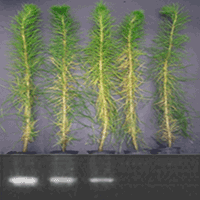
Tolerance to heavy metal stress in seedlings of three pine species from contrasting environmental conditions in Chile
iForest - Biogeosciences and Forestry, Volume 9, Issue 6, Pages 937-945 (2016)
doi: https://doi.org/10.3832/ifor1908-009
Published: Aug 12, 2016 - Copyright © 2016 SISEF
Research Articles
Abstract
Tolerance to metal stress was studied in seedlings of three pine species (Pinus radiata, P. pinaster and P. canariensis) under controlled ex vitro conditions. Mature female cones were randomly collected at two sites in Chile (Llico and Huilquilemu) characterized by contrasting environmental conditions. One-year-old pine seedlings were immersed in trays with solutions of CuSO4 (300 mM) or AlCl3 (100 mM), and their survival, growth rate and decay symptoms were recorded for 60 days. Results showed large differences among provenances in seedling tolerance to CuSO4 and AlCl3 in terms of survival and growth. Multivariate analysis revealed a significant association (p <0.0001) between the first canonical function and the following variables: provenance, species, metal stress and growing rate, as well as between the second canonical function and provenance, species, metal stress and symptomatology, indicating a high degree of genotype-environment interaction. Moreover, the activity of POX, SOD and CAT enzymes was determined 60 days after the beginning of the experiment in the Llico provenance seedlings. P. pinaster showed the highest activity level for all the enzymes considered, while P. canariensis and P. radiata had intermediate and lowest values, respectively. Differential gene expression among pine seedlings under metal stress with CuSO4 for two genes (Cu-Zn-superoxide dismutase and RuBisCo) confirmed P. pinaster as the most tolerant species to CuSO4 treatment. Our results are consistent with the hypothesis that abiotic stress in the maternal environment can induce a “transgenerational plasticity” which could affect progeny performances. The influence of different genetic backgrounds on the tolerance to heavy metals in pine seedlings is also discussed.
Keywords
Pinus spp., Heavy Metals, Abiotic Stresses, Genotype-Environment Interaction
Introduction
Soil pollution by heavy metals is a global environmental issue and a consequence of the escalation of mining and mineral processing, whose spread of metal residues contaminated large areas in Chile ([6], [32]). Metals accumulate in plant organs and tissues causing both physiological and biochemical changes, growth reduction and yield loss, particularly after persistent exposure ([41], [30]). An increase in reactive oxygen species (ROS) is a consequence of metal accumulation. Moreover, ROS are destructive if protective antioxidant mechanisms are not activated efficiently ([12]).
Copper (Cu) is an essential micronutrient with relevant physiological roles in plant growth and development. However, excessive Cu can be lethal to plants, causing a range of deleterious effects, such as the inhibition of photosynthesis and pigment synthesis, plasma membrane damage and other metabolic disturbances. The Cu concentration in plant leaves ranges from 5-30 µg g-1 dry weight (DW). Furthermore, some plants have developed mechanisms for metal detoxification, including exclusion, compartmentalization and binding to organic ligands such as organic acids, amino acids, phytochelatins (PCs), and metallothioneins (Mts - [15], [25]). On the other hand, increasing areas of agricultural lands are acidic, where soil contains high quantities of aluminum (Al - [28]) which is highly toxic to plant growth. Several studies identified Al as a cause of ROS over-production and lipid peroxidation, which affect antioxidant enzyme activities in root tips ([37], [42], [24]). Furthermore, aluminum-induced cell death has been observed after exposure to high levels of Al3+ ([10], [37]). Therefore, a strong connection between excess Al and oxidative stress has been proposed in plants ([10], [44]).
To survive oxidative damage, plants have developed non-enzymatic and enzymatic systems to regulate the intracellular levels of ROS ([4], [36]). The main non-enzymatic antioxidants are ascorbate (AsA) and glutathione (GSH), while the antioxidative enzymes are Superoxide dismutase (SOD, EC 1.15.1.1), Peroxidase (POD, EC1.11.1.7), Catalase (CAT, EC 1.11.1.6), AsA-GSH cycle-related ascorbate peroxidase (APX, EC 1.11.1.11), Monodehydro ascorbate reductase (MDHAR, EC 1.6.5.4), Dehydro ascorbate reductase (DHAR, EC 1.8.5.1), Glutathione peroxidase (GPX, EC 1.11.1.9) and Glutathione reductase (GR, EC 1.6.4.2 - [21]). Higher activities of antioxidant enzymes facilitate the removal of excessive ROS and suppress lipid peroxidation, which could be beneficial for plant performance under Al stress ([4], [31]).
Current research on metal tolerance in plants is mainly focused to their adaptation to very restrictive environmental conditions. Our hypothesis was that transgenerational plasticity might influence progeny performance, resulting in a higher probability of selecting tolerant individuals. Thus, the aim of our work was to evaluate the tolerance of three Pinus spp. species, derived from mother plants selected in extreme agroforestry systems, to increasing concentrations of Cu and Al under controlled ex vitro conditions. Both biochemical and differential gene expression analyses were conducted to support the tolerance screening experiments. The final goal of thi study was the selection of Cu- and Al-tolerant Pinus spp. plants, suitable for greenhouse or trials under metal contamination.
Materials and methods
Plant material
Mature female cones from P. radiata, P. pinaster and P. canariensis were randomly collected from two contrasting localities: (a) the Forestry Reserve “Laguna Torca” (CONAF - Corporación Forestal Nacional, Chilean Forest Service) is a protected area located in Llico (34° 46′ S, 72° 6′ W), near to a marine environment with a mixture of sandy soil; (b) the Experimental Station of the Catholic University of Maule located in Huilquilemu (35° 27′ S, 71° 34′ W) in the Central Valley is characterized by arable and fertile soil. Both localities belong to the Maule Region, Chile, though they are geographically separated by the coastal mountain range.
For seed release, the cones were immersed in hot water (50 °C) for 2 min and then transferred to 40 °C for 48 h (dry treatment). Racks of 64 small pots containing a soil vermiculite mixture (1:1) were used for single-seed sowing. At the beginning of the spring season (August), seed germination was carried out in ex vitro controlled conditions.
Experimental treatments
One-year-old seedlings from P. radiata, P. pinaster and P. canariensis populations were selected for abiotic stress experiments with CuSO4 and AlCl3. Seedlings were planted in single plastic pots containing the vermiculite soil mixture. The roots of the pine plants were immersed in trays with solutions of increasing CuSO4 concentrations (100 mM, 200 mM, 300 mM) or AlCl3 (50 mM, 100 mM). The combinations of Pinus spp. genotypes (populations) with metal stress treatments resulted in a total of 15 experimental variants per site (Llico, Huilquilemu). Control treatments for the three pine species in each locality were immersed in distilled water. A total of 96 plants per treatment were placed in two replica trays (48 pots/tray) following a factorial block design. A total of 72 trays (60 for treatments, 12 for controls) were used in the experiment. During the beginning of the spring season (August-September) the experiment was conducted under ex vitro controlled conditions, while plantlets were totally covered with protective mesh (sunlight reduced by 50%). Both fresh weight (FW) and dry weight (DW) at T0 (before the stress treatments) and TF (after 60 days of stress treatments) were determined for needles (N), stem (S) and roots (R) of individual Pinus spp. seedlings. The number of dead plants (NDP), number of symptomatic plants (NSP) and the growing rate (GR) under treatments were estimated at 0, 15, 30, 45 and 60 days.
Samples for enzyme activity determination and DNA analysis
Approximately 1.0 g of needles (20 plants, two needles per plant) for each block (48 plants) of treatments, were pooled at 0, 15, 30, 45 and 60 days. Plants were randomly selected including both symptomatic and asymptomatic individuals. Two replicas per treatment were determined for the enzymatic activities. For RT-PCR analysis, a single sample per treatment was conducted pooling one needle of 40 randomly selected plants per treatment.
Peroxidase (POX) assay
POX activity was assessed according to Bania & Mahanta ([7]). Briefly, 2 ml of phosphate buffer (pH 6.0/7.0), 100 µl of plant extract, and 1 ml of O-dianisidine solution were mixed. The reaction was initiated by adding 100 µl of 0.2 mM H2O2 and the absorbance was read at 460 nm at 30 second intervals for 5 minutes. Peroxidase activity was calculated using an extinction co-efficient of O-dianisidine and enzyme activity was expressed as unit per mg of protein.
Superoxide dismutase (SOD) assay
SOD activity was monitored according to the method from Roth & Gilbert ([34]) and modified by Kumar et al. ([27]). One ml of reaction mixture contained 50 mM sodium phosphate buffer (pH 7.8), 100 mM EDTA, 20 ml enzyme extract, and 10 mM pyrogallol. Enzyme activity [U (mg protein)-1] was calculated by monitoring the reaction mixture for 120 s (at 60 s intervals) at 420 nm in a spectrophotometer.
Catalase (CAT) assay
CAT activity was assayed by measuring the initial rate of H2O2 disappearance using the method of Beers & Sizer ([8]) reported by Kumar et al. ([27]). One ml of catalase assay reaction mixture contained 0.05 mM sodium phosphate buffer (pH 7.0), 20 ml enzyme extract and 1 mM H2O2. The decrease in H2O2 was followed by a decline in A240, and the activity [U (mg protein)-1] was calculated using a molar absorption coefficient of 40 mM-1 cm-1 for H2O2.
RT-PCR analysis
RT-PCRs were conducted according to Yang et al. ([43]). RNA was extracted from the leaves (middle part) pooled from separate replicates. Extractions were performed using the TRIzol® reagent (Invitrogen, Carlsbad, CA, USA). One g of pooled, frozen leaf tissue was ground to a fine powder in the presence of liquid nitrogen, and then mixed with 10 ml TRIzol. RNA sample concentrations were quantified by determining the 260:280 and 260:230 nm ratios by spectrophotometry and further confirmed on a 1.2% agarose/0.4 M formaldehyde gel. Twenty ng of high quality RNA was added as template to each reaction using the Enhanced Avian HS RTPCR kit® (Sigma, St. Louis, MO, USA). For specific RT-PCR amplification, genes were selected and oligonucleotide primers were designed using sequences from the GenBank public databases (⇒ http://⇒ www.ncbi.nlm.nih.gov). Information on primer source, nucleotide sequence, and PCR annealing temperatures are listed in Tab. 1.
Tab. 1 - Information of the selected genes analyzed by RT-PCR in Pinus spp.
No | Accession number |
Protein/ Function |
Primers (5’-3’) | Tm (oC) |
Size (bp) |
---|---|---|---|---|---|
1 | AF434186 | P. pinaster Cu-Zn-superoxide dismutase precursor |
l-ATAGTTGCGGGTTCTGATGG r-ATGGGAGTGAGTCCAACCAC |
59.96 59.82 |
203 |
2 | AJ309096 | P. pinaster ribulosebisphosphate carboxylase (RuBisCo) small chain |
l-AACAAGTGGGTGCCTTGTCT r-CACCCAGTATCTCCCATCGT |
59.62 59.80 |
210 |
The PCR reaction mixture consisted of 3 μl of first strand cDNA, 5 μl of 10× PCR buffer, 5 μl of 25 mM MgCl2, 1 μl of dNTPs (10 mM each), 1 μl of each 10 μM primers (forward and reverse), 1 U of Taq DNA polymerase (Invitrogen), and ultrapure water (Sigma) to a final volume of 50 μl. PCRs were conducted with the following parameters: 94 °C for 3 min, 30 cycles of 94 °C for 30 s, annealing at 59 °C for 30 s (Tab. 1), 72 °C for 1 min followed by a final incubation at 72 °C for 10 min. RT-PCR products were separated on a 1% agarose gel and stained with ethidium bromide.
Statistical analysis
Using data recorded on day 60, the relationship between the independent variables (localities, species, metal stresses) and dependent variables (GR or growing rate, NSP or symptomatology, NDP or plant survival) were compared using non-parametric statistical tests, due to the nature of the data (categorical variables and non-normal distributions). The Kruskal-Wallis H test was used to test for differences among groups, while the Mann-Whitney U test for the pairwise comparison between groups. Additionally, data were investigated using Canonical Variant Analysis (CVA) as described by Cankaya et al. ([13]), to determine the correlation between sets of variables from the same samples. According to Ter Braak ([38]), CVA is an ordination technique which impose the extra restriction that the axes be linear combinations of environmental variables. Using this approach, the community variation can be directly associated with the environmental variation, and environmental variables may be quantitative or nominal ([16]). The redundancy index is a measure of the variance of one set of variables predicted from the linear combination of the other set of variables. Pearson’s correlation is used to analyze the linear relationship between two sets of data. Enzyme activities of POX, SOD and CAT during the experimental course were analyzed by ANOVA.
Results
Metal stress symptoms
One-year-old plants of P. radiata, P. pinaster and P. canariensis from the contrasting localities of Llico and Huilquilemu were stressed under increasing concentrations of CuSO4 (100 mM, 200 mM, 300 mM) and AlCl3 (50 mM, 100 mM). Tests were conducted under ex vitro, controlled conditions, where a protective mesh (25 m x 25 m) was used as a closed chamber to maintain the homogeneity of variable incidences associated with evapotranspiration, and to reduce possible spatial effects. After one week of stress treatments, phenotypic changes were consistently visible in the non-tolerant plants. Symptoms started from the basal part of the seedlings, and migrated to the upper fraction during the experiment. At the beginning of induced stress with either CuSO4 or AlCl3, the leaves were a pale yellow color, which after 60 days turned into a mixture of opaque gray and shades of red. Tolerant plants preserved the green color of leaves; however, after 60 days some of the leaves showed non-lethal symptoms in their basal and mid sections.
P. pinaster showed the best results under both 200 mM CuSO4 and 300 mM CuSO4 (Fig. 1), while both P. pinaster and P. canariensis showed tolerance to 100 mM AlCl3 (Fig. 2). P. radiata had intense foliar damage, which evolved to plant death in a significant number of individuals. Contrastingly, control treatments (three pine species from each site) immersed in distilled water remained with an intense green color, indicating the optimal physiological quality of the starting materials and their expected behavior during the experimental time (data not shown).
Fig. 1 - General symptoms of pine plants under abiotic stress from CuSO4 treatment in controlled ex vitro conditions. (A): P. radiata ; (B): P. canariensis; (C): P. pinaster.
Fig. 2 - Details of symptoms in pine plants under AlCl3 treatment in controlled ex vitro conditions. (A): P. radiata; (B): P. canariensis; (C): P. pinaster; (I): apical zone; (II): basal zone.
Seedling growth and survival
The characterization of metal tolerance was estimated by the NDP and NSP after 60 days of stress. Considering the most consistent phenotypic contrast between the tolerant and non-tolerant plants, only seedlings grown under 300 mM CuSO4 and 100 mM AlCl3 treatments were selected for further experiments and data analysis.
Differences in the number of tolerant plants (NTP) were verified between Pinus spp., where P. pinaster displayed the highest NTP values (57.3% of individuals tolerant to CuSO4 or AlCl3). However, a remarkable influence of the seed provenance was detected (Tab. 2), being plantlets germinated from seeds collected in Llico more tolerant to metal stresses than those from Huilquilemu (87.4% vs. 12.6%, respectively).
Tab. 2 - Tolerance to metal stresses of Pinus spp. seedlings from the provenances Llico and Huilquilemu after 60 days. (NSP): number of symptomatic plants; (NDP): number of dead plants; (NTP): number of tolerant plants.
Locality / metal stress / variable |
Llico | Huilquilemu | Tolerant plants |
||||||||||
---|---|---|---|---|---|---|---|---|---|---|---|---|---|
CuSO4 (300 mM) | AlCl3 (100 mM) | CuSO4 (300 mM) | AlCl3 (100 mM) | ||||||||||
NSP | NDP | NTP | NSP | NDP | NTP | NSP | NDP | NTP | NSP | NDP | NTP | ||
P. radiata | 96 | 76 | 20 | 96 | 63 | 33 | 96 | 91 | 5 | 96 | 92 | 4 | 62 (17.9%) |
P. canariensis | 96 | 80 | 16 | 58 | 36 | 60 | 96 | 89 | 7 | 96 | 93 | 3 | 86 (24.8%) |
P. pinaster | 84 | 6 | 90 | 57 | 12 | 84 | 96 | 82 | 14 | 96 | 85 | 11 | 199 (57.3%) |
Tolerant plants/treatment | - | - | 126 | - | - | 177 | - | - | 26 | - | - | 18 | 347 (100%) |
Tolerant plants/locality | 303 (87.4%) | 44 (12.6%) | 347 (100%) |
Using exploratory univariate statistical analysis (Mann-Whitney U test), significant differences were found in symptomatology and plant survival in relation to the site and the pine species (Tab. 3). No significant differences in growth rate were detected between P. radiata and P. canariensis, while they both differed from P. pinaster, which had the highest values. Seedlings from Llico showed the highest growth rate and displayed significant differences in comparison with seedlings of mother plants from Huilquilemu.
Tab. 3 - Exploratory univariate analysis for different groups of variables. Different letters in the same column indicate significant differences between the means after Mann-Whitney U test (p<0.05).
Group | Variable | Mean | ||
---|---|---|---|---|
Growth rate (cm) | Symptomatology (scale 1 to 4) |
Plant survival |
||
Location | Llico | 0.772 a | 3.58 a | 0.20 a |
Huilquilemu | 0.241 b | 3.86 b | 0.06 b | |
Species | P. radiata | 0.204 b | 3.87 c | 0.06 c |
P. canariensis | 0.314 b | 3.78 b | 0.10 b | |
P. pinaster | 0.923 a | 3.50 a | 0.23 a | |
Metal stress | 300mM CuSO4 | 1.180 | 3.34 | 0.31 |
100mM AlCl3 | 1.241 | 3.26 | 0.35 |
A time course of the experiments with seedlings from the Llico provenance was estimated (Fig. 3). P. pinaster showed the best results with the lowest number of dead plants under either 300 mM CuSO4 or 100 mM AlCl3 treatments. Overall, the NSP was higher than the NDP, depending on the species and metal stress treatments.
Fig. 3 - Tolerance of pine seedlings (Llico provenance) to CuSO4 (a-b) and AlCl3 (c-d) treatments under controlled conditions. (a) Number of symptomatic plants (NSP) at 300 mM CuSO4; (b): number of dead plants (NDP) at 300 mM CuSO4; (c): NSP at 100 mM AlCl3; (d): NDP at 100 mM AlCl3.
Canonical variant analysis
Canonical variant analysis (CVA) was applied to test for correlations between experimental factors and response variables. To set the canonical functions, three independent variables (i.e., locality, Pinus species and metal stress) were recognized as group 1 (genotype-environment interaction), while the three dependent variables (i.e., growth rate, symptomatology and plant survival) were referred to as group 2 (tolerance to metal stress).
The Pearson’s correlation analysis between the CVA dimensions of groups 1 and 2 revealed low though significant correlation coefficients (Tab. 4). The highest correlations were detected between metal stress and tolerance variables, with a maximum value of 0.295 between plant survival and metal stress.
Tab. 4 - Matrix of correlations between variables of groups 1 (locality, species and metal stress) and 2 (growth rate, symptomatology and plant survival). (***): p<0.001.
Response variables | Factors | ||
---|---|---|---|
Locality | Species | Metal stress | |
Growth rate | -0.181076*** | 0.231419*** | 0.275085*** |
Symptomatology | 0.186185*** | -0.196181*** | -0.274277*** |
Plant survival | -0.211238*** | 0.202894*** | 0.295173*** |
Canonical functions reflect the correlations between latent variables in their possible combinations (Tab. 5). Since there were three variables as original criteria, only three canonical functions were extracted: (1) locality-species-metal stress-growing rate; (2) locality-species-metal stress-symptomatology; and (3) locality-species-metal stress-plant survival. Results indicated a significant (p <0.0001) association between these groups for functions 1 and 2, and a high degree of correlation (r = 0.417) for function 1.
Tab. 5 - Significance and redundancy index of canonical functions.
Function | R canonical | Square | χ2 | dg | p-value | Prop. of variance | Redundancy |
---|---|---|---|---|---|---|---|
1 | 0.417079 | 0.17396 | 304.0054 | 9 | <0.0001 | 0.923265 | 0.160606 |
2 | 0.142656 | 0.02035 | 29.8647 | 4 | <0.0001 | 0.022106 | 0.000450 |
3 | 0.016064 | 0.00026 | 0.3702 | 1 | 0.5429 | 0.054629 | 0.000014 |
As for function 1, the proportion of variance of the metal stress tolerance variables (set 2) explained by the genotype-environment interaction variables (set 1) was 0.173 (Rcanonical = 0.417), while it was 0.020 (Rcanonical = 0.143) for the canonical function 2 (Tab. 5).
The redundancy index is a measure of the ability of original variables (locality, species and metal stress) taken together, to explain the variation in the criterion variables (namely, growing rate, symptomatology and plant survival). Canonical correlation weights (i.e., the impact of each variable on the canonical function) demonstrated that function 1 was mainly influenced by plant survival, metal stress, species and locality variables, while function 2 was mostly affected by the species, symptomatology and locality (Tab. 6).
Tab. 6 - Correlations between canonical functions and the original variables.
Kind | Variables | Canonical functions | ||
---|---|---|---|---|
1 | 2 | 3 | ||
Factors | Locality | 0.498843 | 0.50699 | -0.702936 |
Species | -0.505099 | 0.82943 | 0.238567 | |
Metal stress | -0.704108 | -0.23454 | -0.670241 | |
Response variables | Growing rate | -0.245276 | 0.45572 | 0.843858 |
Symptomatology | -0.224864 | 0.63288 | 0.198845 | |
Plant survival | -0.975096 | -0.44654 | 0.149508 |
Enzyme activity
In the case of seedlings from the Llico provenance, the enzyme activity of POX, SOD and CAT was determined after 60 days since the beginning of the experiment. For 300 mM CuSO4 and 100 mM AlCl3 treatments, randomized samples were selected from the middle parts of five plants per treatment. For P. pinaster seedlings, POX activity increased in both treatments, while it decreased drastically in P. canariensis and P. radiata. In the case of SOD, enzyme activity was higher in P. pinaster than in P. canariensis and P. radiata. For all the three pine species, SOD activity in seedlings grown under AlCl3 treatment increased during the first 30 days, whereas it severely decreased in P. radiata plants after 60 days (Tab. 7).
Tab. 7 - Time course of POX, SOD and CAT enzyme activities [in U (mg protein)-1] in pine seedlings from the Llico provenance, treated with 300 mM CuSO4 or 100 mM AlCl3. Values are means ± standard deviation of three determinations per replica. Different letters in the same column indicate significant differences between the means after ANOVA (p<0.05).
Treatment | Enzyme | Species | TO | 15 Days | 30 Days | 45 Days | 60 Days |
---|---|---|---|---|---|---|---|
300 mM CuSO4 | POX | P. pinaster | 1.30 ± 0.51a | 1.48 ± 0.43a | 1.52 ± 0.62a | 1.53 ± 0.41a | 1.53 ± 0.45a |
P. canariensis | 0.81 ± 0.23b | 0.62 ± 0.13b | 0.42 ± 0.19b | 0.23 ± 0.11b | 0.08 ± 0.03b | ||
P. radiata | 0.48 ± 0.11c | 0.32 ± 0.09c | 0.25 ± 0.14b | 0.24 ± 0.15b | 0.07 ± 0.03b | ||
SOD | P. pinaster | 0.42 ± 0.12a | 0.44 ± 0.21a | 0.45 ± 0.11a | 0.45 ± 0.16a | 0.44 ± 0.15a | |
P. canariensis | 0.43 ± 0.11a | 0.39 ± 0.16a | 0.35 ± 0.08b | 0.33 ± 0.09b | 0.25 ± 0.10b | ||
P. radiata | 0.35 ± 0.10b | 0.27 ± 0.13b | 0.20 ± 0.11c | 0.12 ± 0.04c | 0.09 ± 0.03c | ||
CAT | P. pinaster | 1.22 ± 0.34a | 1.21 ± 0.29a | 1.20 ± 0.21a | 1.20 ± 0.35a | 1.19 ± 0.31a | |
P. canariensis | 1.25 ± 0.26a | 1.27 ± 0.31a | 1.21 ± 0.34a | 1.12 ± 0.22a | 1.05 ± 0.19a | ||
P. radiata | 1.24 ± 0.30a | 1.22 ± 0.36a | 1.11 ± 0.36a | 0.73 ± 0.11b | 0.62 ± 0.15b | ||
100 mM AlCl3 | POX | P. pinaster | 0.72 ± 0.21a | 0.84 ± 0.17a | 0.85 ± 0.24a | 0.86 ± 0.29a | 0.86 ± 0.31a |
P. canariensis | 0.69 ± 0.17a | 0.65 ± 0.15b | 0.42 ± 0.19b | 0.25 ± 0.12b | 0.15 ± 0.06b | ||
P. radiata | 0.65 ± 0.20a | 0.49 ± 0.11b | 0.28 ± 0.14b | 0.22 ± 0.09b | 0.13 ± 0.05b | ||
SOD | P. pinaster | 0.31 ± 0.13a | 0.43 ± 0.14a | 0.42 ± 0.10a | 0.40 ± 0.18a | 0.39 ± 0.10a | |
P. canariensis | 0.30 ± 0.11a | 0.35 ± 0.19ab | 0.34 ± 0.07b | 0.31 ± 0.08b | 0.26 ± 0.12b | ||
P. radiata | 0.26 ± 0.12a | 0.27 ± 0.16b | 0.21 ± 0.11bc | 0.18 ± 0.09c | 0.10 ± 0.03c | ||
CAT | P. pinaster | 0.85 ± 0.19b | 1.12 ± 0.22a | 1.09 ± 0.31a | 1.08 ± 0.21a | 1.07 ± 0.23a | |
P. canariensis | 1.33 ± 0.19a | 1.32 ± 0.21a | 1.30 ± 0.27a | 0.75 ± 0.20b | 0.46 ± 0.16b | ||
P. radiata | 0.83 ± 0.22b | 0.67 ± 0.15b | 0.59 ± 0.18b | 0.38 ± 0.13c | 0.23 ± 0.09c |
Additionally, CAT activity showed the highest values for P. canariensis after 15 (300 mM CuSO4) and 30 days (100 mM AlCl3); however, these enzymatic values decreased during the experiment. At the end of the experiments (60 days), CAT activity was highest in P. pinaster seedlings in both stress treatments. Overall, P. pinaster plants showed the highest level of enzyme activity, followed by P. canariensis (intermediate) and P. radiata, which showed the lowest enzymatic values.
RT-PCR Analysis
Transcriptional activities of Cu-Zn-superoxide dismutase and Ribulose bisphosphate carboxylase (RuBisCo) were measured by semi-quantitative RT-PCRs of selected individuals. Differential gene expression analysis was conducted in pine plants treated with 300 mM CuSO4. P. radiata showed transcript expression only at 15 days for Cu-Zn-superoxide dismutase and at 30 days in the case of RuBisCo (Fig. 4), while P. canariensis seedlings showed gene expression in both cases at 30 days. Remarkably, consistent expression of the studied genes was demonstrated in P. pinasters during stress treatments (60 days). This result was in agreement with the lowest number of dead plants in this pine species.
Discussion
The present study investigated about the tolerance of Pinus spp. seedlings to abiotic stress (CuSO4 and AlCl3) in ex vitro controlled conditions. The interaction between different metal stresses was not considered here, because it is known that tolerances to Cu and Al are induced by separate genetic mechanisms ([15], [37]), and the probability of finding tolerant individuals to combined stress of CuSO4/AlCl3 would be very low. On the other hand, the highest concentration of salts (i.e., 300 mM CuSO4 and 100 mM AlCl3) was applied for both the statistical and molecular analysis.
Our results showed that a higher number of tolerant seedlings originated from seeds collected from mother trees growing in a more severe environment. Indeed, the number of metal-tolerant seedlings obtained from the Llico provenance was largely higher (87.4%) than those from Huilquilemu (12.6%). Both these sites belong to the same region (Maule, Chile), but are separated by the coastal mountain range which determines highly contrasting environmental conditions between the two sites in terms of salt exposure, rain regime, soil type, etc. Moreover, Llico is a secular agroforestry system located along the coast, where pines were planted as protection barriers, while Huilquilemu is an experimental field where germplasm of different plant species is under maintenance and characterization.
The identification of the environmental factors underlying the higher metal tolerance of pine seedlings from the Llico provenance is out of the scope of this study. However, our results support the hypothesis that plant phenotype depends on genotype-environment interactions, which are specific for the area where they grow, but at the same time it could also be determined by the environment experienced by the parent trees ([11]). Indeed, the canonical variate analysis (CVA) carried out in this study seems to support the existence of a maternal effect on seedling tolerance to Cu and Al, independently from the species considered. CVA has also been used to study changes in phenolic concentrations in peach over time ([3]), as well the susceptibility to disease in monocot species and their effect on pathogen inoculum ([9]).
Abiotic stress in the maternal environment has been reported to induce a “transgenerational plasticity” which could affect progeny performances ([26]). Trans-generational plastic responses to the maternal environment are transmitted to offspring phenotypes without any change in DNA ([17]). As a source of phenotypic variation, maternal environmental effects can influence the evolutionary process and the population dynamics of many plant species ([22], [26]). Furthermore, increasing evidence suggests that transgenerational plasticity could be adaptive, enhancing offspring fitness under environments similar to that of the maternal environment ([23]). It has also been proposed to exploit such maternal environmental effects to improve the performance of tree plantations by exposing mother trees to appropriate environmental conditions ([39]). The maternal environment is known to influence many traits, such as seed traits ([40]), germination ([17]), and seedling performance ([19]) in different species, including long-lived plants such as conifers ([39]).
The influence of different genetic backgrounds on the tolerance to heavy metals in plants has been widely discussed ([5], [33]). Crepidorhopalon perennis and C. tenuis (Linderniaceae), two metallophyte herb species from South Central Africa, have been used as models to investigate the relationship between the degree of tolerance of plants populations, bioavailable Cu content in the soil and the ecological isolation of endemic species ([20]). Regarding forest species, resistant trees of both Pinus sylvestris L. and Pinus nigra Arn. have been reported to exhibit a lower degree of genetic variation than metal-sensitive trees with respect to some isozyme loci (SHDH A, PGI, PGM, MDH C and DIA). This allele depletion suggests that genetic changes induced by heavy metals may dramatically affect the adaptation process in forest tree populations ([14]).
In this study, different activities of the enzymes POX, SOD and CAT were found among the three pine species from the Llico provenance, with P. pinaster showing the highest values. Moreover, the expression of genes coding for Cu-Zn-superoxide dismutase and Ribulose bisphosphate carboxylase (RuBisCo) was the highest in P. pinaster after 60-days metal treatments.
The protective functions of antioxidants from heavy metal stress have been reviewed by Schützendübel & Polle ([35]). Based on their chemical and physical properties, different molecular mechanisms of heavy metal toxicity can be distinguished: (1) production of ROS by autoxidation and the Fenton reaction, which is typical for transition metals such as iron or copper; (2) blocking of essential functional groups in biomolecules, which has been reported for non-redox reactive heavy metals such as cadmium and mercury; (3) displacement of essential metal ions from biomolecules, a reaction occurring with different kinds of heavy metals. At the plant cell level, SODs represent the first line of defense against ROS. Ozone is produced at any location where an electron transport chain is present, and O2 activation may occur in different compartments of the cell, including mitochondria, chloroplasts, microsomes, glyoxysomes, peroxisomes, apoplasts, and the cytosol ([18], [2]).
Photosynthetic activity and oxidative stress are considered sensitive biological indicators of heavy metal stress ([29], [35]). The RT-PCR analysis carried out in this study revealed a conspicuous expression of genes coding for Cu-Zn-superoxide dismutase and RuBisCo in P. pinaster (Fig. 4). These results are fairly consistent with the high levels of metal tolerance observed in P. pinaster, while P. canariensis and P. radiata showed intermediate and low tolerance, respectively, according to their low expression of the aforementioned genes.
The results of this study are in agreement with those reported by Acquaviva et al. ([1]), who demonstrated a significant differential expression of Heat shock protein 70, Hemeoxygenase and Superoxide dismutase in P. pinaster trees from two sites with contrasting pollution levels. These authors proposed that the increased expression of the above enzymes may exert protective effects against oxidative stress and represent an adaptative defense mechanism. Moreover, these genes could represent useful tools for monitoring the environmental contamination and better understand the mechanisms underlying plant defense to stress. Furthermore, the response of Camellia sinensis (L.) O. Kuntze to Cu and Al stresses was investigated by Yadav & Mohanpuria ([42]). Exposure to 100 µM CuSO4 or 100 µM AlCl3 led to accumulation of higher levels of ROS in the Assamica compared to the Chinary genotype. Proline content was higher in Chinary compared to Assamica, while chlorophyll and protein contents decreased upon Cu and Al exposure in both varieties. Phytochelatin synthase (PCS), an enzyme involved in phytochelatin synthesis by using glutathione as a substrate, was up-regulated to the highest levels in Chinary. These results suggest that the Chinary could be more Cu- and Al-tolerant than the Assamica genotype.
In conclusion, P. pinaster has shown the best tolerance to both Cu and Al under controlled conditions in terms of plant survival and detoxifying enzyme activity. Tolerant individuals of all the species studied have been selected and planted in a local mining ecosystem, which is particularly vulnerable to heavy metal pollution. Epigenetic analysis will be conducted in selected individuals in future studies.
Acknowledgements
The authors thank Science Editing Chile for language revision and copy-editing of the manuscript. This project was financed by the Catholic University of Maule (UCM) and the Regional Government of Maule, Chile, Project BIP 30.386.978-0.
References
Gscholar
Gscholar
Gscholar
Gscholar
Gscholar
Gscholar
CrossRef | Gscholar
Authors’ Info
Authors’ Affiliation
Claudio Rodríguez
Lisandro Roco
Carolina Vergara
Nataly González-Soto
Rolando García-González
Center for Biotechnology of Natural Resources, Department of Forestry, Faculty of Agronomy and Forestry Sciences, Catholic University of Maule, av.da San Miguel 3605, Talca (Chile)
Corresponding author
Paper Info
Citation
Arencibia AD, Rodríguez C, Roco L, Vergara C, González-Soto N, García-González R (2016). Tolerance to heavy metal stress in seedlings of three pine species from contrasting environmental conditions in Chile. iForest 9: 937-945. - doi: 10.3832/ifor1908-009
Academic Editor
Claudia Cocozza
Paper history
Received: Nov 02, 2015
Accepted: May 02, 2016
First online: Aug 12, 2016
Publication Date: Dec 14, 2016
Publication Time: 3.40 months
Copyright Information
© SISEF - The Italian Society of Silviculture and Forest Ecology 2016
Open Access
This article is distributed under the terms of the Creative Commons Attribution-Non Commercial 4.0 International (https://creativecommons.org/licenses/by-nc/4.0/), which permits unrestricted use, distribution, and reproduction in any medium, provided you give appropriate credit to the original author(s) and the source, provide a link to the Creative Commons license, and indicate if changes were made.
Web Metrics
Breakdown by View Type
Article Usage
Total Article Views: 45747
(from publication date up to now)
Breakdown by View Type
HTML Page Views: 40026
Abstract Page Views: 1945
PDF Downloads: 2820
Citation/Reference Downloads: 46
XML Downloads: 910
Web Metrics
Days since publication: 3023
Overall contacts: 45747
Avg. contacts per week: 105.93
Article Citations
Article citations are based on data periodically collected from the Clarivate Web of Science web site
(last update: Feb 2023)
Total number of cites (since 2016): 3
Average cites per year: 0.38
Publication Metrics
by Dimensions ©
Articles citing this article
List of the papers citing this article based on CrossRef Cited-by.
Related Contents
iForest Similar Articles
Research Articles
Variation of major elements and heavy metals occurrence in hybrid aspen (Populus tremuloides Michx. × P. tremula L.) tree rings in marginal land
vol. 13, pp. 24-32 (online: 15 January 2020)
Short Communications
A quick screening to assess the phytoextraction potential of cadmium and copper in Quercus pubescens plantlets
vol. 10, pp. 93-98 (online: 13 October 2016)
Research Articles
Heavy metal (Zn, Pb, Cd) concentration in soil and moss (Pleurozium schreberii) in the Brynica district, southern Poland
vol. 4, pp. 176-180 (online: 11 August 2011)
Review Papers
Heavy metals and woody plants - biotechnologies for phytoremediation
vol. 4, pp. 7-15 (online: 27 January 2011)
Research Articles
Links between phenology and ecophysiology in a European beech forest
vol. 8, pp. 438-447 (online: 15 December 2014)
Research Articles
Can species Cedrela fissilis Vell. be used in sites contaminated with toxic aluminum and cadmium metals?
vol. 14, pp. 508-516 (online: 11 November 2021)
Review Papers
A review of the performance of woody and herbaceous ornamental plants for phytoremediation in urban areas
vol. 13, pp. 139-151 (online: 14 April 2020)
Research Articles
Effect of seedling stock on the early stand development and physiology of improved loblolly pine (Pinus taeda L.) seedlings
vol. 9, pp. 690-695 (online: 12 May 2016)
Research Articles
Preliminary results of the tolerance to inorganic contaminants and phytoextraction potential of twelve ornamental shrub species tested on an experimental contaminated site
vol. 11, pp. 442-448 (online: 18 June 2018)
Research Articles
Distribution and concentration of cadmium in root tissue of Populus alba determined by scanning electron microscopy and energy-dispersive x-ray microanalysis
vol. 1, pp. 96-103 (online: 20 May 2008)
iForest Database Search
Search By Author
Search By Keyword
Google Scholar Search
Citing Articles
Search By Author
Search By Keywords
PubMed Search
Search By Author
Search By Keyword