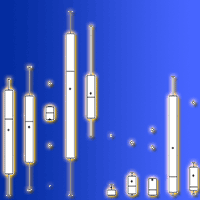
Use of brassinosteroids to overcome unfavourable climatic effects on seed germination in Pinus nigra J. F. Arnold
iForest - Biogeosciences and Forestry, Volume 17, Issue 1, Pages 1-9 (2024)
doi: https://doi.org/10.3832/ifor4340-016
Published: Feb 02, 2024 - Copyright © 2024 SISEF
Research Articles
Abstract
Seeds of forest species can show germination and survival problems if they are exposed to adverse climatic conditions in the course of germination. Brassinosteroids could help seeds to overcome such environmental stress. We tested the effects of exogenous application of a chosen brassinosteroid compound 2α,3α,17β-trihydroxy-5α-androstan-6-one on the germination capacity and germination energy of European black pine (Pinus nigra J. F. Arnold) seeds. Before germination, the seeds were soaked for 24 hours either in demineralised water (control treatment) or in brassinosteroid solutions of four concentrations (high, higher-medium, lower-medium and low-concentration treatments). In the course of germination, the control and all four concentration treatments were subjected to two alternative temperature regimes: the optimal (unstressed) regime (20/30°C) and the stress regime, during which the seeds were exposed to temporary temperature stress (peaking at 42°C). In the optimal temperature regime, the highest germination energy was recorded in the higher-medium-concentration treatment and a significantly increased germination energy when compared to the control was also observed in a high-concentration treatment. The brassinosteroid, when applied in high, higher-medium and lower-medium concentrations, significantly increased the germination capacity compared to the control. The highest germination capacity was recorded in the high-concentration treatment. The temperature stress substantially reduced the germination of P. nigra. In the stress regime, the seeds of the higher-medium-concentration treatment decidedly showed the highest germination energy and capacity and those of the control treatment the lowest. The seed germination energy in the control was significantly lower than that recorded in the high and higher-medium-concentration treatments. The seed germination capacity in the control was significantly lower than those found in all concentration treatments, except for the seeds in the low-concentration treatment. The brassinosteroid application promoted the germination of black pine and partly alleviated the impact of the temporary heat stress. However, this application did not compensate for the heat stress effects completely. The potential for the use of the brassinosteroid may exist chiefly for seedings of Pinus nigra in forest nurseries where the nursery staff try to maintain optimal climatic conditions, and deviations from these conditions are usually only temporary.
Keywords
Brassinosteroids, Pinus nigra, Germination Capacity, Germination Energy, Germination Rate
Introduction
Germination is a critical developmental transition period in the life cycle of plants ([51], [13]). Seed germination, or seedling emergence in general, is significantly more sensitive to adverse ambient conditions than later stages of growth ([28]). More concretely, germination represents a period of maximum vulnerability to the physical changes in the environment and minimal potential for physiological retrenchment if some adverse conditions occur ([3]). Temperature is a critical factor regulating seed dormancy and influencing germination ([43]). When germination is initiated, extremely high (or low) temperatures can easily become a fatal stressor.
If direct seeding is used, the germinating seed can be easily exposed to high temperatures, especially on sites missing the canopy shelter of an older stand. Even in forest nurseries on open outdoor nursery beds, it is not always possible to fully control the temperature and heat stress may occur. Outdoor seedbeds in a nursery do not remain at constant temperatures but experience fluctuations ([6]). Outdoor seed-bed surfaces can become very hot in spring and summer ([35]). Their temperature can easily rise over 45 °C. It is worth mentioning that irrigation to cool the surface temperature begins in the 30-33 °C range in forest nurseries during germination and that the killing point of a stem temperature for germinants is about 40 °C ([35]).
Brassinosteroids (BR when referring to a particular steroid; BRs when denoting brassinosteroids in general) are steroidal plant hormones implicated in the furtherance of plant growth and development ([5]). These hormones influence the division, elongation and differentiation of various cell types of plants ([42]). Brassinosteroids are perceived at the cell surface ([39]). In contrast to animal steroid hormones that bind nuclear receptors directly to modulate gene expressions, BR receptors are kinases localised in the plasma membrane ([24]). BRs have a wide distribution throughout the plant kingdom and can cause biological effects on plant growth when applied exogenously ([31]), and exogenous application can reduce the harmful effects of heat ([47]) and other environmental stressors ([12], [14], [47]). Nawaz et al. ([37]) summarised the roles of BRs in improving plant tolerance against various environmental stressors. The application of BRs may improve water status, increase the content of pigments and proline, increase transpiration and photosynthetic rate, prevent photoinhibition, upregulate the stress-related transcription factors, reduce electrolyte leakage, malondialdehyde (MDA) content and lipid peroxidation and activate or promote antioxidant mechanisms. However, BR effects depend on numerous factors such as plant (tree) species, growth stage, growth conditions (with or without stress), kinds of stress, duration of stress and its crosstalk with other hormones, growth regulators and signalling molecules ([38], [39]). Moreover, plant growth and development are controlled by the BRs in a dosage-dependent manner ([57]).
The presupposition of this study was that a presowing application of the chosen brassinosteroid compound might improve the germination of seeds subjected to temperature stress.
The applied research on BRs was conducted mainly on agricultural plants ([51], [5], [7]). Until the first decade of the new millennium, research focused on the potential of BRs in forestry was rather sporadic and, with some exceptions (e.g., [46]), hardly any in Europe. Nonetheless, the studies from Asia and America showed promising effects of BRs on seed germination ([25], [26]), vegetative propagation ([45]) and early growth of trees ([27]). More recently, studies dealing with the effect of brassinosteroids on the growth of woody plants and their seeds were published in Central Europe. No demonstrable positive effect of BRs on the initial growth of young forest tree plantations under Central European conditions has been demonstrated ([40], [41], [15], [52]). However, some positive effects have been indicated for seeds of several species including BR-mediated alleviation of environmental stresses ([44], [23], [9]). Therefore, a gradual verification of the effects of BRs is needed on a wider spectrum of European forest tree species, which also takes into account the climatic extremes that Europe experienced in the previous decades ([29]).
A BR compound (2α,3α,17β-trihydroxy-5α-androstan-6-one) was synthesised in the Czech Republic (see Material and Methods for further details). Its production cost is lower than that of many natural BRs. This paper aims to assess the effects of this BR compound on the germination parameters of European black pine (Pinus nigra J. F. Arnold). The effects of this BR were investigated under conditions of an optimal temperature regime and temporary stress induced by a short-term heat spell timed in the early phase of the germination process.
The black pine was chosen for the experiment since the species is native to Southern Europe and parts of Central Europe ([49]), as well as in Turkey and parts of Central Asia ([4], [10]). The tree is widely used in forestry as its wood is durable and easy to process ([11]). Black pine can grow on a variety of soils with different chemistry ([16]), in dry as well as humid habitats ([33], [49]), which also enables its use for forest restoration in degraded habitats ([56], [4]). In the scenario of a shift towards a warmer and more arid climate in Central Europe, black pine could represent a component for tree species composition or diversification of forests even in areas further north than its natural distribution today.
Material and methods
The research was conducted from January to February 2015 in the Truba laboratory under the Department of Silviculture, Faculty of Forestry and Wood Sciences of the Czech University of Life Sciences, Prague, Czech Republic.
For this research, the European black pine (Pinus nigra) was chosen, and the effect of brassinosteroid 2α,3α,17β-trihydroxy-5α-androstan-6-one ([22]) was tested under the conditions specified further in the text. The chemical compound used was synthesised by PHPchem Co. Ltd. (Sulovice, Czech Republic) and provided in a concentrated solution containing 1 g of the brassinosteroid, 30 ml of ethanol and 1 ml of Tween (Polysorbate) 20 made up to 1000 ml with demineralised water.
The seed material originated from Western Slovenia. The cones matured in the autumn of 2011 and were collected in the winter of 2011/2012. Seed extraction and cleaning were done in the spring of 2012. From the spring of 2012 to the spring of 2013, the seed was stored in plastic bags at -7 °C in the Netherlands at a company that organised the collection. From March 2013 to June 2014 the seed was kept in a cold, air-conditioned store of a Czech importer (Forests of the Czech Republic, state enterprise) at 2.0-3.5 °C. From June 2014 to the start of the experiment, the seed was stored in plastic bags at 8 °C in the Truba laboratory. The purity of the seed lot and thousand-seed weight, determined according to the International Rules for Seed Testing ([18]), was 99.9% and 21.920 g, respectively.
Altogether, 10.000 seeds were examined under controlled conditions. The seeds of four BR treatments were soaked in BR solutions of four concentrations. The concentrations of BR solutions were categorised as high (H) = 0.4 mg l-1, higher-medium (HM) = 0.04 mg l-1, lower-medium (LM) = 0.02 mg l-1, and low (L) = 0.004 mg l-1, covering the spectrum of concentrations in which the value with optimum efficiency could likely be found, according to some literature sources ([25], [26]). Control (C) seeds were only soaked in demineralised water. The soaking lasted 24 hours for all treatments.
Each treatment contained 2.000 seeds divided into 20 vessels (containers). Every container included 100 seeds. All treatments, including control, were exposed to two germination regimes. Half of the seeds (10 containers for each treatment) were subjected to temporary heat stress (S), and the other half was kept under optimal temperature not exceeding 30 °C (O). Altogether, the entire research included ten different treatment-regime combinations, and each of these combinations included 1.000 seeds divided into ten replications (Tab. 1).
Tab. 1 - Design of the experiment. In the heat-stress regime, the seeds were subjected to temporary heat stress with a maximum temperature of 42 °C. This highest temperature lasted for two hours, see further text.
Treatment | BR concentration (mg l-1) |
Regime | Treatment-regime combination | Code | Number of replications |
Number of seeds |
---|---|---|---|---|---|---|
Control | 0.000 | optimal | control + optimal temperature | CO | 10 | 1000 |
heat-stress | control + heat stress | CS | 10 | 1000 | ||
Low | 0.004 | optimal | low + optimal temperature | LO | 10 | 1000 |
heat-stress | low + heat stress | LS | 10 | 1000 | ||
Lower- medium |
0.020 | optimal | lower-medium + optimal temperature | LMO | 10 | 1000 |
heat-stress | lower-medium + heat stress | LMS | 10 | 1000 | ||
Higher-medium | 0.040 | optimal | higher-medium + optimal temperature | HMO | 10 | 1000 |
heat-stress | higher-medium + heat stress | HMS | 10 | 1000 | ||
High | 0.400 | optimal | high + optimal temperature | HO | 10 | 1000 |
heat-stress | high + heat-stress | HS | 10 | 1000 |
Preparation of the experiment
We prepared slightly more than 10.000 seeds for the study according to the thousand-seed weight, washed them in demineralised water and removed all debris. Pure seeds were sterilised in sodium hypochlorite (1% solution) for 10 minutes and then thoroughly rinsed. The seed lot was then separated into five equal groups (treatments) that were immersed either in the demineralised water (control) or in the soaking solutions of the particular BR concentrations, as they are delineated in Tab. 1, for 24 hrs at room temperature (20 °C).
The seeds were germinated on three layers of filter paper (50 g m-2) soaked in demineralised water in transparent plastic vessels 6.5 × 9 × 3.5 cm in size (100 seeds/vessel). The seeds did not touch each other. The vessels were covered with translucent plastic lids protecting the seeds while keeping high moisture inside. The relative air humidity ranged between 90-92% inside the vessels (measured with sensors inside chosen sample vessels).
The germination was conducted in two growth chambers, POL-EKO KK 700 (Pol-Eko-Aparatura sp.j., Wodzislaw Slaski, Poland), for 28 days with alternating temperatures of 20/30 °C (dark/light) and eight hours of illumination per day. Illumination at an intensity of 11.200-13.050 lx was provided using cool white fluorescent tubes. For the heat-stress regime, a temporary illuminated heat-stress segment was introduced in one of the growth chambers. The heat-stress event began 128 hours after the start of germination. The heat-stress segment lasted 10 hours and peaked at 42 °C (see Fig. 1 for more detail) approximately mimicking the heat-stress conditions inside the topsoil of an unsheltered site on a sunny spring day.
Fig. 1 - Temperature-time profile of a temporary heat-stress segment inserted into the heat-stress regime 128 hours after the start of the germination programme (diagram by L. Rostislav).
The illuminated heat-stress segment thus replaced the typical segment with light and a temperature of 30 °C within the sixth 24-hour cycle. At the end of the 10-hour heat-stress segment, the temperature was stabilized at 20 °C without illumination. The length of the non-illuminated segment (at 20 °C) immediately following the heat-stress segment was, therefore, shorter, keeping the timing of the 24-hour cycles the same in both chambers throughout the experiment.
It is worth noting that with only two growth (incubation) chambers, one for each regime, the experiment had no actual replicates. This represents some limitations of the experimental design. On the other hand, the temperature and light conditions were monitored independently by built-in and external sensors (dataloggers), which showed that the set parameters of the experiment were maintained in both growth chambers.
Evaluation of seed germination
Germinants were counted at weekly intervals (days 7, 14, 21 and 28) and removed from the vessels. The seeds were classified as completely germinated when their primary root and hypocotyl together exceeded four times the length of the seed ([18]). The rotten (apparently deceased) seeds were also counted and removed to prevent the spreading of potential infection. The final numbers of empty and diseased seeds were determined at the end of the germination test by cutting open non-germinated seeds. For our study, germination energy is defined as the percentage of germinated seeds on day 7 (inclusive) of the germination test. In compliance with the ISTA ([18]) standards, the germination capacity was viewed as the cumulative percentage of seeds germinated by day 21 (inclusive) of the germination test. The subsequent counting of germinants (day 28) was conducted to check whether the chosen length of the germination test was sufficient.
The seed germination rate (GR) was calculated for each treatment-regime combination as the sum of values obtained by dividing the weekly percentages of completely germinated filled seeds by the number of days the seeds had been left in the growth chamber, using the following formula (adapted from [30] - eqn. 1):
where n is the week of counting, GPn is the current (not cumulative) percentage of completely germinated seeds in the n-th week, Δtn is the number of days elapsed from the beginning of the experiment (7, 14 and 21 days).
Germinant size refers to the overall length of a seedling recorded as completely germinated (see above) at one of the times of counting. This length included the radicle, hypocotyl and cotyledons and was measured to the nearest 1 mm.
Analysis of data
Multiple comparisons for binomially distributed data ([1]) were used for the analyses of germination capacity and energy. The Kruskal-Wallis test with multiple comparisons was used for assessing the radicular length and germination rates since the character of the data did not meet the assumptions for parametric two-way ANOVA. Multiple post-hoc comparisons of mean ranks of all pairs of groups according to Siegel & Castellan ([50]) were used. The chosen significance level α for all the conducted analyses was 0.05.
Results
Germination energy
In the optimal regime, germination energy (percentage of completely germinated seeds on day 7 of the germination test) was positively influenced in the treatments with higher-medium (HMO) and high (HO) concentrations of the applied BR. HMO showed the highest germination energy and significantly differed (p-values < 0.001) from all other treatments. The seeds in the control (CO), low (LO) and lower-medium (LMO) treatments showed similar values of germination energy (significantly lower than those recorded in HMO and HO) and did not differ from each other in this parameter (Fig. 2).
Fig. 2 - Germination energy. Cumulative percentage of germinated black pine on day 7. Error bars depict the 95% confidence intervals. Different letters above the bars denote significant (p<0.05) differences between the particular treatment-regime combinations. (CO): control + optimal conditions; (LO): low concentration + optimal conditions; (LMO): lower-medium concentration + optimal conditions; (HMO): higher-medium concentration + optimal conditions; (HO): high concentration + optimal conditions; (CS): control + heat stress; (LS): low concentration + heat stress; (LMS): lower-medium concentration + heat stress; (HMS): higher-medium concentration + heat stress; (HS): high concentration + heat stress.
In the treatments of the heat-stress regime, the values of germination energy were significantly lower than those of the optimal (unstressed) regime except for the higher-medium treatment (HMS). HMS showed comparable germination energy to those recorded in several unstressed treatments (CO, LO, LMO). BR application seems to promote germination in the heat-stress regime in comparison to the respective control (CS), however, a significant positive effect was documented in HMS and HS only (Fig. 2).
Germination capacity
For our study, the germination capacity is viewed as a cumulative percentage of completely germinated seeds on day 21. Under the optimal regime (Fig. 3), no significant difference in germination capacity was found between the control (CO) and low concentration (LO - p-value = 0.999). However, the other concentrations of the tested BR in the absence of heat stress (LMO, HMO, HO) showed significantly higher values of germination capacity than the control (CO). The differences in germination between the control (CO) and high-concentration (HO) treatment and between the control (CO) and higher-medium-concentration (HMO) treatment were even highly significant (p-value < 0.001).
Fig. 3 - Germination capacity. Cumulative percentage of germinated black pine seeds on day 21. Error bars depict the 95% confidence intervals. Different letters above the bars denote significant (p<0.05) differences between the particular treatment-regime combinations. (CO): control + optimal conditions; (LO): low concentration + optimal conditions; (LMO): lower-medium concentration + optimal conditions; (HMO): higher-medium concentration + optimal conditions; (HO): high concentration + optimal conditions; (CS): control + heat stress; (LS): low concentration + heat stress; (LMS): lower-medium concentration + heat stress; (HMS): higher-medium concentration + heat stress; (HS): high concentration + heat stress.
In the absence of heat stress, the comparison of germination capacity in the low (LO) and lower-medium concentration (LMO) of BR did not show any significant difference (p-value = 0.232), despite the germination capacity in LMO being higher than that in LO. As was the case of the control (CO), the low-concentration treatment (LO) showed significantly lower germination capacity when compared with the higher-medium-concentration (HMO) and high-concentration (HO) treatments under the unstressed regime. Both these comparisons showed highly significant differences (p-value = 0.001 and < 0.001, respectively). No significant difference (p-value = 0.821) in germination capacity emerged between the lower-medium (LMO) and higher-medium (HMO) concentrations under the unstressed regime. However, the lower-medium (LMO) and higher-medium (HMO) concentrations showed significantly lower germination capacities than the high-concentration treatment (HO). The p-value in both cases was < 0.001.
Under the heat-stress regime, there was no significant difference between the control (CS) and low-concentration (LS) treatments in germination capacity (p-value = 0.317). However, the seeds in the control (CS) showed significantly lower germination capacity than the seeds in all the other BR treatments (LMS, HMS and HS). When comparing the low concentration (LS) with the lower-medium (LMS) and high (HS) concentrations, no significant differences were found under the heat-stress regime (p-values = 0.665 and 0.314, respectively). Highly significant differences in germination capacity under the heat-stress regime were obtained if the higher-medium concentration (HMS) was compared with the low (LS), lower-medium (LMS) and high (HS) concentrations.
In general, the germination capacity values under the optimal as well as heat-stress regimes showed an increasing, positive trend with increasing BR concentration, except for high concentration in the heat-stress regime (Fig. 3).
Seed germination rate
The values of seed germination rate provide a similar pattern as the germination capacity. The seed germination rate was visibly lowered in the treatments of the heat-stress regime. In this stress regime, the medians ranged between 0 and 1 and the means between 0.39 and 2.35, while in the optimal regime, the medians ranged between 3.7 and 6.2 and the means between 3.3 and 5.3. The effect of BR application was detectable to some extent in HMO and HMS. However, the statistical analyses did not classify most of the differences visible in the graph as significant (Fig. 4).
Fig. 4 - Germination rate. (CO): control + optimal conditions; (LO): low concentration + optimal conditions; (LMO): lower-medium concentration + optimal conditions; (HMO): higher-medium concentration + optimal conditions; (HO): high concentration + optimal conditions; (CS): control + heat stress; (LS): low concentration + heat stress; (LMS): lower-medium concentration + heat stress; (HMS): higher-medium concentration + heat stress; (HS): high concentration + heat stress. Different letters below the box plots denote significant (p<0.05) differences within particular treatment-regime combinations.
Germinant size
The completely germinated individuals (see “Materials and Methods”) that were found on day 7 from the start of the germination test showed comparable average size in the following seven out of ten treatment-regime combinations: CO, LO, LMO, CS, LS, LMS, HS (Fig. 5). The medians of the above-listed treatment-regime combinations ranged between 25 and 30 mm, and these seven combinations did not differ significantly from each other. HMO, HO and HMS showed higher average values (medians ranging between 33.5 and 40.0 mm) than the other treatment-regime combinations in the experiment, except for LMS (median = 30). LMS significantly differed from neither treatment-regime combination.
Fig. 5 - The values of germinant size recorded in the particular treatment regime combination on days 7, 14 and 21 from the start of the germination test. (CO): control + optimal conditions; (LO): low concentration + optimal conditions; (LMO): lower-medium concentration + optimal conditions; (HMO): higher-medium concentration + optimal conditions; (HO): high concentration + optimal conditions; (CS): control + heat stress; (LS): low concentration + heat stress; (LMS): lower-medium concentration + heat stress; (HMS): higher-medium concentration + heat stress; (HS): high concentration + heat stress. Different letters below the box plots denote significant (p<0.05) differences within particular treatment-regime combinations, and an asterisk indicates that a particular treatment-regime combination was not included in the statistical analysis due to insufficient data. Outliers and extremes were not included to simplify the graph and make it more intelligible.
On day 14, the germinant-size values recorded in the compared treatment-regime combinations were more differentiated (compared with day 7), manifesting more visibly the effect of stress of the induced heat (Fig. 5). CS and LS showed the lowest values (median = 21 and 22 mm, respectively), whereas HMO the highest value of the germinant size (median = 42 mm).
On day 21, the stressing effect of the induced heat event was still visible, except for CS. However, the significance of the differences among the treatment-regime combinations was already affected by the low numbers of germinants between days 14 and 21 because the germination had already decreased. The values of cumulative germination approached their limits in the third week of germination (see Fig. S1 in Supplementary material). The last germinant recorded in LS also showed the lowest value (20 mm) among the compared treatment-regime combinations. The greatest germinant size was registered in LMO (median = 30 mm) and CS (single value of the last germinant = 30 mm).
Discussion
Our study aimed to assess: (i) whether the exogenous application of 2α,3α,17β-trihydroxy-5α-androstan-6-one can improve the germination capacity and germination energy of European black pine (Pinus nigra) seeds subjected to the optimal and heat-stress regime of germination; (ii) which of the four concentrations of the tested BR is the most effective.
High temperature induces growth retardation and oxidative stress in plants by the overproduction of reactive oxygen species that can damage lipids, proteins and nucleic acids ([58], [47]). The application of BRs has been reported to alleviate heat stress in plants ([53], [47]).
In our experiment, the germination of black pine seeds was markedly decreased by the temporary heat stress, although the stress event occurred relatively close to the first count date. The applied BR enhanced the germination of black pine seeds and also alleviated the effects of heat stress on the germination energy and the germination capacity to some extent. From the tested BR concentrations, the higher-medium concentration (0.04 mg l-1) most efficiently raised the germination energy in both temperature regimes (Fig. 2) and the germination capacity in the heat-stress regime (Fig. 3). However, even the higher-medium concentration of BR was unable to compensate for the heat stress completely. The high concentration (0.4 mg l-1) of the tested BR most efficiently enhanced germination capacity in the optimal regime (Fig. 3).
The values of the germination rate (Fig. 4) also suggest the detrimental effect of heat stress on the germination of black pine seeds. These values indicate that the higher-medium and high concentrations are the most efficient among those tested in our experiment. Nonetheless, the differences in germination rates among the compared treatment-regime combinations were seldom significant, likely due to a limited number of values entering the statistical analysis and, to some extent, the variability of these values or the statistical methods employed.
Li et al. ([25]) tested various concentrations of natural BR on seeds of Chinese pine (Pinus tabulaeformis Carr.). Among the tested concentrations (0.01, 0.05, 0.1 and 0.5 mg l-1), the 0.05 mg l-1 showed the best stimulating effect on the germination energy and the germination capacity of Chinese pine seeds. A BR concentration of 0.01 mg l-1 induced the second most beneficial effect on the germination capacity and energy of the seeds. This effect was practically comparable to the best-ranked concentration (0.05 mg l-1) and markedly better than the effect of BR concentrations of 0.1 mg l-1 and 0.5 mg l-1. Cukor et al. ([9]) reported the potential of BR (2α,3α,17β- trihydroxy-5α-andro-stan-6-one) to reduce the impact of temporary heat stress on the germination capacity of Scots pine (Pinus sylvestris L.). BR concentrations of 0.4 and 0.04 mg l-1 seemed effective in reducing the negative impacts of heat stress on germination capacity. However, in the non-stress regime, the low concentration of BR (0.004 mg l-1) appeared to be the most efficient in increasing the germination capacity.
As for trees of other genera, Li et al. ([25]) tested a wide range of concentrations of natural BR (0.0001 to 0.5 mg l-1) on seeds of black locust (Robinia pseudoacacia L.). The positive effects on germination parameters gradually rose with increasing BR concentrations up to 0.1 mg l-1 where the highest values of germination energy and germination capacity were found. At a BR concentration of 0.5 mg l-1, there was already an evident decrease in the positive effect, although this concentration also promoted germination. Li et al. ([26]) tested the application of natural BR on the germination of seeds of the tree of heaven (Ailanthus altissima [Mill.] Swingle). Among the different concentrations of BR (0.1 to 0.6 mg l-1), that of 0.4 mg l-1 proved optimal. Pretreatment of seeds with a natural BR solution of 0.4 mg l-1 increased the germination energy by 18.8% and shortened the mean germination time by 1.4 days.
The above-referred literature sources show that the value of optimal BR concentration varies for different species of trees in different situations. However, all of these studies suggest that BRs can promote tree germination. Therefore, each similar study may provide important insight to complement our knowledge on the effects of BR on the germination of a particular tree species, thereby directing further research and indicating possible applications in forestry practice.
The germinant size serves as a supporting parameter in our study, showing that the temporary temperature stress did not only slow down the seed germination process but had more profound consequences. If we only saw temporarily slowed germination after the heat-stress event (induced before the first census, which took place on day 7), we would expect an increase in the average size of the stress-treated germinants at the second census (day 14). This would be because the germinated seeds from the first week, whose growth would only be delayed by heat stress, would have had another week to grow before being counted and measured on day 14. However, there was no increase in the size of the germinants from the heat-stress treatment at the second count (day 14 - Fig. 5).
On the other hand, heat did not completely halt the germination of seed lots in the heat-stress treatments, although it did reduce it significantly. This can presumably be attributed to the fact that heat stress was induced in a single and relatively short episode. The timing of heat stress and the stage of germination of individual seeds played an important role.
Although many studies have been conducted, and extensive progress has been achieved during the previous decades ([32]), the particular mechanisms by which BRs improve plant resistance primarily concerning abiotic stress remain at least in some aspects unexplained ([17], [48]), and further research is needed to understand them in detail ([32]). It seems that BRs mediate heat tolerance through several complex ways ([2]); however, only some of these mechanisms may be applicable in explaining the positive effects of exogenously applied BR on germinating seeds. The assumption is that BRs activate or intensify the functioning of the antioxidant defensive system ([20]) consisting of enzymatic antioxidants, such as superoxide dismutase (SOD), peroxidase (POD), catalase (CAT), ascorbate peroxidase (APX), and non-enzymatic antioxidants, such as ascorbic acid (AsA) or tocopherols ([8], [47]). These compounds help plants to overcome temperature stress and regenerate after it. Under heat stress, the exogenous application of BRs (including their synthetic analogues) can significantly decrease MDA ([53]), whose content is widely used to indicate oxidative damage to membrane lipids and may be toxic at high levels. It should be noted that the role of MDA in plants is complex. MDA also exerts a positive role in activating regulatory genes involved in the defence system. However, MDA needs to be soon and efficiently eliminated by the plant because the toxicity of MDA holds if its levels remain high, irreparably modifying proteins and nucleic acids ([36]). Therefore, we can specify that BRs may contribute to the balance by reducing too-high levels of MDA.
Zhang et al. ([58]) studied the role of 24-epibrassinolide in the thermotolerance of melon (Cucumis melo L.). The heat stress significantly increased the action of antioxidant enzymes. In the presence of BR under heat stress, the activities of SOD, POD, CAT and APX were 13.3%, 59.2%, 95.5% and 33.7%, respectively, compared to the heat stress alone. The applied BR also significantly reduced the content of MDA. Similarly, Jin et al. ([19]) reported that 24-epibrassinolide alleviated the oxidative stress in seedlings of Ficus concinna (Miq.) Miq., induced by high temperature through stimulation of the enzymatic and non-enzymatic antioxidant and glyoxalase systems. On the other hand, the effect of BRs on heat-stress alleviation may depend on the species or cultivar ([47]). Mazorra et al. ([34]) observed the heat-shock response in tomato brassinosteroid mutants and concluded that thermotolerance was independent of brassinosteroid homeostasis.
Rather than explaining the mechanisms of BR functioning, the general goal of our study was to add more experience regarding the response of a particular plant to BR application. As in previous studies ([23], [9]), our work aimed to learn more about the seed response to BR application of a particular forest species that is commonly used in European forestry. In the context of climate change, the presence of stressors such as heat or drought is expected to occur more frequently in Europe ([21], [54], [55]). In our opinion, knowledge of the response of specific tree species to certain concentrations and formulations of BRs in the presence of concrete stressors is as crucial for practical forestry as explaining the mechanisms of their functioning for plant physiology.
To summarise our results and their implications for forestry practice, the brassinosteroid application promoted the germination of black pine and partly alleviated the impact of the temporary heat stress. However, this application did not compensate for the heat stress effects completely. The potential for the use of the brassinosteroid may exist for seedings of Pinus nigra in forest nurseries where the nursery staff try to maintain optimal climatic conditions, and deviations from these conditions are usually only temporary.
Conclusions
The heat substantially reduced the germination of black pine seeds. The values of the germination energy and germination capacity of seeds in the treatments subjected to heat stress were markedly and, in most cases, significantly lower than those of seeds germinating in the treatments in the optimal temperature regime. The BR application significantly increased the germination capacity of black pine seeds at the concentrations of 0.02, 0.04 and 0.4 mg l-1 within both (optimal and heat-stress) temperature regimes. The BR concentrations of 0.04 and 0.4 mg l-1 resulted in significant positive effects on the germination energy within both temperature regimes. However, the BR application did not erase the differences between these temperature regimes. Considering all parameters and aspects evaluated in this study, we can view the higher-medium concentration (0.04 mg l-1) of the tested BR as suitable for the recommendation before seeding. Nevertheless, in one case (germination capacity in the optimal regime), the high concentration of the BR showed better results.
The values of germination rate and size of germinants recorded in the compared treatment-regime combinations support (though mostly indirectly) the findings described above. That is because the differences in germination rate and size of germinants among treatment regime combinations were seldom statistically significant. However, the values of these additional parameters follow a similar pattern to that of the germination energy and germination capacity.
Based on our data, we can conclude that the BR application in our experiment promoted the germination of black pine and also partly alleviated the impact of the temporary heat stress, although the BR application did not compensate for the heat stress effects completely.
Acknowledgements
Our thanks go out to Nad’a Rašáková for the help in the laboratory, Linda Rostislav for assistance with the data processing, Josef Gallo for other support and to Jitka Šišáková and Richard Manore for proofreading the manuscript. The paper was accomplished thanks to the support provided by the Technology Agency of the Czech Republic (projects no. TACR QK22020045 and TACR SS01020189). The research was conducted in the Truba laboratory under the Department of Silviculture, Faculty of Forestry and Wood Sciences of the Czech University of Life Sciences in Prague.
List of abbreviations
The following abbreviations have been used throughout the paper:
- APX: ascorbate peroxidase
- AsA: ascorbic acid
- BR: brassinosteroid (if a particular steroid is referred)
- Brs: brassinosteroids (stands for brassinosteroids in general)
- CAT: catalase
- DNA: deoxyribonucleic acid
- GP: percentage of completely germinated seeds
- GR: germination rate
- MDA: malondialdehyde
- POD: peroxidase
- RNA: ribonucleic acid
- SOD: superoxide dismutase
- TBARS: thiobarbituric acid reactive substances
References
Gscholar
Gscholar
CrossRef | Gscholar
CrossRef | Gscholar
Gscholar
Gscholar
Gscholar
Gscholar
CrossRef | Gscholar
Gscholar
CrossRef | Gscholar
Gscholar
Gscholar
CrossRef | Gscholar
Supplementary Material
Authors’ Info
Authors’ Affiliation
Ivan Kuneš 0000-0002-1875-384X
Martin Baláš 0000-0003-4613-5907
Vilém Podrázský 0000-0002-6736-5640
Miroslav Šulitka 0000-0003-2066-259X
Jirí Remeš 0000-0003-4277-615X
Faculty of Forestry and Wood Sciences, Czech University of Life Sciences Prague, Prague (Czech Republic)
Corresponding author
Paper Info
Citation
Suraweera PACNB, Kuneš I, Baláš M, Podrázský V, Šulitka M, Remeš J (2024). Use of brassinosteroids to overcome unfavourable climatic effects on seed germination in Pinus nigra J. F. Arnold. iForest 17: 1-9. - doi: 10.3832/ifor4340-016
Academic Editor
Michele Carbognani
Paper history
Received: Mar 01, 2023
Accepted: Nov 07, 2023
First online: Feb 02, 2024
Publication Date: Feb 29, 2024
Publication Time: 2.90 months
Copyright Information
© SISEF - The Italian Society of Silviculture and Forest Ecology 2024
Open Access
This article is distributed under the terms of the Creative Commons Attribution-Non Commercial 4.0 International (https://creativecommons.org/licenses/by-nc/4.0/), which permits unrestricted use, distribution, and reproduction in any medium, provided you give appropriate credit to the original author(s) and the source, provide a link to the Creative Commons license, and indicate if changes were made.
Web Metrics
Breakdown by View Type
Article Usage
Total Article Views: 3011
(from publication date up to now)
Breakdown by View Type
HTML Page Views: 2238
Abstract Page Views: 257
PDF Downloads: 486
Citation/Reference Downloads: 1
XML Downloads: 29
Web Metrics
Days since publication: 85
Overall contacts: 3011
Avg. contacts per week: 247.96
Article Citations
Article citations are based on data periodically collected from the Clarivate Web of Science web site
(last update: Feb 2023)
(No citations were found up to date. Please come back later)
Publication Metrics
by Dimensions ©
Articles citing this article
List of the papers citing this article based on CrossRef Cited-by.
Related Contents
iForest Similar Articles
Research Articles
Effects of brassinosteroid application on seed germination of Norway spruce, Scots pine, Douglas fir and English oak
vol. 10, pp. 121-127 (online: 02 October 2016)
Research Articles
Influence of mother plant and scarification agents on seed germination rate and vigor in Retama sphaerocarpa L. (Boissier)
vol. 7, pp. 306-312 (online: 08 April 2014)
Research Articles
Role of serotiny on Pinus pinaster Aiton germination and its relation to mother plant age and fire severity
vol. 12, pp. 491-497 (online: 02 November 2019)
Technical Reports
Effects of different mechanical treatments on Quercus variabilis, Q. wutaishanica and Q. robur acorn germination
vol. 8, pp. 728-734 (online: 05 May 2015)
Research Articles
Optimum light transmittance for seed germination and early seedling recruitment of Pinus koraiensis: implications for natural regeneration
vol. 8, pp. 853-859 (online: 22 May 2015)
Research Articles
Seed germination traits of Pinus heldreichii in two Greek populations and implications for conservation
vol. 15, pp. 331-338 (online: 24 August 2022)
Research Articles
Germination and seedling growth of holm oak (Quercus ilex L.): effects of provenance, temperature, and radicle pruning
vol. 7, pp. 103-109 (online: 18 December 2013)
Research Articles
Outcome of Ceratocystis platani inoculations in Platanus × acerifolia in relation to season and inoculum dose
vol. 9, pp. 608-617 (online: 17 March 2016)
Short Communications
Evidence of Alectoris chukar (Aves, Galliformes) as seed dispersal and germinating agent for Pistacia khinjuk in Balochistan, Pakistan
vol. 14, pp. 378-382 (online: 22 August 2021)
Research Articles
Allelopathic effects of dominant ground vegetation species on initial growth of Pinus sylvestris L. seedlings in response to different temperature scenarios
vol. 12, pp. 132-140 (online: 27 February 2019)
iForest Database Search
Search By Author
Search By Keyword
Google Scholar Search
Citing Articles
Search By Author
Search By Keywords
PubMed Search
Search By Author
Search By Keyword