with the lowest growth rate and the smallest SLA. At the end of the trial, no individuals representing E. pilularis were present. The second cluster contained species with a wide variety of responses in terms of their growth strategy. Some species had a very high growth rate and a large SLA, for example, E. cloeziana, E. urophylla_Flores and E. alba_Timor. This cluster also included species with medium growth rates and low SLA, like E. robusta and E. brassiana, and species with slower growth and higher SLA, like E. toreliana. Altogether, cluster 2 can be divided into two others clusters: (i) C2a including provenances 14, 31, 22, 24, 30, 4, 3 (Fig. 6) having a good growth and survival, as well as medium-high SLA; and (ii) C2b including provenances 21, 8, 20, 7, 27, 29, 23, 28, 10, 32, 5, 5, 17 (Fig. 6) with various characteristics. The species in cluster 3 had large SLA values but slow growth. The last cluster includes the species with slow growth and small SLA, like E. tesselaris.
Fig. 6 - Cluster dendrogram of species/provenances. Cluster 1: (11) E. pilularis and (6) E. peltata; Cluster 2: (14) E. cloeziana, (31) E. urophylla_Flores, (22) E. grandis, (24) E. pellita, (30) E. urophylla_Mte Lewotobi, (4) E. maculata, (3) E. citriodora, (21) E. exserta, (8) E. torelliana, (20) E. brassiana, (7) E. polycarpa, (27) E. resinifera, (29) E. tereticornis, (23) E. paniculata, (28) E. robusta, (10) E. phaeotricha, (32) E. urophylla_Timor and (5) E. nesophila, (17) E. alba_Timor; Cluster 3: (19) E. bigalerita, (18) E. apodophylla, (26) E. punctata and (16) E. alba_Australia; Cluster 4: (9) E. acmenoides, (1) E. microcorys, (15) E. raveretiana, (13) E. tetrodonta, (25) E. propinqua, (2) E. tesselaris and (12) E. umbra.
Discussion
Variability of functional traits among species
Survival is the first fundamental criterion to assess adaptation ([14]). Plants compete for light, water, nutrients, air and space to survive. In our study, ranking species according to their survival rate changed significantly from 15 months to 54 months. The 100% mortality of E. pilularis trees after 54 months from establishment reflects their very poor adaptation to the local conditions in Pointe-Noire. This result corroborated those obtained by Brezard ([4]) in the same region. It is worth noting that the climate of the original area for E. pilularis is very different from that at the plantation site. However, this result needs to be confirmed as only 9 plants of this species were initially included in the experiment (Tab. S2 in Supplementary material).
Species with a low survival rate but good growth, like E. citriodora, were not necessarily poorly adapted in the study area, as several factors may be involved, such as plantation density leading to competition between species. Indeed, intense competition between adjacent plots could have led to differences between fast- and slow-growing species, with the suppression of the latter species ([17]).
Our study revealed a difference of survival rate and growth both among species of the same subgenus and among subgenera. One of the characteristics in which species of the same or different habitats vary is their growth potential. In a common environment, trade-offs between functional traits are the basis for the observation of different growth strategies and finally different strategies of adaptation ([37]). Adaptations can be divided into two general categories: functional adaptations, which help an organism to survive in its environment; and growth and reproductive adaptations, which help an organism to grow and reproduce successfully. Both are required to ensure the “full adaptation”.
Correlation
All growth traits were positively correlated between 15 and 54 months since tree establishment. The stability of leaf thickness and the decrease in leaf density led to an increase in SLA between 15 and 54 months. This result can be explained by the fact that water was a limiting factor at 15 months, while at 54 months this was less limiting because of the root development over a larger area and greater depth ([43]).
Our results showed negative and significant correlations between SLA, LT and LD. These results corroborated those of Vile et al. ([47]) and Niinemets ([31]). Low values for SLA due to thicker and/or denser leaves contribute to leaf life span, nutrient retention and protection against desiccation ([1]). From the correlations between height and functional traits, we were able to identify the different species’ strategies to acquire and use the available environmental resources.
Species’ adaptive strategies
Functional traits play a critical role in the adaptation process ([3]). Understanding local adaptive species traits according to climatic factors is key when it comes to developing plantation strategies ([49]). It was firstly assumed that species from areas with climatic conditions similar to those occurring in Pointe-Noire would be better suited than those from areas with a different climate. This hypothesis was only verified for one species, E. tereticornis, which achieved a good balance between resource acquisition and use. However, some species from areas with very different climatic characteristics compared to those at Pointe-Noire appear to be more suitable, as in the case of most species in cluster 2. These species thrive in humid, subhumid, tropical or subtropical regions with rainfall of 1100 to 1500 mm and a dry season lasting 1 to 5 months. A similar rainfall pattern is found in the Congo. The eucalypts’ adaptations allow many species to thrive under often difficult conditions, with poor soils and highly variable rainfall. Consequently, a small number of eucalypt species are grown very successfully in plantations ([38]). Genetic diversity is valuable for species survival and adaptation. Some individuals carry genes that allow them to respond to environmental constraints and enhance their adaptive capacity more successfully than others ([39]). Genetic variations can, therefore, modulate the local effects of environmental change ([34]). Local adaptation can be constrained by natural selection and gene flow ([21], [26]). Previous studies revealed that local adaptation among different populations (and provenances) of E. camaldulensis could be due to variation in adaptive phenotypes, which correspond to the local environment. These include morphological traits (growth form, leaf thickness, stomatal density and phenology - [18]), growth rate ([33]), wood properties ([10]), physiological responses ([30]), and drought tolerance ([27]). The adaptive clines inferred from phenotypic variation were supported by evidence of genetic adaptation in coding genes ([44]).
Leaf traits do not vary randomly, but depend on trade-offs from investing carbon in leaves ([48]). Specific leaf area is an important functional trait because it is an indicator of relative growth rate, stress tolerance and leaf life span. It is generally assumed that intraspecific SLA variation is largely due to environmentally induced phenotypic plasticity ([28]). However, genetic effects should also be considered such as local adaptation or genetic drift ([40]). At the interspecific level, functional traits can explain community composition and structure ([25], [7]). They can also be used to classify plant species’ strategies according to Grime ([15]) or to explain species occurrence and distribution ([6]). Our results suggest that population of different eucalypt species could be able to survive under different climate conditions by adjusting their SLA. In the long term, phenotypic plasticity may allow populations to adapt genetically to environmental changes via genetic assimilation ([36]).
The results of cluster analysis showed that the first group includes two species which are totally unsuited to the local conditions in Pointe-Noire. Conversely, species of the second cluster can adapt to the local conditions in Pointe-Noire because of their greater plasticity. Some species can rapidly exploit the environmental resources and allocate them to development, while others show a slow resource acquisition but a fairly efficient use. We found that species in the third cluster had strategies allowing them to acquire resources rapidly with a slow return in terms of investment on leaf economic spectrum ([48]). According to Lambers & Poorter ([24]), these species are specialised in acquiring large quantities of resources (water, light, carbon), while investing very little in growth. Finally, the fourth cluster includes species whose strategy involves a slow acquisition and use of resources, thus resulting in their poor growth due to the reduced photosynthetic capacity.
Conclusion
Our study provides important advances for the understanding the adaptive strategies of Eucalyptus spp. in the experimental conditions at Pointe-Noire, Republic of the Congo. Based on leaf traits and growth, four major groups of species were identified: (i) species with a large SLA and good growth; (ii) species with a small SLA and poor growth; (iii) efficient species with small SLA and very good growth; and (iv) species with large SLA and poor growth. The species included in the groups (ii) and (iv) are the least suitable to the environmental conditions at the trial site; those in the group (iv) maximise the capture of resources, but have poor growth.
Following this study, it would be relevant to determine the differential expression of genes involved in growth in different provenances and contrasting environments. Further study should focus on species’ different strategies for acquiring and using resources in their area of origin and the area of introduction. A combined phenotypic and genomic approach ([42]) should be included in future studies of plant adaptation to local conditions in the Republic of the Congo.
Acknowledgements
We are grateful to Pacifique Ntadi and the CRDPI technical team for field harvesting. We would like to thank the Conservation Action Research Network (CARN) of the Congo Basin Forest Partnership (CBFP) for their help to support this study.
PhV and CGME conceived and designed the experiments; CBSVL and MPM performed the trials; CGME and GJLP carried out data analysis. CGME took the lead in writing the manuscript and all authors provided critical feedback.
References
Gscholar
CrossRef | Gscholar
Gscholar
Gscholar
CrossRef | Gscholar
CrossRef | Gscholar
Online | Gscholar
Gscholar
Gscholar
CrossRef | Gscholar
Online | Gscholar
CrossRef | Gscholar
CrossRef | Gscholar
Authors’ Info
Authors’ Affiliation
Ecole Nationale Supérieure d’Agronomie et de Foresterie (ENSAF), Marien Ngouabi University, Brazzaville (Republic of the Congo)
Centre de Recherche sur la Durabilité et la Productivité des Plantations Industrielles - CRDPI, Pointe-Noire (Republic of the Congo)
Castela Bath Shéba Vitel Loubassou
Institut National de Recherche Forestière - IRF, Brazzaville (Republic of the Congo)
Programme National d’Afforestation et de Reboisement - PRONAR, Brazzaville (Republic of the Congo)
Laboratoire de Biodiversité, Gestion des Ecosystèmes et de l’Environnement - LBGE, Faculté des Sciences et Techniques, Université Marien Ngouabi, BP 69 Brazzaville (Republic of the Congo)
Centre de Coopération International de Recherche en Agronomie pour le Développement - CIRAD, UMR AGAP, Amélioration Génétique et Adaptation des Plantes Tropicales et Méditerranéennes, Montpellier (France)
Corresponding author
Paper Info
Citation
Makouanzi Ekomono CG, Loubassou CBSV, Mbama MP, Loubota Panzou GJ, Vigneron P (2021). Adaptability and interspecific variability in growth and leaf traits of eucalypt. iForest 14: 560-568. - doi: 10.3832/ifor3660-014
Academic Editor
Rossella Guerrieri
Paper history
Received: Sep 26, 2020
Accepted: Oct 10, 2021
First online: Dec 09, 2021
Publication Date: Dec 31, 2021
Publication Time: 2.00 months
Copyright Information
© SISEF - The Italian Society of Silviculture and Forest Ecology 2021
Open Access
This article is distributed under the terms of the Creative Commons Attribution-Non Commercial 4.0 International (https://creativecommons.org/licenses/by-nc/4.0/), which permits unrestricted use, distribution, and reproduction in any medium, provided you give appropriate credit to the original author(s) and the source, provide a link to the Creative Commons license, and indicate if changes were made.
Web Metrics
Breakdown by View Type
Article Usage
Total Article Views: 29778
(from publication date up to now)
Breakdown by View Type
HTML Page Views: 25831
Abstract Page Views: 1874
PDF Downloads: 1602
Citation/Reference Downloads: 5
XML Downloads: 466
Web Metrics
Days since publication: 1311
Overall contacts: 29778
Avg. contacts per week: 159.00
Article Citations
Article citations are based on data periodically collected from the Clarivate Web of Science web site
(last update: Mar 2025)
(No citations were found up to date. Please come back later)
Publication Metrics
by Dimensions ©
Articles citing this article
List of the papers citing this article based on CrossRef Cited-by.
Related Contents
iForest Similar Articles
Research Articles
Interaction between planting spacing and wood properties of Eucalyptus clones grown in short rotation
vol. 14, pp. 12-17 (online: 02 January 2021)
Research Articles
Variation of wood and bark density and production in coppiced Eucalyptus globulus trees in a second rotation
vol. 9, pp. 270-275 (online: 08 September 2015)
Research Articles
Impact of rotation length of Eucalyptus globulus Labill. on wood production, kraft pulping, and forest value
vol. 15, pp. 433-443 (online: 20 October 2022)
Research Articles
Contrasted growth response of hybrid larch (Larix × marschlinsii), jack pine (Pinus banksiana) and white spruce (Picea glauca) to wood ash application in northwestern Quebec, Canada
vol. 14, pp. 155-165 (online: 06 April 2021)
Research Articles
Potential for utilization of wood ash on coastal arenosols with limited buffer capacity in KwaZulu-Natal and its effect on eucalypt stand nutrition and growth
vol. 10, pp. 180-188 (online: 19 November 2016)
Research Articles
Preliminary study on genetic variation of growth traits and wood properties and superior clones selection of Populus ussuriensis Kom.
vol. 12, pp. 459-466 (online: 29 September 2019)
Research Articles
Validation of models using near-infrared spectroscopy to estimate basic density and chemical composition of Eucalyptus wood
vol. 17, pp. 338-345 (online: 03 November 2024)
Research Articles
NIR-based models for estimating selected physical and chemical wood properties from fast-growing plantations
vol. 15, pp. 372-380 (online: 05 October 2022)
Research Articles
Physical, chemical and mechanical properties of Pinus sylvestris wood at five sites in Portugal
vol. 10, pp. 669-679 (online: 11 July 2017)
Research Articles
Effect of stand density on longitudinal variation of wood and bark growth in fast-growing Eucalyptus plantations
vol. 12, pp. 527-532 (online: 12 December 2019)
iForest Database Search
Search By Author
Search By Keyword
Google Scholar Search
Citing Articles
Search By Author
Search By Keywords
PubMed Search
Search By Author
Search By Keyword