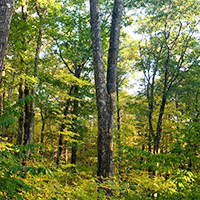
Changes in aboveground biomass following alternative harvesting in oak-hickory forests in the eastern USA
iForest - Biogeosciences and Forestry, Volume 8, Issue 5, Pages 652-660 (2015)
doi: https://doi.org/10.3832/ifor1349-007
Published: Jan 13, 2015 - Copyright © 2015 SISEF
Research Articles
Abstract
Managing forest lands for the sustainability of ecosystem functions and services by developing and implementing sound silvicultural methods through site-specific practices is a core concept in ecosystem management. In this study, we used long-term data collected at the extensive plots of the Missouri Forest Ecosystem Project (MOFEP) in the southeastern Missouri Ozarks (USA) to study the changes in aboveground biomass (AGB) under three silvicultural treatments: even-aged management sites (EAM), uneven-aged management sites (UAM), and non-harvested management sites (NHM). Treatments changed the magnitude of AGB dynamics. The forests maintained an AGB of 147.9 Mg ha-1 in 1990 and it increased to 175.6 Mg ha-1 by 2009. The forests were manipulated with four treatments: clear-cut, non-harvest, uneven-aged single-tree, and uneven-aged group selection and yielded AGB values of 30.7, 139.5, 125.7, and 148.7 Mg ha-1 of AGB in 2009, respectively. Over the 18-year study period, these forests accumulated 1.78 ± 0.26 Mg ha-1 yr-1, ranging from 1.60 to 1.94 Mg ha-1 yr-1 at the NHM plots. Changes in the net AGB growth rate were contributed by different growth rates of live trees and mortality and exhibited clear intra-annual variation during the five sampling periods. We observed a decreasing contribution of Quercus velutina (black oak) AGB (~6%), an increasing trend for Q. alba (white oak), and a stable change for Q. coccinea (scarlet oak) during the study period.
Keywords
Aboveground Biomass, MOFEP, Oak-hickory Forest, Forest Management, Alternative Harvest, Carbon
Introduction
Increasing pressure is being placed on forest managers for the sustainability of ecosystem functions and services by developing and implementing sound silvicultural methods for their forest lands. A particular challenge, in this regard, is to seek site-specific management options because of the variations among ecosystem types and between the regions and their importance at different temporal scales (e.g., short-term vs. long-term values - [25]). Stimulated by these needs, numerous large-scale experiments in the United States ([11]) and elsewhere ([17], [34], [15], [29]) were initiated to manipulate ecosystem structures and compositions. These experiments, unlike conventional approaches in forestry and ecology ([36]), were designed to mimic natural and human disturbances at large spatial scales and to quantify the ecological responses with sound controls of the manipulations and statistical confidence (i.e., random block design with at least three replications). For example, a full factorial design was employed in the Missouri Forest Ecosystem Project (MOFEP) in the southeastern Missouri Ozarks (MO, USA) to examine even-aged, uneven-aged, and no-harvest silvicultural treatments at the landscape level (100s ha to 1000s km2). Similarly, in the Teakettle Experimental Forest (TEF) in the California Sierras (USA), a group of scientists carefully selected eighteen 4-ha plots for experiments on the effects of prescribed burning and thinning ([32]). The DEMO project in southern Washington ([2]) and the Vermont Forest Ecosystem Management Demonstration Project in central Vermont (USA - [23]) were all initiated to address similar challenges by collecting direct evidences for assessing the gains and losses of alternative management plans on specific ecosystem functions. These studies have some features in common, including: (1) manipulations were made at the ecosystem level; (2) comprehensive measurements were made of species, ecosystem processes, biophysical changes, and social-economic metrics; (3) diverse expertise and multiple teams worked at the same sites; and (4) challenges occurred in data sharing, cross-team integration, synthesis, and securing funding for long-term endeavors.
The oak-hickory forest is the most important hardwood forest in the eastern USA, with a total area of 51 million ha ([3], [6]). Oak and hickory woods are also the main commercial wood products in the USA. Currently, large amounts of oak-hickory forests, either publicly or privately owned, are under timber harvest rotations (i.e., clear-cutting and thinning) for commercial timber. Clear-cutting has long been recommended as the primary harvest method for oak-hickory forests because it favors the regeneration of the market-preferred oak species ([35]). However, the even-aged method of clear-cutting caused large disturbance and, therefore, raised many problems such as habitat loss, nutrient leaching, and carbon emission ([16]). On the other hand, uneven-aged methods (e.g., group opening cut, single-tree selection) and even-aged thinning methods with the objective of reshaping the forest structure are less intensive in terms of reductions in stand density and canopy cover. These alternatives had been proposed to maintain and enhance other ecosystems services (e.g., wildlife habitat) of the forests in addition to timber quality. Therefore, it is recommended to today’s forest managers in the USA ([30], [14]). These methods were evolved from the conventional timber-oriented silvicultural methods of Europe, North America, and South America to enhance the structural and species complexity of harvested sites at multiple spatial and temporal scales ([16]). However, there has been no consensus on how alternative harvesting methods may promote aboveground biomass (AGB
) storage and growth in oak-hickory forests in light of carbon sequestration. Most previous studies looked into uneven-aged methods and thinning or clear-cutting independently, rather than through comprehensive comparisons of the tradeoffs among the conventional methods. Additionally, previous research lacked long-term data from sound experimental designs and, hence, fell short in assessing the dynamics of the AGB
growth. Moreover, harvest activities will inevitably change forest structure and species composition, which would bring additional direct and indirect effects to the dynamics of the AGB
.
The long-term data collected from the extensive plots of the MOFEP experiment provides us a great opportunity to study the AGB
growth under different silvicultural practices. In this study, we focused on how different silvicultural treatments may change the forest AGB
and dynamics over the harvesting re-entry or cutting. The experimental treatments (comprising this experiment) include the even-aged methods of clear-cutting and intermediate thinning and the uneven-aged methods of single-tree selection and group selection compared to a no-harvest treatment. By 2010, the experimental forests had approached the ends of their first entry (i.e., cutting cycle), during which six consistent surveys were conducted. Using the survey data, our objectives are to: (1) evaluate and compare the changes in the AGB during the first 15 years post-harvest among the treatments with pre-harvesting conditions in 1990; and (2) quantify the changes in major species and their contributions to the stand AGB
. By achieving the above study objectives, we expect that the lessons learned will be compared laterally with other similar experiments and that the managers may modify their future plans for the Ozarks and elsewhere.
Methods
Study sites and experimental design
The MOFEP is a long-term manipulative experiment that was initiated by the Missouri Department of Conservation (MDC) to evaluate the effects of alternative forest management on multiple ecosystem structures and functions over at least a 100-year period ([38]). The study region in the southeast Missouri Ozarks was covered in rich lush forests with domination of multiple oak species, commonly known as the mixed oak-hickory forests. Unfortunately, the Ozark landscape was nearly cleared for use in railroad ties and timbers by 1930. Today’s forests were naturally regenerated, covering >90% of the Ozarks. Among the many pressing issues on the management of these forests for the future, one is to understand how different silvicultural methods may alter their functions and services. A particular question is on understanding the mechanisms regulating the declines of oaks across the landscape ([13]). Historically, shortleaf pine was much more abundant in the southeastern Ozarks, including the MOFEP sites, than it is today. Extensive logging from 1880 to 1920, along with a rapid increase in fire frequency and open-range grazing, greatly favored an increase in hardwood abundance, particularly for oaks, and prevented the establishment and growth of shortleaf pine. Wildfire suppression began in the 1930s, which also favored an oak-dominated forest since shortleaf pine is shade-intolerant. The MDC initiated a massive project (i.e., the MOFEP) in the early 1990s to collect valuable experimental data in a long-term, top-to-bottom study to determine the ongoing and future needs of Missouri’s forests ([21]).
The MOFEP is located at the boundaries among Shannon County, Reynolds County, and Carter County in the southeastern Missouri Ozarks (Fig. 1). It is a physiographic and geologic highland region of the central USA and an extension of the southwestern Appalachian Mountains. The forests in this area are mainly upland oak, oak-hickory, and a few oak-pine communities ([5]). Dominant species include black oak (Quercus velutina), white oak (Q. alba), scarlet oak (Q. coccinea), post oak (Q. stellata), hickory (Carya spp.), and black gum (Nyssa sylvatica). Shortleaf pine (Pinus echinata) is the only pine species in our study sites ([26]). The mean annual temperature and total annual precipitation are 13.3 °C and 1120 mm, respectively. The whole area was not glaciated and has not been under water for 250 million years ([4]). The soils are mostly Alfisols and Ultisols formed by the weathering of the underlying Ordovician and Cambrian sandstones and dolomites ([21]).
Fig. 1 - The location and experimental design of the Missouri Ozarks Forest Ecosystems Project (MOFEP) in southeastern Missouri, USA.
The MOFEP experiment includes three types of treatments with sizes ranging from 312 to 514 ha for each of nine compartments: even-aged management sites (EAM), non-harvested management sites (NHM), and uneven-aged management sites (UAM), each of which has three replicated compartments ([5]). All of the compartments were distributed as randomized completed block designs ([36]). At each compartment, the land was divided into stands of ~3.2 ha with similar ecological land types (ELTs) according to common slope and aspect ([5]).
Harvest prescriptions at the EAM compartments followed the MDC Forest Land Management Guidelines ([31]) as well as the guidelines of Roach & Gingrich ([35]). All EAM compartments have a harvesting rotation of 90-105 years for 90% of the area within each compartment. For the EAM compartments, there are four types of treatments: clear-cutting (CC), intermediate-cut (I), non-harvest left (L), and non-harvest old-growth (OG). For the CC stands, which are restricted to 10-12% of the areas of the whole sites at each cutting cycle, all of the trees were non-selectively cut, but a few trees were left in reserve as seed sources for shortleaf pine or wildlife benefits; in the I treatment stands, intermediate thinning was applied given that stands had enough timber for commercial sale ([4]). The objective of the I treatment is to prepare it for future clear-cutting cycles; therefore, those trees of low commercial value or poor condition will be cut in order to free space for other trees ([37]). L stands were not cut in the first cutting cycle but would join the further harvest rotations, unless they were left as old-growth, indicating that the structures and functions of the L stands at the EAM, UAM, and NHM remain the same. Apart from the 90% CC, I, and L, the remaining 10% of the plots in the EAM were managed as OG, which is reserved throughout the rotation. Similarly, there are four types of treatments at the UAM compartments: uneven-aged single-tree selection (U), uneven-aged group selection (UG), L, and OG. In plots with uneven-age selection, 5% of the stand acreage was in group openings based on Law and Lorimer’s principles ([5]), which gives approximately 1/3 full sunlight for ~20 m tall trees, which is required by oaks for maximum photosynthesis. The size of the circular openings ranged from 21 m, 32 m, and 42 m on southwest slopes, ridges, and northeast slopes, respectively. The UG treatment represents plots that had a combination of single-tree selection and group openings where all trees >1.4 m tall were slashed. During the first harvest in 1996, 41-69% of the forests at the UAM compartments received both U and UG treatments. The objective of U is to maintain stand quality and a reverse J-shape tree size distribution that favors tree growth ([38]). At all of the UAM harvested stands, UG is also applied to 5% of the total stand area. The UG areas have three opening sizes: ~415 m2 for south-facing slopes, ~800 m2 for neutral slopes, and ~1450 m2 for north-facing slopes ([38]), enabling the center of the ground opening to receive 33% full sunlight. In our study, we treated all of the plots that have areas overlapping with the open-ground cut areas as UG treatments (although, they also underwent U treatments at the same time) and others as U treatments (without UG overlapping inside the plots). Altogether, a total of 46 UG plots and 80 U plots were identified. Similar to the EAM, L will be harvested sometimes while OG will not. In the NHM, none of the plots are harvested and, therefore, will be treated as L in this study.
Data collection
Overall, a total of 648 vegetation plots for all nine compartments were installed in 1991.
All of the overstory trees and snags, defined as trees ≥11.5 cm dbh, were measured in the entire 0.2 ha area within each of the 648 plots. Understory trees within the range 3.8 to 11.5 cm dbh were measured at four 0.02 ha subplots, which are located at the south, north, east, and west plot edges from the center. The dbh measurements were taken six times during the non-growing seasons of 1990-1991 (i.e., pre-treatment), 1994-1995, 1997-1998, 2001-2002, 2005-2006, and 2009-2010. The measurements in 2001-2002, 2005-2006, and 2009-2010 started after the growing season of the first year; therefore, they should be considered as data for 2001, 2005, and 2009 because dbh does not change during the non-growing season. Most measurements in 1997-1998 were also finished before the 1998 growing season or at the beginning of the growing season; therefore, we considered this measurement as growth after 1997. For all of the 648 plots, 19 plots are mixed with two treatments (i.e., I and CC) or are glade plots that generally do not have high enough tree densities to be considered as forests; therefore, they were excluded from this study. Consequently, we have a total of 629 vegetation plots for data analysis. Among the 179 vegetation plots in the EAM compartments, 30 plots were marked as clear-cut, 33 were intermediate cut, 116 were non-harvest, and 26 were old-growth in the EAM compartments. In the UAM compartments, 80 plots were single-tree selection cut, 46 were group selection cut, 51 were non-harvest, and 32 were old-growth non-harvest in the UAM compartment. There are 215 plots at the NHM compartments. Within each stand, at least one 0.2 ha circular vegetation plot was randomly assigned for regularly scheduled tree inventories of dbh, canopy dominance, species, vigor surveys, snags, etc. Vegetation plots were established for the inventory of overstory trees (≥11.5 cm dbh), understory trees (3.8 to 11.4 cm dbh), downed logs, and plot physical characteristics (slope, slope position, aspect, soil types, etc. - [5]). The use of dbh classes (i.e., 11.5 cm) was based on forest management and timber harvesting standards for the Ozarks region.
Statistical analysis
The dbh data collected during the 18-year period was used to calculate the AGB
from the allometric equations that were derived from previous studies, according to the empirical relationship between AGB
and dbh ([43], [28] - eqn. 1):
where AGB
(Mg ha-1) is the aboveground dry weight of the α
tree and β
is an empirical coefficients estimated by regression analysis for each tree species. For some rare species that do not have empirical allometric equations, we used the equations from other minor species that are from the same genus first, then from family, and lastly from a similar species ([28]). Since the rare species only contribute about 5% of the dataset, we are confident that the calculated AGB
for each plot is sufficiently accurate for further data analysis. For each of the 629 plots, the weights of all overstory trees were summed to obtain the plot overstory AGB
for each of the six inventory time periods. Similarly, we obtained the understory AGB
for each of the four subplots at every plot. The total AGB
of each plot is calculated as the sum of overstory and understory AGB
.
Given that both the EAM and UAM compartments have L plots, the effects of the individual timber harvest treatments on the AGB
growth were analyzed independently within the EAM and UAM treatments to minimize the variability related to the treatment type. The NHM compartments were also analyzed as the references of the L (i.e., L and OG treatments) in the EAM and UAM treatments. Our independent analyses among EAM, UAM, and NHM will also minimize the potential errors caused by the spatial positions of the compartments. For example, the effects of the U treatment on the AGB should be compared to the L and OG in the UAM, rather than the L and OG in the EAM compartments. Finally, linear regressions were employed by treating a year as an independent variable for predicting the AGB
from 1997 to 2009 (i.e., after the harvesting manipulations) and the annual AGB
growth rate for each plot. The annual AGB
growth rate for each plot was then averaged by treatment (i.e., EAM, UAM, and NHM). A one-way ANOVA was used to test if there was a significant difference among the treatments at p=0.05.
The AGB
data series with the above calculations show only the AGB
growth of live trees. The net AGB
of a stand is the result of both tree growth and mortality. Quantifying both the changes from tree growth and mortality will enable us to distinguish the effects of harvesting on the two dominant processes driving the net AGB
. Here, we calculated the dead tree biomass using the same method of allometric equations for each plot and treatment. The change in dead tree biomass is considered as the AGB
loss between the two consecutive measurements. The AGB
gross growth rate and AGB
loss rate by year are calculated as follows (eqn. 2, eqn. 3):
where GGR
(Mg ha-1 yr-1) is the gross AGB
increase from the growth of live trees, AGB
t2 and AGB
t1 are the AGB
at year t1 and t2, respectively, and DB
t2 is the newly found dead trees at t2 to calculate the AGB
loss rate from mortality (LR
). To understand how harvesting has affected the AGB
storage among the tree sizes and species, we also calculated the percentage of the AGB
contribution to the total biomass by the dbh class. The AGBs were categorized into 16 groups at each 5 cm dbh class by species and treatment (i.e., CC, I, L, OG, U, UG). We did not separate the L or OG at different compartments because our preliminary analysis suggested very small differences between the plots of these two types.
A second data analysis was aimed at assessing the AGB
changes to investigate whether species may respond to the same treatment differently and, consequently, their contributions to the storage, and whether the dynamic of the AGB
following the treatments might be species-dependent. The AGB
proportion of each species to the total AGB
s of black oak, white oak, scarlet oaks, post oak, black hickory (Carya texana), pignut hickory (C. glabara), mockernun hickory (C. tomentosa), and shortleaf pine are calculated for L, OG, CC, I, U, and UG at each compartment for each survey year. All remaining minor species were combined as “Others” in this study.
Results
Treatment effect on AGB
Treatment had greatly changed the magnitudes and post-harvest dynamics of the AGB
at the MOFEP experiment (Fig. 2). The mixed oak-hickory forests at the MOFEP sites maintained an AGB
of 147.9 Mg ha-1 in 1990, which increased to 175.6 Mg ha-1 by 2009 (i.e., the mean AGB
of the L and OG treatments). Among the four manipulated harvest treatments in 1995, clear-cutting in the EAM compartments produced the greatest reduction on AGB
to near-zero, while uneven-aged single-tree selective thinning (U) at the UAM caused the lowest change in AGB
to 117.4 Mg ha-1, and uneven-aged group openings (UG) of the UAM and intermediate-cut (I) of the EAM reduced the AGB
s to 89.1 and 106.6 Mg ha-1, respectively. By 2009, the four harvested forests had AGB
values of 30.7, 139.5, 125.7, and 148.7 Mg ha-1 at CC and L of the EAM and U and UG of the UAM, respectively. The reduction and change in understory AGB
, however, has not been parallel to the total AGB
. Over the 18-year study period, there have been steady decreases in understory AGB
at the MOFEP forests from 9.3 Mg ha-1 to 7.2 Mg ha-1. The harvest treatments in the EAM compartments produced the greatest changes in the understory AGB
and the fastest rebounding, while these effects at other harvested sites seemed very small (~3 Mg ha-1) and disappeared ten years after the treatments.
Fig. 2 - Changes in total aboveground biomass (AGB
) and understory AGB
from 1991 to 2009 in even-aged management (EAM, left) and uneven-aged management (UAM, right) sites in the MOFEP experiment. EAM (CC: clear-cutting; I: intermediate-thin), UAM (single-tree selective thinning (U), uneven-aged group openings (UG), L: non-harvest left, and OG: non-harvest old-growth reserve (OG).
Forests at the MOFEP sites over the study period accumulated a mean (± SE) of 1.78 ± 0.26 Mg ha-1 yr-1, ranging from 1.60 to 1.94 Mg ha-1 yr-1 at undisturbed plots (Fig. 3). In the EAM compartments, the annual AGB
growth rates for CC, I, L, and OG were 2.78, 3.41, 1.82, and 1.89 Mg ha-1 yr-1, respectively. At the UAM sites, the annual AGB
growth rates for U, UG, L, and OG were 2.83, 3.44, 1.94, and 1.67 Mg ha-1 yr-1, respectively (Fig. 3). Overall, the harvesting increased the AGB
growth around 1 Mg ha-1 yr-1. Except for CC, the overstory AGB
accounted for ~94% of the total AGB
across all of the plots, with understories accounting for the remaining 6% (Fig. 2). At the CC, due to the lack of overstory, young and small trees contributed to almost all of the AGB
and its growth from the understory.
Fig. 3 - The changes in the mean (SE) net AGB growth rate (Mg ha-1 yr-1) from 1997 to 2009 by treatment of even-aged management (EAM) and uneven-aged management (UAM) in the MOFEP experiment. See Fig. 2 for abbreviations.
These changes in the net AGB
growth rate at the MOFEP experiment were contributed to by different growth rates of live trees and mortality and exhibited clear intra-annual variation during the five sampling periods (Fig. 4). In the controlled plots (i.e., L and OG treatments), the AGB
growth varied from 1.8 to 4.3 Mg ha-1 yr-1, with the lowest value during 1995-1997 at the OG of EAM and the highest value during 1997-2001 at the OG of the EAM compartments. The AGB
loss due to mortality, meanwhile, varied from 1.4 to 3.2 Mg ha-1 yr-1. The treatments caused higher variations in AGB
loss among the treatments but there was consistent AGB
growth at the I and U treatments, resulting in an average GGR
of 4 Mg ha-1 yr-1. The GGR
at the CC was the lowest; however, due to its minor AGB loss, its net AGB
growth remained the highest. The UG experienced very low AGB
growth right after the harvest, but it recovered fastest by a combination of high growth and low mortality (Fig. 3). Overall, it appeared that harvesting affected the intra-annual variations of AGB
loss more than that of growth.
Fig. 4 - The mean (SE) of aboveground biomass (AGB
) growth and loss through mortality and tree harvesting at five periods in the MOFEP experiment between 1990 and 2009. See Fig. 2 for abbreviations.
The treatments changed the AGB
distributions by dbh class. Stand density by dbh class was a reverse J-shape while it was a bell-shaped distribution for AGB
(Fig. 5). By 2009, there appeared to be reductions in trees at the smallest dbh class at the controlled plots, while the AGB
distribution was elevated and shifted to larger dbh classes at the control treatments (i.e., L and OG). The q-values were higher in 1998 - an indicator of a smaller amount of large trees - at the I, U, and UG, after the harvesting. The treatment reduced both stand density and AGB
distribution among the dbh classes, with clear shifts to smaller trees. In 2009, the q-values of stand density decreased at all manipulated sites, with dbh classes at 15-20 cm replacing those of 11.5-14.9 cm classes (Fig. 5). For the AGB
, its distributions and dbh classes of 35-40 cm and 40-45 cm had the highest AGB
for L and OG in 1997, while the highest AGB
was around 30-35 cm for U and UG and 25-30 cm for the I treatment. In 2009, all manipulated sites had peak values that returned to ~40 cm, which were similar to those of the controlled plots.
Fig. 5 - Distribution and changes in aboveground biomass (AGB
) and stand density by dbh class in 1990 and 2009 in the MOFEP experiment. See Fig. 2 for abbreviations.
Species contribution to AGB dynamics
Black oak, scarlet oak, white oak, post oak, shortleaf pine, black hickory, pignut hickory, and mockernut hickory were the eight dominant species contributing to ~95% of the total AGB
in all manipulated sites, which was slightly higher than 90% at the OG sites (Tab. 1). The three main species contributing to the AGB
were black oak, scarlet oak, and white oak, with each species accounting for >20% of the total AGB
in all treatments. At the controlled plots (L and OG treatments), we observed a decreasing contribution of black oak AGB
(~6%), an increasing trend for white oak, and a stable change for scarlet oak over the 18-year study period (Tab. 1). These changes in species contribution to the total AGB
were also evident from their net growth rates (Tab. 2). The only exception was black oak, with a negative growth rate, but it remained an increasing proportion to the total (Tab. 1 and Tab. 2). White oak has the highest growth rate (>2 Mg ha-1 yr-1) and its AGB
contribution increased by 5.7% in all of the treatments (Tab. 1). On the contrary, the proportion of black oak to the total AGB
decreased at ~5% (Tab. 1). These changes in dominancy between black oak and white oak were found regardless of the treatments (Tab. 1). Other species showed variable, negligible changes in their AGB
s and their contributions to the total AGB
.
Tab. 1 - The proportion of aboveground biomass (AGB
, %) by species in 1991, 1997, and 2009 in the MOFEP experiment. The treatments L and OG were undisturbed controls. (NA): not applicable.
Treatment | Year | B. oak | W. oak | S. oak | P. oak | S. pine | B. hickory |
P. hickory |
M. hickory |
Others | |
---|---|---|---|---|---|---|---|---|---|---|---|
EAM | CC | 1991 | 28.1 | 23.7 | 19.3 | 8.6 | 2.3 | 5.5 | 3.7 | 4.5 | 4.4 |
1997 | NA | NA | NA | NA | NA | NA | NA | NA | NA | ||
2009 | 24.6 | 34.4 | 23.8 | 0.1 | 4.9 | 0.2 | 1.2 | 1.1 | 9.7 | ||
I | 1991 | 33.6 | 20.4 | 19 | 5.9 | 5.6 | 5.3 | 3.6 | 4.2 | 2.4 | |
1997 | 27 | 24.4 | 16.6 | 5.1 | 6.4 | 6.2 | 4.9 | 4.9 | 4.5 | ||
2009 | 22.2 | 27.7 | 19 | 4.4 | 5.3 | 6 | 5.7 | 5.4 | 4.2 | ||
L | 1991 | 29.3 | 22.3 | 19.3 | 10.5 | 3.4 | 3.7 | 3.5 | 3.8 | 4.2 | |
1997 | 27.6 | 24.5 | 18.8 | 10.3 | 3.5 | 3.5 | 3.7 | 3.8 | 4.4 | ||
2009 | 24.1 | 27.4 | 19.9 | 9.3 | 3.4 | 3.4 | 4.2 | 4.1 | 4.2 | ||
OG | 1991 | 22 | 28.7 | 24.4 | 3.7 | 3 | 4.9 | 4 | 3.8 | 5.5 | |
1997 | 19.8 | 31.7 | 20.7 | 3.8 | 3.1 | 4.7 | 4.4 | 4.1 | 7.8 | ||
2009 | 15.5 | 35.5 | 19.7 | 3.7 | 3.1 | 4.9 | 4.8 | 4.2 | 8.6 | ||
NHM | L | 1991 | 28.4 | 20 | 25.5 | 7.6 | 4.6 | 5 | 3 | 1.9 | 4 |
1997 | 26.8 | 21.8 | 24.8 | 7.4 | 4.6 | 4.7 | 3.2 | 2.1 | 4.6 | ||
2009 | 22.4 | 24.8 | 24.4 | 7.1 | 4.9 | 4.5 | 3.9 | 2.5 | 5.4 | ||
UAM | L | 1991 | 26.6 | 14.9 | 31 | 9 | 4.9 | 3.1 | 3.1 | 3.6 | 3.9 |
1997 | 24.6 | 16.5 | 30.7 | 8.6 | 4.9 | 3 | 3.3 | 3.5 | 4.9 | ||
2009 | 20.6 | 19.3 | 31.4 | 7.6 | 4.9 | 3.2 | 4 | 3.7 | 5.3 | ||
OG | 1991 | 25.8 | 19.7 | 25.2 | 5.7 | 5.8 | 3.4 | 2.3 | 3.7 | 8.4 | |
1997 | 24 | 20.7 | 23.2 | 5.6 | 5.9 | 3.6 | 2.7 | 3.5 | 10.8 | ||
2009 | 19.9 | 25.1 | 20.5 | 5.2 | 6.4 | 3.4 | 3.3 | 4.1 | 12.2 | ||
U | 1991 | 27.7 | 19.1 | 25 | 6.9 | 5.1 | 3.3 | 4.4 | 4.7 | 3.7 | |
1997 | 28.1 | 20.8 | 22.5 | 6.4 | 6 | 3.4 | 5.1 | 4.1 | 3.6 | ||
2009 | 22.2 | 25.3 | 23.7 | 6 | 5.7 | 3.6 | 5.5 | 4.4 | 3.6 | ||
UG | 1991 | 30 | 14.2 | 31 | 5.9 | 3.6 | 3 | 4.5 | 4.4 | 3.3 | |
1997 | 27.8 | 15.6 | 29.1 | 6.4 | 5.1 | 3.3 | 4.5 | 4.7 | 3.5 | ||
2009 | 23.9 | 19.7 | 29.4 | 5.5 | 4.5 | 3.8 | 5.2 | 4.4 | 3.5 |
Tab. 2 - The overstory net aboveground biomass (AGB
) growth rate (kg ha-1 yr-1) by species at the MOFEP compartment.
Treatment | B. oak | W. oak | S. oak | P. oak | S. pine | B. hickory |
P. hickory |
M. hickory |
Others | |
---|---|---|---|---|---|---|---|---|---|---|
EAM | CC | 265 | 334 | 316 | 4 | 35 | 23 | 87 | 39 | 185 |
I | 328 | 1256 | 915 | 164 | 106 | 224 | 318 | 253 | 131 | |
L | 10 | 935 | 562 | 88 | 100 | 80 | 182 | 128 | 64 | |
OG | -374 | 1211 | 311 | 165 | 97 | 171 | 197 | 109 | 276 | |
NHM | L | -247 | 844 | 385 | 122 | 166 | 89 | 206 | 132 | 215 |
UAM | L | -91 | 753 | 776 | 45 | 160 | 127 | 252 | 120 | 190 |
OG | -167 | 991 | 37 | 70 | 306 | 66 | 210 | 190 | 425 | |
U | 87 | 1172 | 833 | 226 | 196 | 173 | 200 | 197 | 104 | |
UG | 464 | 951 | 995 | 236 | 167 | 200 | 305 | 143 | 137 |
Silvicultural treatments enhanced the net AGB
growth of all eight species, while it reduced the net AGB
growth of the other minor species (Tab. 2). U and I increased the growth rates of white oak and post oak more than UG, while UG increased the growth rate of black oak. The effects of I, U, and UG on the net AGB
growth rates of hickory species and shortleaf pine were not clear. CC reduced the net AGB
growth rates of all species except for black oak, resulting in a net negative growth rate (Tab. 3). The treatment effects on the net AGB
growth rate of shortleaf pine were not clear among the treatments.
Tab. 3 - The loss rate (kg ha-1 yr-1) of overstory aboveground biomass (AGB
) due to tree mortality and harvesting by species in the MOFEP experiment.
Treatment | B. oak | W. oak | S. oak | P. oak | S. pine | B. hickory |
P. hickory |
M. hickory |
Others | |
---|---|---|---|---|---|---|---|---|---|---|
EAM | CC | 40 | 3 | 10 | 2 | 2 | 1 | 3 | 13 | 1 |
I | 759 | 226 | 354 | 77 | 55 | 67 | 10 | 20 | 77 | |
L | 939 | 204 | 680 | 165 | 35 | 55 | 17 | 44 | 137 | |
OG | 999 | 315 | 998 | 25 | 20 | 44 | 25 | 48 | 176 | |
NHM | L | 1197 | 255 | 955 | 113 | 46 | 73 | 15 | 16 | 146 |
UAM | L | 978 | 118 | 893 | 179 | 44 | 28 | 13 | 40 | 89 |
OG | 987 | 208 | 886 | 93 | 34 | 43 | 4 | 21 | 295 | |
U | 605 | 151 | 579 | 48 | 61 | 34 | 77 | 40 | 77 | |
UG | 478 | 158 | 538 | 64 | 26 | 6 | 38 | 60 | 68 |
As stated before, the net AGB
growth was more a result of tree mortality from the harvest activities than a consequence of tree growth. However, this conclusion is species-dependent. Harvesting reduced the AGB
loss for oaks and other minor species (i.e., by comparing the loss rates among species in Tab. 3), while the harvest effects on hickories seemed to be more complicated. The U and UG treatments increased the AGB
loss of pignut hickory and mockernut hickory, but tended to reduce with black hickory. The I treatment showed no effect on the hickory AGB
loss. The I and U treatments increased the shortleaf pine AGB
loss, but the UG treatment reduced its loss (Tab. 3).
Discussion and onclusions
Treatment effects on AGB dynamics
Large-scale ecosystem studies are important because they provide a scientific base for addressing critical resource management issues (e.g., conservation of biodiversity, old-growth forests, carbon sequestration) and meet the desire of managers to practice stewardship across multiple spatial scales that involve multiple ownerships ([25], [11]). Globally, there has been a rapid increase in understanding how alternative management at stand, landscape, and regional levels can be implemented to increase the multiple functions (e.g., carbon, timber) and services (e.g., biodiversity) of forests though experimental and modeling exercises ([32], [25], [2]). For example, Swanson ([42]) demonstrated that clear-cutting and partial overstory retention with rotation lengths of 100 and 200 years produced contrasting results on carbon storage (i.e., biomass and its increment) in Chilean forests. Man et al. ([29]) also reported that reduced harvest levels stored significantly more carbon over the 100-year planning horizon as compared to strategies that focused on tree growth rates ([20]). Similar conclusions have also been drawn from boreal forests ([17], [29]), Europe ([15]), and Chile ([42]), Australia ([34]), and other regions.
The 60- to 70-year-old oak-hickory forests in the Ozarks were regenerated from clear-cutting in the early part of the 20th century ([13]). These forests store 148 Mg ha-1 of AGB
(Fig. 2), which is consistent with the previous reports of the Ozarks forests ([26]) and deciduous forests in North America ([7]). However, the above figure was lower than that reported by Gu et al. ([18]) at the Missouri Ozarks Flux (MOFLUX) site located in the Baskett Research and Education Area, which is 200 km away from the MOFEP site, where stand composition and structure are similar to that of MOFEP site. The MOFLUX is the nearest flux tower with direct carbon flux measurements. Our results also confirm the role of regenerating forests in contributing to the carbon sequestration of terrestrial ecosystems ([10], [11], [33]). A surprising result is that the AGB
of understory vegetation during the study period continued to contribute, suggesting that the forests continue moving to their stem-exclusion stage and their capacity to accumulate biomass will continue in the future, as supported by the recent studies showing the changes of forest productivity following disturbances ([1], [24]). Additionally, the annual accumulation of the AGB
in these forests (~2 Mg ha-1 yr-1) is based on the large ground sampling in the MOFEP site, which shows that the Ozarks remain a very productive landscape, with its carbon sequestration rate being higher than previously reported for the region ([44]).
The harvest effects on the stand dynamics of oak-hickory forests have been widely studied ([19], [20]), but no study looked into tree growth based on long-term experimental data or manipulations from the changes in biomass perspective. Here, based on the MOFEP experimental data over an 18-year period, we investigated for the first time the AGB
growth rates in the oak-hickory forests under different harvest treatments to understand the biomass accumulation (i.e., carbon accumulation) of the oak-hickory forests in the Ozarks. The results showed that all alternative harvesting manipulations implemented in the MOFEP design increased the net AGB growth from 2 to 3 Mg ha-1 yr-1 in the first 15 years of re-growth, which is higher than the average accumulation of undisturbed forests. Interestingly, the total AGB
after the U treatment with the UAM returned to its pre-harvesting level by 2000 (Fig. 2). While it may take decades for the CC sites to reach this level, the AGB
at the UG and I treatments will likely reach the pre-harvest level in another 5-10 years (i.e., trend projections from Fig. 2).
As the global community increases its attention on the roles of forests in carbon sequestration, the results from this study contribute further in situ evidence for quantifying the sequestration strength of the Ozarks region. However, we should be cautious to use the net AGB
rate increase as carbon credits because the net carbon gain of an ecosystem is also affected by its carbon loss through decomposition ([8]). A forest ecosystem becomes a carbon source after harvesting due to elevated respiration from harvest residuals and soil organic carbon (including roots - [10]). The balance between the accumulation and loss of carbon in forests and its dynamics following a disturbance are highly variable among forests ([1]). For example, Chiang et al. ([12]) reported that the AGB growth rate in Ohio in the eastern USA decreased during the first year after a thinning, but bounced back to pre-harvest levels within ten years. In our study, we found that changes in the net AGB
growth rate after the manipulation were more complicated than just counting the years after the disturbance. For example, the net AGB rate was low during the 1997-2001 period, but increased to higher than pre-harvest levels after 2001 (Fig. 2). Additionally, it seemed that the net AGB
growth at the MOFEP sites was more controlled by tree mortality and harvests than growth (Fig. 4), suggesting that harvests (except for clear-cutting) improved the capability of forest carbon sequestration through lowering tree mortality rather than through net primary production (i.e., photosynthesis). This is consistent with Yuan et al. ([45]) who found that the net ecosystem production of deciduous forests is determined more by respiratory loss than photosynthesis gain. The Langsaeter principle on the gross growth of forests, i.e., tree growth per unit area does not change when the stocking level changes ([41]), also supports our findings. Finally, whether harvesting increases the carbon assimilation capacity of the Ozarks oak-hickory forest is also dependent on the fate of the harvested wood. A comprehensive life cycle analysis (LCA) is the obvious future effort for assessing the consequences of alternative management options at the MOFEP sites and elsewhere. Nevertheless, our results showed that I, U, and UG can reduce AGB
loss through tree mortality and harvests by approximately 50% (i.e., 1 Mg ha-1 yr-1). Given a harvest cycle or rotation of about 105 years and assuming that harvested wood can be preserved as sequestrated carbon after being removed from the sites, harvested MOFEP forests would contribute an extra 7.5 carbon credits per ha for each rotation. However, if we consider that 30% of the harvested biomass is left on the forest ground (e.g., I, U, and UG removed forest biomass for 47, 34, and 67 Mg ha-1, respectively), and assume that 30% of the non-bole biomass remained on the ground ([41], [9]) that will decompose in the first 15-year rotation ([27]), the carbon loss would be 7.1, 5.1, and 10.0 Mg ha-1 for I, U, and UG, respectively. If we add the proportion lost from roots, the net carbon loss will be another 15-20% ([26], [28]). This will result in a much higher carbon debt that is positively related to the harvest intensity. Clearly, whether forest harvests will create carbon credits is dependent on the amount of biomass removed by harvesting and the use of harvested wood.
Species responses to harvest
The MOFEP experiment was not just designed to promote biomass growth, but has diverse purposes such as habitat improvement for wildlife, ground flora, and target oak species. One of the main objectives for seeking alternative management options is to reshape the species composition of the forest. For example, CC can aid in the regeneration of oak species and the I treatment removes the cull trees and undesirable species ([35]). The U treatment is for regenerating small trees through small openings by canopy gaps while thinning elsewhere to reallocate growing space ([20]), while the main objective of the UG is similar to the U, but it also facilitates the growth of shade-intolerant oak species in the group openings. In recent years, the Ozarks have undergone a widespread reduction of black and scarlet oak ([21], [22]), with white and post oak replacing them, due to the fact that black oaks are intolerant of low-light conditions, have short longevity, and are more susceptible to drought ([39]). Alternative management is sought to change this trend. However, we found a decline in the black oak proportion to the total AGB
, while white oaks showed an increase (Tab. 1). Other studies also suggested that the growth of oaks is more affected by their crown positions rather than species differences ([40]). Although black oaks usually grow faster than white oaks and, therefore, are more likely to be dominant or co-dominant trees in the stand, large black oaks also suffer from high a mortality rate from harvesting activities ([39]). As expected, we found a high AGB
loss for black oak at the control treatments, but harvesting further increased their loss by 20-50%. On the contrary, the harvest effect on white oak AGB
loss seemed negligible (Tab. 2). Even though the AGB
growth rate of black oaks increased from negative to positive, its proportion to the total AGB
was reduced. This is probably due to the high quantity and fast growth of white oaks in the overstory as well as a smaller proportion being harvested ([21]), whose collective growth was more important that the effects of harvesting. The biomass accumulation in other minor species, rather than the eight most common species, was also suppressed by harvesting, indicating that harvests are effective in shaping species composition toward economically beneficial species (i.e., oaks).
Acknowledgements
This study was partially supported by the Missouri Department of Conservation and the IceMe of NUIST. Lisa Delp Taylor edited the earlier drafts of the manuscript. Yanling Wang helped constructing the references. JC designed the overall experiment of this study and developed the final manuscript, JX compiled the survey data, drafted the first manuscript, and performed initial analysis, RJ collected all of the field data and provided detailed information on survey plots, and JK helped to integrate manuscript description.
References
CrossRef | Gscholar
Gscholar
Online | Gscholar
Online | Gscholar
Gscholar
CrossRef | Gscholar
Gscholar
Gscholar
CrossRef | Gscholar
CrossRef | Gscholar
Gscholar
Gscholar
CrossRef | Gscholar
CrossRef | Gscholar
Gscholar
Gscholar
Gscholar
CrossRef | Gscholar
CrossRef | Gscholar
Authors’ Info
Authors’ Affiliation
International Center for Ecology, Meteorology and Environment (IceMe), School of Applied Meteorology, Nanjing University of Information Science and Technology, Nanjing 210044 (China)
Missouri Department of Conservation, Ellington, MO 63638 (USA)
USDA Forest Service, Northern Research Station, Columbia, MO 65211 (USA)
Corresponding author
Paper Info
Citation
Chen J, Xu J, Jensen R, Kabrick J (2015). Changes in aboveground biomass following alternative harvesting in oak-hickory forests in the eastern USA. iForest 8: 652-660. - doi: 10.3832/ifor1349-007
Academic Editor
Raffaele Lafortezza
Paper history
Received: May 12, 2014
Accepted: Nov 02, 2014
First online: Jan 13, 2015
Publication Date: Oct 01, 2015
Publication Time: 2.40 months
Copyright Information
© SISEF - The Italian Society of Silviculture and Forest Ecology 2015
Open Access
This article is distributed under the terms of the Creative Commons Attribution-Non Commercial 4.0 International (https://creativecommons.org/licenses/by-nc/4.0/), which permits unrestricted use, distribution, and reproduction in any medium, provided you give appropriate credit to the original author(s) and the source, provide a link to the Creative Commons license, and indicate if changes were made.
Web Metrics
Breakdown by View Type
Article Usage
Total Article Views: 49654
(from publication date up to now)
Breakdown by View Type
HTML Page Views: 43068
Abstract Page Views: 2188
PDF Downloads: 3174
Citation/Reference Downloads: 23
XML Downloads: 1201
Web Metrics
Days since publication: 3756
Overall contacts: 49654
Avg. contacts per week: 92.54
Article Citations
Article citations are based on data periodically collected from the Clarivate Web of Science web site
(last update: Mar 2025)
Total number of cites (since 2015): 4
Average cites per year: 0.36
Publication Metrics
by Dimensions ©
Articles citing this article
List of the papers citing this article based on CrossRef Cited-by.
Related Contents
iForest Similar Articles
Research Articles
Outlook of the European forest-based sector: forest growth, harvest demand, wood-product markets, and forest carbon dynamics implications
vol. 11, pp. 315-328 (online: 18 April 2018)
Research Articles
Allometric relationships for volume and biomass for stone pine (Pinus pinea L.) in Italian coastal stands
vol. 6, pp. 331-335 (online: 29 August 2013)
Research Articles
Effects of traditional forest management on carbon storage in a Mediterranean holm oak (Quercus ilex L.) coppice
vol. 11, pp. 344-351 (online: 18 April 2018)
Research Articles
Voluntary carbon credits from improved forest management: policy guidelines and case study
vol. 11, pp. 1-10 (online: 09 January 2018)
Research Articles
Disassociating tree species associations in the eastern United States
vol. 7, pp. 248-254 (online: 13 March 2014)
Research Articles
Modeling aboveground carbon in flooded forests using synthetic aperture radar data: a case study from a natural reserve in Turkish Thrace
vol. 17, pp. 277-285 (online: 27 September 2024)
Research Articles
Carbon stock in Kolli forests, Eastern Ghats (India) with emphasis on aboveground biomass, litter, woody debris and soils
vol. 4, pp. 61-65 (online: 05 April 2011)
Research Articles
Estimating biomass and carbon sequestration of plantations around industrial areas using very high resolution stereo satellite imagery
vol. 12, pp. 533-541 (online: 12 December 2019)
Research Articles
Heuristic forest planning model for optimizing timber production and carbon sequestration in teak plantations
vol. 10, pp. 430-439 (online: 24 March 2017)
Research Articles
Estimation of above-ground biomass and sequestered carbon of Taurus Cedar (Cedrus libani L.) in Antalya, Turkey
vol. 6, pp. 278-284 (online: 01 July 2013)
iForest Database Search
Search By Author
Search By Keyword
Google Scholar Search
Citing Articles
Search By Author
Search By Keywords
PubMed Search
Search By Author
Search By Keyword