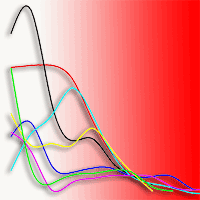
Factors affecting the quantity and type of tree-related microhabitats in Mediterranean mountain forests of high nature value
iForest - Biogeosciences and Forestry, Volume 14, Issue 3, Pages 250-259 (2021)
doi: https://doi.org/10.3832/ifor3568-014
Published: May 21, 2021 - Copyright © 2021 SISEF
Research Articles
Abstract
Tree-related microhabitats (hereafter TreMs) are structures occurring on trees, such as rot holes, cavities, large nests, mould, fruiting bodies and mycelia of decomposer fungi. TreMs have been widely recognized as important substrates and structures useful for biodiversity conservation in forest ecosystems, and they can be used as indicators for describing and monitoring forest naturalness. However, most studies on the occurrence of TreMs have been mainly done in forest ecosystems of Central Europe, while less research has been conducted in Mediterranean mountain forests. In this study, we investigated the diversity and abundance of 23 types of TreMs on living trees and on deadwood in seven Mediterranean mountains unmanaged forests located in the Apennines (Italy). The abundance of TreMs was evaluated by counting the number of TreMs per tree, while the diversity of TreMs was evaluated by means of the Shannon-Wiener index. We focused on the relationships between diversity and abundance of TreMs, and tree size (e.g., diameter, height, volume), and the time since the last harvest. Among the investigated stands, 2612 living trees, 457 standing dead trees and snags, and 1247 lying deadwood pieces were analysed. For living trees, a generalized linear mixed model was applied to test the effect of several variables on the abundance of TreMs per tree. Diameter at breast height (DBH) of tree stems influenced the abundance and diversity of TreMs. The time since the last harvest also significantly affected the probability that TreMs could be formed in a long-term perspective. The interaction of the predictors “DBH2” and “Years since the last harvest” generated a better model than the one in which the two variables were kept separate. Indeed, these two factors together would better represent the transition of a previously managed forest to a more natural state over time. This study might provide useful information to land managers committed to forestry practices towards sustainable management and biodiversity conservation, especially referring to survey and inventory of forests of high nature value.
Keywords
Biodiversity Indicators, Forest Structure, Old-growth Forests, Tree Microhabitats, Woody Debris
Introduction
Forest ecosystems with a high degree of naturalness tend to have more complex horizontal and vertical structures, which may support more species by increasing the diversity and abundance of ecological niches at small scale. These forest stands may serve as suitable reference for managing forest in a sustainable way ([34]).
In Europe, many studies have been conducted to describe old-growth forests and their level of naturalness, focusing on their structure and composition ([5]), dynamics and natural regeneration ([26]), and deadwood characteristics ([41]). For example, the heterogeneity in tree heights and high values of living tree volume or stand basal area have been considered features useful to characterize and increase forest naturalness ([34]). Moreover, large amounts of standing and downed dead trees, but also woody debris on the forest floor at all stages of decay, have been identified as key factors for the conservation of biodiversity in forests ([7]). Most of the research focused on old-growth forests of the Mediterranean mountains has given attention to different forest structural features, such as the vertical and horizontal heterogeneity of the stand, gap dynamics, and deadwood amounts ([22], [20]), considering also the state of conservation of habitats and veteran trees ([38]).
Structures such as rot holes, cavities, large nests, mould, fruiting bodies, and mycelia of decomposer fungi occurring on trees have been proposed as indicators for monitoring forest biodiversity and ecosystem integrity ([3], [27]). These structures are commonly named tree-related microhabitats (hereafter TreMs). Several studies have described TreMs profile in old-growth forests ([11], [28]), as well as the dynamics of TreMs in unmanaged temperate mountain mixed forests ([15]). In the original definition of Larrieu et al. ([17]), TreMs are “distinct, well-delineated structures occurring on living or standing dead trees that constitute a particular and essential substrate or life site for species or communities during at least a part of their life cycle to develop, feed, shelter or breed”. This definition can be also applied to TreMs occurring on lying deadwood, which hosts many saproxilic insects, fungi and lichens ([1], [31]).
Diversified stand structure, large deadwood amounts and the occurrence of TreMs have been linked to habitat heterogeneity and species richness, including saproxylic organisms ([1], [33]). Because TreMs are important substrates and structures for living organisms, they have received increasing attention in sustainable forestry, mainly for identifying habitat trees ([16]). TreMs, less explored in managed forests than in old-growth forests, can be used as indicators of biodiversity in forest monitoring schemes ([27]). Many taxa, including lichens, bryophytes, fungi, insects, arthropods, small mammals and birds, rely on TreMs traits, positively affecting forest functioning ([41]). Therefore, insights on the factors influencing the abundance of TreMs may allow the implementation of scientifically-sound and data-driven forest monitoring and management practices; in particular, they can be useful to identify ecologically valuable habitats for preserving forest biodiversity.
The development of TreMs can be strongly influenced by silvicultural practices and structural features ([14], [37], [10]). Several studies have explored the link between TreMs, management approaches and stem diameters ([45], [25], [43], [29]), though investigation has generally focused on living or standing dead trees ([17]). However, factors affecting numbers and types of TreMs have not been completely investigated for the different geographical regions ([28]). This lack of knowledge is particularly evident in Mediterranean environments. Recent studies have focused on beech-fir forests in Central and Western Europe ([44], [43], [13], [14], [15]) and only a few on Mediterranean broadleaved forests ([37], [32]).
In this study, in seven unmanaged forests (without any silvicultural activities for decades or centuries) located in the Apennines (Italy), we investigated the diversity and abundance of 23 types of TreMs on the following elements: living trees, standing dead trees, snags, dead downed trees, coarse woody debris and stumps. The abundance of TreMs was obtained by counting the number of TreMs per tree, while the diversity of the several types of TreMs was evaluated through the use of diversity indices, such as the Shannon-Wiener diversity Index. We focused on the relationships between TreMs (abundance and diversity), tree size (e.g., diameter, height, volume) and time since the last harvest. We aimed at identifying which tree characteristics would support higher abundance and diversity of TreMs, providing a valuable indicator for monitoring forest biodiversity. For living trees, a generalized linear mixed model was applied to test the effect of several variables on the abundance of TreMs at the tree scale. We hypothesized a positive effect of tree stem diameters and time since the last harvest on the diversity and abundance of TreMs per tree. The magnitude of these relationships could be mainly driven both by the abandonment of silvicultural practices and by the size of individual trees (diameter, height, volume), and their interactions. Therefore, we analysed the combined effects of the time since the last harvest and the size of trees on the abundance of different TreMs.
Materials and methods
Study sites
The study was conducted in seven unmanaged forests (Tab. 1) located in the Italian Peninsula (central and southern Apennines) and characterized by different structural characteristics. The study areas are all part of natural reserves, Natura 2000 network sites or national parks, where natural development processes are the main drivers of the forest stand evolution (Tab. 1).
Tab. 1 - Main characteristics of the investigated forests listed for the time since the last harvest.
Characteristics | Site | ||||||
---|---|---|---|---|---|---|---|
Abeti Soprani | Collemeluccio | Montedimezzo | Area Pavari |
Bosco Aschiero | Fonte Novello | Val Cervara |
|
Location | EU Natura 2000 Network (Molise) | MaB Unesco Reserve (Molise) | Gargano National Park (Puglia) | Gran Sasso e Monti della Laga National Park (Abruzzo) | Abruzzo, Lazio e Molise National Park |
||
Latitude N | 41° 86’ | 41° 72’ | 41° 75’ | 41° 82’ | 42° 50’ | 42°50’ | 41° 49’ |
Longitude E | 14° 30’ | 14° 35’ | 14° 21’ | 15° 99’ | 13° 58’ | 13°50’ | 13° 43’ |
Forest area (ha) | 232 | 374 | 291 | 5.4 | 5.2 | 13.4 | 300 |
Altitudinal range (m a.s.l.) | 1250-1450 | 900-1000 | 950-1050 | 720-800 | 1580-1640 | 1320-1360 | 1730-1830 |
Mean annual temperature (°C) | 8.4 | 9.2 | 8.6 | 11.6 | 10 | 10 | 7.2 |
Mean annual precipitation (mm) | 1124 | 960 | 1022 | 1041 | 1246 | 1071 | 1211 |
Main tree species | silver fir | fir-beech | oak-beech | beech | beech | beech | beech |
Mean age of living trees (yrs) | 131 | 121 | 139 | 164 | 170 | 152 | 262 |
Time since the last harvest (yrs) | 31 | 51 | 56 | 58 | 310 | 312 | >500 |
The elevation of the study sites ranges from 700 to 1800 m a.s.l. These forests are currently unmanaged, and their structural characteristics refer to high forest with diversified horizontal and vertical stand structures. They refer to pure beech forests for “Bosco Aschiero”, “Fonte Novello”, “Val Cervara” and “Area Pavari”, whereas “Montedimezzo” is a deciduous oak-beech mixed forest; “Abeti Soprani” is a silver fir-beech mixed forest, while the main species in “Collemeluccio” are silver fir and deciduous oaks. The last two study sites are both relict stands, where silver fir still occurs, surviving from the last glacial period. Their structures and tree species mixture are similar to those of native forests, throughout the Apennines, nowadays present only in small old-growth forest patches along the Italian Peninsula. This association becomes more stable in less fertile sites, where beech is less competitive.
The beech forests of “Bosco Aschiero” and “Fonte Novello” are within the “Gran Sasso e Monti della Laga” National Park. The “Fonte Novello” forest has not been managed in the last centuries, due to legal debates concerning the position of the administrative boundaries between municipalities. Whereas, “Bosco Aschiero” has escaped from logging practices, developing old-growth structural characteristics, due to the rocky and steep terrain. The beech high forest of “Val Cervara” is included in the “Abruzzo, Lazio e Molise” National Park. This forest has been included within the UNESCO Heritage sites, as one of the “Ancient and primeval beech forests of the Carpathians and other regions of Europe”. It has been unmanaged for centuries, due to difficult access, and because of its protective role against avalanches.
Furthermore, the “Abeti Soprani” forest is no longer managed and has not been logged for more than three decades, due to the low interest for conifer timber in the local context. “Collemeluccio” and “Montedimezzo” forests are part of the “Collemeluccio-Montedimezzo Alto Molise” MaB Unesco Reserve and have been unmanaged for the last 50-60 years. Finally, the beech high forest of “Area Pavari”, located in the “Gargano” National Park, is part of an old-growth forest research network established in 1952; it has been unmanaged since 1954. For further details regarding the study sites, see Lombardi et al. ([20]).
Survey of living trees and deadwood components
At each site, a 1-ha wide square plot was established and clearly delimited. One hectare was chosen because old-growth forest traits are usually characterized by high horizontal heterogeneity and a single large plot may better capture the structural diversity of a forest than several small plots ([21]).
The main forest stand attributes were recorded, measuring for each species the diameter at breast height (DBH ≥ 10 cm) and the total height for all the living trees and standing dead trees (SDT); the total length was measured for dead downed trees (DDT). In addition, the minimum and maximum diameter and length were recorded for snags (minimum diameter ≥ 5 cm), coarse woody debris (CWD - minimum diameter ≥ 5 cm and minimum length ≥ 1 m), and stumps (minimum diameter ≥ 5 cm). SDT and DDT were characterized by the presence of crown (dead branches and twigs), while snags were stems without crown (short broken bole, little bark remaining, soft wood); CWD refers to the remains of large branches or stem portions or large woody debris lying on the forest floor. A threshold height of 1.3 m was used to distinguish stumps (less than 1.3 m) from snags (higher than 1.3 m). Living trees, SDT, snags and stumps were measured when more than half the base of their trunk occurred within the plot. CWD and DDT were measured when more than half the base of its thicker end occurred within the plot (more details can be found in [20]).
The volume of living trees, SDT and DDT was calculated by double-entry volume equations ([42]), while the volume of snags, CWD and stumps was computed as follows (eqn. 1):
where V is the volume (m3), h is the height or length (m), D is the maximum diameter (m) and d is the minimum diameter (m).
Decay stage of each deadwood piece was classified visually, using the five-grade scale proposed by Hunter ([9]), in which the morphological characteristics of deadwood are assessed. Further details on the applied sampling protocol are available in Lombardi et al. ([20]).
Assessment of microhabitats
In this survey, we referred to a set of 23 types of TreMs, according to Winter & Möller ([45]), with minor changes (Tab. 2). Although new survey schemes have been made available ([17]), which will enable standardized TreMs recording in future forest inventories, local monitoring schemes still refer to the classification of Winter & Möller ([45]).
Tab. 2 - Description of the 23 types of TreMs censused in the study sites (modified from [45]).
ID TreMs | TreMs description |
---|---|
M1 | Occurrence of fruiting bodies of Fomes fomentarius |
M2 | Occurrence of fruiting bodies of Fomitopsis pinicola |
M3 | Occurrence of other fungal infection |
M4 | Crown broken <50% |
M5 | Several main branches are broken: >50% of the crown broken |
M6 | Broken fork: complete fracture of one of the two forking branches |
M7 | Broken stem: the crown is totally absent and very small living twigs have remained |
M8 | Substitute or secondary crown |
M9 | Lightning scar at least 3 m long and reaching the sapwood |
M10 | Crack: cleft into the sapwood >50cm long along the stem and at least 2 cm deep |
M11 | Splintered stem: the split-up results in numerous scales of wood >50 cm long |
M12 | Cavities with >5 cm aperture |
M13 | Cavity string: at least three woodpecker cavities |
M14 | Deep stem cavities: a tubular cavity with little or without mould |
M15 | Cavities with mould of at least 8000 cm3 |
M16 | Mould pockets: space between loose bark and the sapwood |
M17 | Bark pockets: same structure as M16, but without mould |
M18 | Canker: proliferation of cell growth at least 10 cm in diameter |
M19 | Bark loss: patches with bark loss of at least 5 cm caused by natural falling of trees |
M20 | Uprooted stump, with a minimum height of 1.20 m of the vertical root plate |
M21 | System of gallery of Scolytidae insects |
M22 | Saproxylic insect holes |
M23 | Water filled rot hole on stump |
In each study site, we recorded the TreM type and the abundance of TreMs occurring on each living tree and deadwood component. We carefully examined the entire living and standing dead tree, but also all the horizontal deadwood elements occurring on the forest floor. In total, 2612 living trees, 457 SDT and snags, and 1247 CWD were individually and exhaustively inspected. Some TreMs were recorded only on living trees, such as those on branches and broken crowns (M4-M8), and others on stems (M9-M11 and M14-M19). M5 was visually estimated with binoculars, while M16 and M17 were measured in their length and deepness in the trunk, in order to distinguish them from each other. Finally, M21 and M22 were counted if their extension was higher than 0.5 m2. All the censused TreMs were linked to the forest components on which they were observed (living trees, SDT, snags, DDT, CWD, stumps).
Data analysis
In each site, we recorded the diversity of each type of TreMs (M1, M2, M3 etc) and the abundance of TreMs on each living trees and on five deadwood components.
In our analyses, we considered two variables: (1) the variable “abundance of TreMs per tree”, considered as the sum of the TreMs counted on each living tree and/or deadwood component; (2) the variable “diversity of TreMs”, based on the number of the different types of TreMs (Tab. 2) occurring on each living tree and/or deadwood component. The TreMs diversity was evaluated through the Shannon diversity index ([40]).
In order to verify the differences among the Shannon diversity index values, between components and among sites, the confidence intervals were calculated through a bootstrap procedure ([4]). Furthermore, to assess the significance, the PERMANOVA statistical test (Permutational Analysis of Variance) was applied: it considers the values of similarities without any assumption about the data distribution ([24]). Then, the PERMANOVA test verifies the simultaneous responses of one or more variables for one or more factors in an ANOVA experimental model, calculating the p-values through permutations ([23]).
Differences between the considered components (living trees, SDT, DDT, snag, CWD and stump) in the number of TreMs per element were verified based on the whole dataset, using the Kruskal-Wallis test (“coin” package for the R programming language) and the pair-wise Conover test (“PMCMR” package for the R programming language). In all comparisons, the significance was associated to the critical value p≤0.05.
For living trees, we tested the effect of several independent variables, or their combination, on TreMs number per tree, using different models. The variables were the following: (1) time since the last harvest (YEAR); (2) stem diameter (DBH); (3) tree height (HT); (4) height of crown insertion point (HC); (5) stem volume (VOL), interaction between DBH2 and YEAR, expressed as DBH2 · YEAR. Models were run over the whole set of available independent variables (in total 2612 trees, whose 209 with at least one TreMs and 2403 with zero TreM), based on a linear mixed model, including both fixed and random effects. The independent variables were used as fixed-effect predictors. The site ID was added as random effect. The analysis considered as possible the incorporation of the random effects in the model, allowing the non-independence of observations ([47]). Since the “number of TreMs” referred to count data with an excess of zeros, we used a zero-inflated Poisson error distribution for applying the above cited models. This model was able to handle both the presence/absence of TreMs and their abundance (i.e., the number of TreMs per tree - [12]).
The following models were compared for testing the effect of the above-mentioned variables on the number of TreMs per tree:
- Model [1-5]: One-variable models (YEAR, DBH, HT, HC, VOL);
- Model [6]: Complete additive model (YEAR+DBH+HT+HC+VOL);
- Model [7]: Combined model (DBH2·YEAR).
We fitted these models based on the Akaike Information Criterion (AIC); for model [6], we used a stepwise procedure (in each step, a variable was considered for addition to or subtraction from the set of explanatory variables based on F-tests). This process allowed to simplify the models, then obtaining more efficient statistics. Results of the model were considered significant at a probability level of 5%. Among the compared models (based on the variables specified above), the model with the lowest Akaike Information Criterion (AIC) value was selected. In addition, for the selected model, VIF (variance inflation factor) was analysed to check for multicollinearity. For the GLMMs, the “glmmTMB” in the TMB package for the R programming language ([36]) was used.
Results
Forest features and deadwood amounts
The main forest attributes of the investigated stands are shown in Tab. 3.
Tab. 3 - Main structural and dendrometric features of the seven study sites. (BA): basal area; (Vlive): living tree volume; (Vdead): Deadwood volume.
Site | Trees (n ha-1) |
Mean DBH (cm) |
BA (m2 ha-1) |
Vlive (m3 ha-1) |
Vdead (m3 ha-1) |
Vdead / (Vlive+ Vdead) (%) |
---|---|---|---|---|---|---|
Abeti Soprani | 591 | 34.8 | 56.3 | 569.8 | 95.6 | 14.4 |
Collemeluccio | 570 | 32.1 | 46.1 | 557.8 | 17.4 | 3.0 |
Montedimezzo | 344 | 40.4 | 44.0 | 702.5 | 26.5 | 3.6 |
Area Pavari | 191 | 49.8 | 37.2 | 666.3 | 89.5 | 11.8 |
Bosco Aschiero | 277 | 48.4 | 51.1 | 584.1 | 21.3 | 3.5 |
Fonte Novello | 406 | 44.3 | 62.7 | 1070.3 | 88.9 | 7.7 |
Val Cervara | 233 | 37.0 | 25.1 | 363.6 | 143.0 | 28.2 |
Mean | 373 | 41.0 | 46.1 | 644.9 | 68.9 | 10.3 |
SD | 158 | 6.8 | 12.4 | 216.3 | 47.9 | 9.0 |
Structural characteristics differed among the studied stands, with a number of trees per hectare varying from 191 in Area Pavari to 591 in Abeti Soprani. Living tree volume ranged between 364 and 1070 m3 ha-1, with the lowest values recorded in the beech forest of Val Cervara and the highest in Fonte Novello. All the studied stands showed a multi-sized diameter distribution of living trees (Fig. 1), with the occurrence of a high number of tree diameter classes.
Fig. 1 - Number of trees per hectare in relation to the diameter classes (10 cm) in the seven study sites.
Although with varying trends, each study site displayed a decreasing number of living trees as the diameter classes increased. The highest number of trees with the lowest stem DBH was found in Collemeluccio, whereas Fonte Novello and Area Pavari had trees with the largest stem DBH. Trees with stem DBH larger than 80 cm were mainly observed in Bosco Aschiero and Val Cervara, while they were totally absent in Montedimezzo and Abeti Soprani. Deadwood volume showed a large variability among the study sites (Tab. 3), ranging between 17.4 m3 ha-1 in Collemeluccio and 143.0 m3 ha-1 in Val Cervara. Considering all the study sites, an average deadwood volume of 68.9 m3 ha-1 was found, with a standard deviation (SD) of 47.9 m3 ha-1. The proportion of deadwood volume was also reported in relation to the overall stand volume (living trees plus deadwood components). Val Cervara was characterized by the largest proportion of deadwood (28.2% of the total volume), while the other sites showed deadwood proportions lower than 10%, with the exception of Abeti Soprani and Area Pavari, where the proportion was 14.4% and 11.8%, respectively.
Abundance of microhabitats
The abundance of TreMs (as number of TreMs) significantly varied among the study sites and between the different components. Tab. 4 reports the total number of TreMs and the number of TreMs per living tree and per each deadwood component.
Tab. 4 - Abundance of TreMs on living trees and deadwood components. (Year): time since the last harvest; (No): number of trees (for living trees) and number of woody debris (for deadwood components) with presence of at least one TreM; (No-Tr %): percentage of living trees or deadwood components with at least one TreMs; (N-TreM): total number of TreMs; (M-TreM): mean number of TreMs per tree ± standard deviation; (SD): standard deviation.
Components | Site | Year | No | No-Tr % | N-TreM | M-TreM |
---|---|---|---|---|---|---|
2612 Living trees 362 TreMs |
Abeti Soprani | 31 | 6 | 1.0 | 6 | 0.01 ± 0.10 |
Collemeluccio | 51 | 9 | 1.6 | 11 | 0.02 ± 0.16 | |
Montedimezzo | 56 | 17 | 4.9 | 23 | 0.07 ± 0.31 | |
Area Pavari | 58 | 17 | 8.9 | 34 | 0.18 ± 0.71 | |
Bosco Aschiero | 310 | 50 | 18.1 | 91 | 0.33 ± 0.85 | |
Fonte Novello | 312 | 50 | 12.3 | 64 | 0.16 ± 0.48 | |
Val Cervara | >500 | 60 | 25.8 | 133 | 0.57 ± 1.18 | |
Mean | - | 30 | 10.4 | 52 | 0.19 | |
SD | - | 23 | 9.1 | 47 | 0.20 | |
1704 Deadwood components 1178 TreMs |
Abeti Soprani | 31 | 35 | 8.8 | 75 | 0.20 ± 0.65 |
Collemeluccio | 51 | 38 | 14.8 | 64 | 0.25 ± 0.67 | |
Montedimezzo | 56 | 5 | 2.6 | 12 | 0.05 ± 0.40 | |
Area Pavari | 58 | 37 | 20.9 | 91 | 0.54 ± 1.11 | |
Bosco Aschiero | 310 | 12 | 6.8 | 24 | 0.13 ± 0.52 | |
Fonte Novello | 312 | 153 | 54.1 | 316 | 1.14 ± 1.10 | |
Val Cervara | >500 | 225 | 100.0 | 596 | 2.64 ± 1.67 | |
Mean | - | 72 | 29.7 | 168 | 0.71 | |
SD | - | 83 | 35.4 | 214 | 0.96 |
The survey on the 2612 living trees revealed a total number of 362 TreMs. Only 10.4% of living tree had at least one TreM (Tab. 4). Considering all the study sites, on average, 52 TreMs were found for living trees with a standard deviation of 47 and high heterogeneity across the study sites. The highest number of TreMs was recorded in the sites unmanaged for more than one century, namely Fonte Novello (64 TreMs), Bosco Aschiero (91 TreMs), and Val Cervara (133 TreMs).
On average, 0.19 TreMs per living tree, with a SD of 0.2 were observed. Particularly, they increased with long time since the last harvest, as in Val Cervara (0.57 TreMs per tree) and Bosco Aschiero (0.33 TreMs per tree). The lowest number of TreMs per tree was recorded in Abeti Soprani (0.01 TreM per tree) and Collemeluccio (0.02 TreMs per tree), both characterized by short time since the last harvest (30-50 years).
The abundance of TreMs (N-TreMs) was higher on deadwood components than on living trees. The survey on the 1704 deadwood components revealed a total of 1178 TreMs, occurring on 505 deadwood components. About 30% of deadwood elements had at least one TreM (Tab. 4). A higher number of TreMs was observed in the sites unharvested for very long time, namely Fonte Novello (316 TreMs) and Val Cervara (596 TreMs).
On average, 0.71 TreMs, with a SD of 0.96, were recorded on deadwood components, revealing a higher value than that obtained for living trees (0.19 TreMs per tree). The highest number of TreMs per deadwood component was recorded in Val Cervara (2.64), characterized by a long time since the last harvest, in Fonte Novello (1.14) and in Area Pavari (0.54), where the presence of living trees and deadwood components with high diameter values was more frequent.
In Fig. 2 the mean values of TreMs and their variability are reported for living trees and deadwood components; the observed variability was always very high. The largest number of TreMs per element was found on snags (1.4), CWD (0.8) and DDT (0.8), while less TreMs per element were recorded on living trees (0.2) and STD (0.3). Moreover, the Kruskal-Wallis test revealed highly significant differences (Kruskal-Wallis χ2 = 514.88, df = 5, p<0.0001) between medians. The pair-wise Conover test showed significant difference between snags, CWD and DDT and all the other components, while no significant differences were observed between living trees and STD. Furthermore, the number of TreMs for each element was always higher in sites where human-related disturbances had not occurred for more than 300 years. Finally, among the deadwood components, in absolute terms, the highest number of TreMs occurred on CWD (694) and on snags (185), while the lowest number of TreMs was observed on SDT (76).
Fig. 2 - Number of TreMs recorded on each element (living trees and deadwood components) and represented as box-plot distributions: CWD (coarse woody debris), DDT (dead downed trees), LV (living trees), SDT (standing dead trees), snags and stumps. In the box plot, the upper limit corresponds to the value of the first quartile (Q1) of the distribution and the lower limit to the third quartile (Q3); the heavy horizontal line is the median. Errors bars connect the two extreme values, obtained as a linear combination of Q1 and Q3 values. Cases with values more than three-box lengths, from the upper or lower edge of the box, are shown as open circles. The closed circles inside the box is the mean number of TreMs per element. The Kruskal-Wallis test and the pairwise Conover non-parametric test were applied.
Fig. 3 shows, for each type of TreMs, the total number of TreMs recorded on living trees and deadwood components. A high number of M21 (on average 58) and M22 (on average 60) was observed on deadwood components, strictly linked to the activity of saproxylic insects. Fungal structures (M1, M2, and M3) were almost exclusively present on deadwood components, especially on the CWD. On average, these TreMs ranged between 6 for M1 and 16 for M3. On living tree, the above mentioned TreMs (fungi) also occurred, but outnumbered. Cavities were frequently represented by M12, characterizing mostly living trees (on average 6 TreMs) and, to a lesser extent, deadwood components, mainly snags. Also, other cavities with or without mould (M14 and M15), occurred more frequently on living trees than on deadwood components, while the bark loss was recorded only on living trees (M19). Finally, two types of TreMs were totally absent, M6 (broken forks) and M13 (cavity strings).
Fig. 3 - Number of each type of TreMs (average values and related variability), occurring in all sites divided by types, for living trees and for deadwood components. The error bars represent the standard deviation of the mean.
Diversity of microhabitats
The variability and the mean values of the Shannon diversity index (SI) related to TreMs diversity, for each investigated component and for all the study sites, are reported in Fig. 4. PERMANOVA analysis showed that SI was significantly higher in living trees than in all the deadwood components. Particularly, a higher diversity of TreMs was observed in Area Pavari, Fonte Novello and Val Cervara more than the other study sites. These three sites differed from the other for the time since the last harvest (about 60 years for Area Pavari and more than 300 years for Fonte Novello and Val Cervara), but they had similar structural stand characteristics, in particular the occurrence of trees with stem DBH higher than 80 cm. Conversely, Collemeluccio and Abeti Soprani showed the lowest SI; these study areas had the highest number of trees with stem DBH ranging between 10 and 30 cm, but also unmanaged for 30-50 years. Moreover, a positive correlation between SI and the years since the last harvest was observed for living trees, while no correlations were observed for all the other components.
Fig. 4 - Variability and median values of Shannon’s Index related to TreMs, occurring on each investigated component, in each study sites (AS: Abeti Soprani; COLL: Collemeluccio; MdM: Montedimezzo; AP: Area Pavari; ASCH: Bosco Aschiero; FN: Fonte Novello; VC: Val Cervara).
DDT were found only in three sites (Area Pavari, Fonte Novello and Val Cervara), showing high diversity of TreMs (mean SI = 1.63). Area Pavari was characterized by the presence of trees with high DBH values. A lower SI (on average, 1.43) was found for snags, with the highest value found in Val Cervara (2.04). However, the PERMANOVA test showed no significant differences for the diversity of TreMs between snags and DDT. Also, CWD, SDT and stumps had the lowest diversity of TreMs, with SI ranging between 1.02 and 1.06, without significant differences between these components. The SI obtained for CWD was similar in all sites, while for SDT and stumps the lowest values were recorded in Montedimezzo and Collemeluccio.
Correlation between variables and modelling of number of microhabitats per tree
Significant correlations between the number of TreMs and the diameter of deadwood elements (r=0.37; p<0.001), the deadwood length (r=0.31; p<0.001), and the time since the last harvest (r=0.55; p<0.001) were observed. Moreover, a significant correlation between the deadwood volume and the number of TreMs (r=0.34; p<0.001) was also observed, while the deadwood decay stage (r=0.14; p=0.125 did not influence the number of TreMs.
For living trees, the number of TreMs were significantly correlated with the diameter of tree stems (r=0.42; p<0.001) and the time since the last harvest (r=0.37; p<0.001), but also with the tree volume (r=0.27; p<0.001). Conversely, no significant correlations were found between tree height (r=0.022; p=0.264) or the height of crown insertion point (r=0.06; p=0.758) and number of TreMs.
Tab. 5 shows the results of the linear mixed model procedure applied to the models [1-7] to test the effect of the considered variables on the number of TreMs per living tree. In the one-variable models each model was significant, even if the model with the lowest AIC value was the model [1], with diameter as the predictive variable (AIC = 1677). In the complete additive model [6], the stepwise procedure eliminated all variables (in each step, a variable was considered for addition to or subtraction from the set of explanatory variables based on F-tests), except for the DBH and YEAR, the only variables that had a significant effect on the number of TreMs per living tree, but with a higher AIC value (1779) than in model [1].
Tab. 5 - Values of the estimates derived from generalized fixed and random effects models to test the effect of independent variables on abundance of TreMs per living tree. The independent variables were the following: tree DBH; (YEAR): years since the last harvest; (HT): tree height; (HC): height of crown insertion point; (VOL): tree volume. The selected model is [7]. Models were run over the whole dataset (in total 2612 trees). (***): p < 0.0001;
Model | Variable | Estimate | Std. Error | z-value | Pr(>|z|) | AIC |
---|---|---|---|---|---|---|
[1] One-variable | Intercept | -0.1129 | 0.0785 | -8.708 | <2.0e-16 *** | 1677 |
DBH | 0.0088 | 0.0005 | 19.096 | <2.0e-16 *** | ||
[2] One-variable | Intercept | -0.0041 | 0.0541 | -7.594 | 3.1e-14 *** | 2021 |
YEAR | 0.0010 | 0.0002 | 3.568 | 0.00036 *** | ||
[3] One-variable | Intercept | -0.0640 | 0.0911 | -7.267 | 3.69e-13 *** | 1905 |
HT | 0.0128 | 0.0015 | 11.109 | <2.0e-16 *** | ||
[4] One-variable | Intercept | 0.1219 | 0.0853 | -5.177 | 2.26e-07 *** | 2010 |
HC | 0.0069 | 0.0025 | 4.255 | 2.09e-05 *** | ||
[5] One-variable | Intercept | 0.0389 | 0.0769 | -5.767 | 8.09e-09 *** | 1685 |
VOL | 0.0767 | 0.0038 | 19.634 | <2.0e-16 *** | ||
[6] Additive complete | Intercept | 0.0292 | 0.0581 | -12.060 | <2.0e-16 *** | 1799 |
DBH | 0.0049 | 0.0011 | 10.300 | <2.0e-16 *** | ||
YEAR | 0.0008 | 0.0002 | 4.590 | 4.43e-06 *** | ||
HT | -0.0116 | 0.0026 | -1.672 | 0.0945 | ||
HC | -0.0079 | 0.0033 | -0.544 | 0.5866 | ||
VOL | 0.0702 | 0.0071 | -0.059 | 0.9531 | ||
[7] Combined model | Intercept | 0.0032 | 0.0453 | -15.491 | <2.0e-16 *** | 1465 |
DBH2 · YEAR | 5.38e-07 | 2.00e-08 | 19.276 | <2.0e-16 *** |
By comparing and transforming several combinations of these two variables, we found that the model with the lowest AIC value (1465) was the combined model between squared DBH and YEAR, expressed as a single predictor (DBH2 · YEAR). Therefore, the model [7] was the model that estimated better the number of microhabitats per tree. Finally, for this model, the variance inflation factor (VIF) was lower than 2; therefore, the absence of multicollinearity was supported ([46]). Based on this model, the number of TreMs per living tree increased when tree diameter and the time since the last harvest were higher (Fig. 5).
Fig. 5 - Number of TreMs per tree in relation to the diameter at breast height of trees (DBH) and to the years since the last harvest (YEAR) based on raw data. The two variables are expressed as single predictor (DBH2 · YEAR). Sample size = 2612 trees.
Discussion
This study aimed at identifying tree attributes associated with higher number and diversity of TreMs, supporting the survey of forest biodiversity in Mediterranean mountain environments. The size of trees, in particular stem DBH, was strongly associated with both the abundance and the diversity of TreMs. In addition, the time since the last harvest also had an important role, significantly affecting the probability that TreMs could be formed in a long-term perspective. This indicates that forests left unmanaged for a long time (centuries) will develop structural elements for higher diversity and abundance of TreMs per each component, in comparison with stands in which the last harvest dates back a few decades ([37]). The analyses recognized the stem DBH as the most relevant dendrometric parameter useful to monitor and assess the number of TreMs per living tree, followed by the time since the last harvest. The combination of DBH and time since the last harvest provided a better model than including the two variables separately, in part due to the relationship between these two predictors, but also because these two factors together may better represent the transition of a previously managed forest to a more natural state over time.
A positive relationship between the stem DBH and the abundance of TreMs was also observed in other environmental conditions ([13], [14], [37], [43]). However, this relationship cannot be generalized. Indeed, in the forest stands investigated in this study, when the last harvest dated back a few decades, the abundance of TreMs did not vary significantly as the stem DBH increased. These results are coherent with the observations of Winter & Möller ([45]), who found no significant relationships between the stem DBH and the abundance of TreMs in managed forests and in stands in which the last harvest dated back a few decades. However, Paillet et al. ([29]) and Vuidot et al. ([43]) found a relationship with diameter even after a few decades of abandonment, but also in managed forests ([43]). Conversely, positive correlations between the abundance of TreMs and the stem DBH were found in forests left unmanaged for centuries. A greater variability of disturbance-generated TreMs and stand structural elements could possibly be found on a long-time scale, owing to the time needed to develop habitat and resource requirements for forest organisms. The effect of forest management, or its absence, on the abundance of TreMs was also shown in other studies ([25], [44]). At tree level, Vuidot et al. ([43]) demonstrated that, whatever form of forest management, the number and type of TreMs were mainly influenced by individual tree features, and the probability of a certain density of TreMs was the same for similar trees.
Especially for living trees, abundance of TreMs was higher in forests that had been left unmanaged for long. Conversely, abundance of TreMs was much lower in forests in which the last harvest dated back a few decades (30-50 years). This highlighted how the time since the last harvest was a major factor in determining forest structure (e.g., stem DBH, tree density) and microclimate (e.g., light penetration, moisture availability), in turn affecting the formation of TreMs on living trees. Regnery et al. ([37]) obtained similar results in Mediterranean forests. Vuidot et al. ([43]), Larrieu & Cabanettes ([13]) and Regnery et al. ([37]) found that snags were richer in TreMs than living trees. In unmanaged forests located in mountainous regions of France, Paillet et al. ([28]) found that the higher density of TreMs was not only due to the higher occurrence of favourable elements (e.g., large trees and snags), but also to the fact that trees might host more TreMs when the forest was unharvested for long. Furthermore, it was also confirmed that large trees and dead trees could host more TreeMs than their smaller or living counterparts did ([29]). Also, in our study sites, regardless of the environmental conditions, the abandonment of management practices favoured the abundance and diversity of TreMs.
We found that snag (1.4 TreMs per element) and CWD (0.8 TreMs per element) were the richest deadwood components in TreMs (in total 879 TreMs, equal to 74.6% of all TreMs recorded). The role of CWD in providing TreMs was particularly important when the woody debris occurring on the forest floor were of large dimensions. This was particularly evident in forest stands that had been unmanaged for more than 100 years. The abundance of TreMs on CWD was high, though the lack of studies that consider the abundance of TreMs on this deadwood component hinders any comparison. In analogy with the single large vs. several small discussion ([17]), it is still unclear whether high biodiversity is associated with higher availability of several small elements rather than to one single large element. Indeed, at the plot level, since each tree is a potential host of TreMs, forest stands with a low number of trees may also show a reduction in the abundance and diversity of TreMs ([10]). Conversely, we observed that the highest diversity of TreMs was observed in Area Pavari, where the tree density was very low (191 trees per hectare). By contrast, in Abeti Soprani, in which 591 trees per hectare occurred, the diversity of TreMs was lower. We suggest that tree size and stand characteristics had key role in determining the abundance of TreMs. In particular, trees in Area Pavari were characterized by very large size, then increasing the probability to host valuable TreMs combinations for biodiversity conservation. In this sense, the preservation of big living trees, large deadwood elements, and old-growth forest patches might help promote structural diversity, in turn preserve TreMs. Nevertheless, a combination of single large and several small deadwood elements (and living trees) might represent a good compromise in sustainable forest management approaches, but it should be evaluated in relation to the species at stake.
In addition, differences in the abundance of TreMs among sites were influenced by the time since the last harvest. We found that the abundance of TreMs, especially for deadwood components, in comparison with living trees, was higher in those sites in which the last harvest dated back more than three centuries. Higher amount of TreMs on deadwood components than on living trees was also detected in deciduous oak forests, in central Italy, by Parisi et al. ([32]). An exception was observed in Bosco Aschiero, where living trees hosted more numerous TreMs than deadwood components. Studying the abundance of TreMs on living trees or on SDT and snags has often been favoured ([28]), while few studies ([44], [30], [32]) have considered the abundance of TreMs on other deadwood components, such as stumps, CWD and DDT. Considering the deadwood components, the most common TreMs were the holes and the system of gallery (M22 and M23) originated by saproxylics ([2], [31]).
The most numerous TreMs observed on living trees was M4 (broken crown). The number of M4 found in our sites (16 TreMs per ha) was higher than in Paillet et al. ([28]) and in Bouget et al. ([2]), who observed 8-12 and 5-8 TreMs per ha, respectively. In these Mediterranean mountains, heavy snow and windstorms may cause considerable damage to tree crowns, particularly in old trees, making them susceptible to secondary attacks by pests and fungi. The resulting array of substrates and resources for decomposers is of relevance for conservation ([1], [31]). A better understanding of TreMs dynamics and of the complex interrelations between forest biodiversity and environmental disturbance is warranted to integrate monitoring protocols into conservation strategies and sustainable management of forests. Some proportions of all development phases of the forest, including senescence patches and canopy gaps, caused by the death of one or more trees, as well as different stand densities, should be regarded as having high conservation value in recognition of the role they play in ecosystem processes and in maintaining biodiversity in these Mediterranean mountain forests of high nature value.
In total, the CWD hosted more TreMs (694) than other deadwood components. However, SI highlighted how the diversity of TreMs was particularly high on living trees. Snags and DDT were also characterized by fair diversity of TreMs. Paillet et al. ([28]) recognized snags as substrates that might increase the occurrence of TreMs. Yet, SDT might favour the occurrence of TreMs, either in terms of total number ([43]), diversity, or types ([14]). For living trees, high SI was observed in Val Cervara and Fonte Novello, and then in Area Pavari and Bosco Aschiero. These sites were characterized by a similar stand structure, especially for the presence of large trees (DBH>80 cm). For this reason, the time since the last harvest was not the main factor influencing the abundance of TreMs. As an example, in Area Pavari, where the last harvest dated back at least six decades, SI was higher than in Bosco Aschiero, unmanaged for more than three centuries. Indeed, the stem DBH was the principal dendrometric parameter that influenced the abundance of TreMs and a positive correlation between stem DBH of living trees and the abundance of TreMs was also observed in other studies ([37], [28]). In addition, the presence of large trees might also increase the diversity and the abundance of some particular types of TreMs ([15], [43]), such as bark loss or branch damages ([25]).
The presence of large trees could, therefore, be considered an appropriate indicator of forest biodiversity ([19], [18]), also in these Mediterranean mountain areas, and provide effective monitoring schemes and help identify alternative management strategies. As an example, variable-density thinning might contribute to increase the relative proportion of large trees, in turn enhancing the density and spatial distribution of TreMs. Nevertheless, also small trees would provide TreMs ([6]). Whether TreMs can be used as valuable forest structure indicators of biodiversity in forest monitoring schemes is still a matter of debate. Indeed, it is a matter of fact that TreMs should be always integrated with the measurement of other indicators, such as deadwood (e.g., standing dead trees and snags), in evaluating biodiversity, especially for some taxa, such as birds and bats ([27]).
Referring to perspectives for forest management, results may help understand trade-offs of integrative vs. segregative approaches for the conservation of forest biodiversity. Old-growth forests provide unique habitats for numerous species of animals and fungi that evolved in environmental conditions regulated by natural processes ([39]). Old-growth forest patches might help to better recover large-scale effects of forest management in Mediterranean landscapes of Europe, restoring biodiversity in degraded areas on a regional scale. The segregation of high nature value forests would provide habitat for old-growth dependent species, balancing sustainable timber production targeted in managed forests. Nevertheless, the integration of nature conservation and economic management should still consider late-successional forest stages to compensate rotations for timber production that are much shorter than the lifespan of trees. Unravelling these complex relationships between forest structure, component size, and species diversity requires further research in both old-growth and managed forests across Europe. Therefore, for conservation purposes at the landscape scale, the occurrence of all the successional phases of a forest stand should be favoured ([8]). In this sense, biomass demands might interfere with conservation strategies in these Mediterranean mountain forests, and wise management practices need to be adopted to balance trade-offs between increasing demand for fuel wood and decreasing proportion of habitat trees in managed forests.
Conclusions
Tree features affected the abundance and diversity of TreMs in unmanaged forests in the Italian Apennines. Abundance and diversity of TreMs were mainly influenced by the stem DBH and the years since the last harvest. The combination of these two factors highlighted a significant increase of TreMs abundance and diversity in these old-growth forest patches.
Structural diversity at the stand scale increased proportionally with the time since the last harvest. Restoration towards old forest structures, such as extending rotation periods and retaining large trees (i.e., habitat trees), as well as snags and CWD, might represent suitable management strategies to preserve important TreMs and biodiversity in managed forests of high nature value in Mediterranean mountain ranges, while maintaining forest production.
Considering the current pattern of land abandonment in the Apennines, as well as other mountain areas throughout Europe, evidence on the abundance and types of TreMs would become useful indicators of naturalness within national forest inventory protocols and monitoring schemes, as anticipated for deadwood. Indeed, TreMs might act as biodiversity incubation centres, and their occurrence and types could be easily recorded in the field and be implemented in guidelines and prescriptions for the conservation of biodiversity, especially when managing forests for complexity ([35]).
Acknowledgements
The research is linked to activities conducted within the COST (European Cooperation in Science and Technology) Action CLIMO (Climate-Smart Forestry in Mountain Regions - CA15226) financially supported by the EU Framework Programme for Research and Innovation HORIZON 2020.
We thank the anonymous reviewers for their careful reading of our manuscript; their many insightful comments and suggestions significantly helped us to improve and clarify the manuscript.
References
CrossRef | Gscholar
CrossRef | Gscholar
CrossRef | Gscholar
CrossRef | Gscholar
Gscholar
Gscholar
Gscholar
Authors’ Info
Authors’ Affiliation
Fabio Lombardi 0000-0003-3517-5890
Dipartimento di Agraria, Università Mediterranea di Reggio Calabria (Italy)
Roberto Tognetti 0000-0002-7771-6176
Dipartimento di Agricoltura, Ambiente e Alimenti, Università degli Studi del Molise, Campobasso (Italy)
Dipartimento di Bioscienze e Territorio, Università degli Studi del Molise, Pesche, IS (Italy)
Dipartimento di Gestione dei Sistemi Agrari, Alimentari e Forestali, Università degli Studi di Firenze (Italy)
Dipartimento per l’Innovazione nei Sistemi Biologici, Agroalimentari e Forestali, Università degli Studi della Tuscia, Viterbo (Italy)
Corresponding author
Paper Info
Citation
Marziliano PA, Antonucci S, Tognetti R, Marchetti M, Chirici G, Corona P, Lombardi F (2021). Factors affecting the quantity and type of tree-related microhabitats in Mediterranean mountain forests of high nature value. iForest 14: 250-259. - doi: 10.3832/ifor3568-014
Academic Editor
Michele Carbognani
Paper history
Received: Jul 01, 2020
Accepted: Mar 25, 2021
First online: May 21, 2021
Publication Date: Jun 30, 2021
Publication Time: 1.90 months
Copyright Information
© SISEF - The Italian Society of Silviculture and Forest Ecology 2021
Open Access
This article is distributed under the terms of the Creative Commons Attribution-Non Commercial 4.0 International (https://creativecommons.org/licenses/by-nc/4.0/), which permits unrestricted use, distribution, and reproduction in any medium, provided you give appropriate credit to the original author(s) and the source, provide a link to the Creative Commons license, and indicate if changes were made.
Web Metrics
Breakdown by View Type
Article Usage
Total Article Views: 31326
(from publication date up to now)
Breakdown by View Type
HTML Page Views: 26700
Abstract Page Views: 2175
PDF Downloads: 2047
Citation/Reference Downloads: 7
XML Downloads: 397
Web Metrics
Days since publication: 1280
Overall contacts: 31326
Avg. contacts per week: 171.31
Article Citations
Article citations are based on data periodically collected from the Clarivate Web of Science web site
(last update: Feb 2023)
Total number of cites (since 2021): 4
Average cites per year: 1.33
Publication Metrics
by Dimensions ©
Articles citing this article
List of the papers citing this article based on CrossRef Cited-by.
Related Contents
iForest Similar Articles
Research Articles
Biodiversity conservation and wood production in a Natura 2000 Mediterranean forest. A trade-off evaluation focused on the occurrence of microhabitats
vol. 12, pp. 76-84 (online: 24 January 2019)
Research Articles
Early responses of biodiversity indicators to various thinning treatments in mountain beech forests
vol. 11, pp. 609-618 (online: 25 September 2018)
Research Articles
Long-term effects of single-tree selection cutting management on coarse woody debris in natural mixed beech stands in the Caspian forest (Iran)
vol. 10, pp. 652-658 (online: 20 June 2017)
Research Articles
Effect of topography on tree species composition and volume of coarse woody debris in an Oriental beech (Fagus orientalis Lipsky) old growth forests, northern Iran
vol. 9, pp. 658-665 (online: 17 March 2016)
Research Articles
Exposure elevation and forest structure predict the abundance of saproxylic beetles’ communities in mountain managed beech forests
vol. 16, pp. 155-164 (online: 08 June 2023)
Research Articles
Assessment of the protective function of forests against debris flows in a gorge of the Slovenian Alps
vol. 8, pp. 73-81 (online: 17 June 2014)
Research Articles
Investigating the effect of selective logging on tree biodiversity and structure of the tropical forests of Papua New Guinea
vol. 9, pp. 475-482 (online: 25 January 2016)
Research Articles
Stand structure and deadwood amount influences saproxylic fungal biodiversity in Mediterranean mountain unmanaged forests
vol. 9, pp. 115-124 (online: 08 September 2015)
Research Articles
Effects of silvicultural thinning on stand structure and coarse woody debris in the deciduous Arasbaran forest, Iran
vol. 16, pp. 377-384 (online: 19 December 2023)
Research Articles
Assessment of hedge stand types as determinants of woody species richness in rural field margins
vol. 6, pp. 201-208 (online: 27 May 2013)
iForest Database Search
Search By Author
Search By Keyword
Google Scholar Search
Citing Articles
Search By Author
Search By Keywords
PubMed Search
Search By Author
Search By Keyword