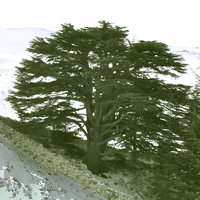
Estimation of above-ground biomass and sequestered carbon of Taurus Cedar (Cedrus libani L.) in Antalya, Turkey
iForest - Biogeosciences and Forestry, Volume 6, Issue 5, Pages 278-284 (2013)
doi: https://doi.org/10.3832/ifor0899-006
Published: Jul 01, 2013 - Copyright © 2013 SISEF
Research Articles
Abstract
Based on data obtained from 36 sample trees, above-ground biomass development of Taurus Cedar was modeled according to tree components on a single-tree basis. Carbon concentrations of tree components were established with the help of samples taken from sample trees. The biomass and sequestered carbon were modeled from the standing stem volume of single trees. It was determined that a coefficient of 0.51 could be used for Taurus Cedar species as a conversion factor from fresh weight to dry weight. Carbon concentrations were found to be lowest in branch barks, with a ratio of 49.5%, and highest in needles, with a ratio of 52.8%. According to the results of a comparison between volume, biomass and stored carbon amounts, 70.27 tons of biomass and 35.56 tons of carbon are stored in each standing stem volume of 100 m3.
Keywords
Above-ground Biomass, Carbon Concentration, Carbon Storage, Cedrus libani L., Stem Volume
Introduction
The use of fossil fuels has determined an increase in the CO2 concentration of the atmosphere, causing the global greenhouse effect. According to the United Nations Framework Convention on Climate Change (UNFCCC) and its Kyoto Protocol (KP), forest ecosystems may contribute toward reducing human-induced greenhouse effect ([36]).
It is well-known that any increase in the level of atmospheric carbon dioxide and other greenhouse gases also increases atmospheric temperature. Carbon dioxide is the most greenhouse gas with the greatest effect, and the steady increase in the amount of carbon dioxide in the atmosphere may be attributed to the use of fossil fuels and deforestation throughout the world ([26]).
Forest ecosystems play a critical role in reducing the greenhouse effect and stabilizing climate by storing atmospheric carbon dioxide as biomass ([8], [5], [25]).
In order to understand the carbon sequestration process and carbon cycle, it is necessary to obtain data on tree biomass. On the other hand, because carbon is becoming a valued product on the global market, estimating the amount of carbon stored in growing trees and harvested wood is also important ([22]). The determination of tree biomass is a challenging, time-consuming and costly process due to operations such as the cutting, uprooting, drying, and weighing of tree matter. Alternative techniques have been developed for the estimation of biomass from easily measured tree characteristics. Within the literature, the estimation of biomass values has generally used allometric equations. Allometry is the relationship between above-ground biomass and diameter at breast height and/or total height, below-ground biomass and diameter at breast height and/or total height, and above-ground biomass and below-ground biomass ([35], [13]). In former studies, scientists have frequently used allometric models for assessing above-ground and below-ground biomass ([27], [31], [3], [18], [29], [32], [2], [21]). Correspondingly, recent studies in Turkey have used allometric relationships to estimate the above-ground biomass for common tree species ([9], [10], [11]). These studies allow the estimation of above-ground biomass according to stem, branch, and leaf components; however, without additional evaluations, such techniques do not enable the estimation of the amount of bark and above-ground biomass, which are commercially valuable and thus removed from the forest during harvest, as well as those with no commercial value, that are left in the forest. Furthermore, there are a limited number of studies on the carbon contents of tree components that may be used for the estimation of the carbon storage capacity of forest ecosystems in Turkey.
This study focuses on Taurus Cedar, a native species of Turkey spread along the Taurus Mountains between the elevations of 800 and 2100 m ([16], [6]). In Turkey, a total of 99 325 hectares of pure Taurus Cedar stands exist, 67 850 of these hectares are productive and 31 475 are unproductive ([37]).
This study examined the following: (1) the determination of the commercially valuable above-ground biomass that has been removed from the forest during harvest as well as that with no commercial value, which has been left; (2) the determination of the carbon content of above-ground tree components; and (3) the development of appropriate models for the conversion of standing stem volume to biomass and stored carbon values of above-ground tree components.
Materials and Methods
Study area
Sample trees were randomly selected in pure stands of Taurus Cedar located within the boundaries of the Department of Forestry of Elmali (Antalya) (29º 56’-30º 04’ E - 36º 33’-36º 36’ N), where Taurus Cedars grow very successfully. Although this species spreads across the Taurus mountains, its expansion outside the Antalya region is partial and only in small areas. The best growing area and widest expansion of Taurus Cedar is in the Antalya region. In addition, Taurus Cedar is a very valuable species, and permission for tree cutting is generally very hard to obtain. Therefore, data was collected from Elmali (Antalya) district and primarily reflects the state of trees growing on that region. A typical Mediterranean climate prevails in the area of this study: summers are hot and dry, and winters are warm with high rainfall. According to meteorological data, the annual average temperature is 12.9 °C, average annual precipitation is 428.4 mm, and average relative humidity is 55.25%. The elevation of the sampling sites was within the range of 1400 to 1770 m, and slopes varied between 10% and 45%. Sampling sites were largely within site class III and partially within site class II.
Taurus Cedar distribution in Turkey and Antalya Regional Forest Directorate are shown in Fig. 1.
Experimental data
Single trees from pure Taurus Cedar stands in different development phases were analyzed in order to determine above-ground biomass development. A total of 36 sample trees were measured in various diameter and height groups. Some characteristics of sample trees are shown in Tab. 1. As forest stands in Turkey are defined according to tree species, diameter and canopy closure, the principle for determining the biomass development as a function of diameter or of diameter and tree height, rather than age function, was adopted in order to provide a practical means of assessing biomass and energy potential.
Tab. 1 - Some characteristics of the trees sampled in this investigation.
Sample no |
DBH (cm) |
Height (m) |
Site class |
Altitude (m) |
Exposure |
---|---|---|---|---|---|
1 | 16 | 12.7 | 3 | 1650 | SW |
2 | 29 | 17.7 | 3 | 1470 | N |
3 | 20 | 12.2 | 3 | 1518 | N |
4 | 14 | 8.85 | 3 | 1645 | W |
5 | 12 | 8.79 | 3 | 1580 | W |
6 | 43 | 20.65 | 2 | 1450 | NW |
7 | 33 | 19.23 | 2 | 1473 | N |
8 | 26 | 14.48 | 3 | 1490 | W |
9 | 17 | 10.15 | 3 | 1470 | N |
10 | 8 | 6.52 | 3 | 1408 | NE |
11 | 13 | 9.66 | 3 | 1540 | NW |
12 | 11 | 9.02 | 3 | 1550 | N |
13 | 18 | 9.05 | 3 | 1640 | NW |
14 | 20 | 14.2 | 3 | 1526 | N |
15 | 19 | 12.95 | 3 | 1510 | NE |
16 | 21 | 12.15 | 3 | 1512 | N |
17 | 30 | 17.38 | 3 | 1548 | NE |
18 | 23 | 18.23 | 3 | 1602 | N |
19 | 32 | 19.6 | 2 | 1430 | NW |
20 | 9 | 8.05 | 3 | 1416 | N |
21 | 10 | 9.92 | 3 | 1425 | N |
22 | 15 | 12.35 | 3 | 1730 | SW |
23 | 24 | 13.81 | 3 | 1547 | NW |
24 | 25 | 15.33 | 3 | 1510 | NW |
25 | 28 | 15.96 | 3 | 1590 | NW |
26 | 22 | 13.95 | 3 | 1500 | NW |
27 | 19 | 12.49 | 3 | 1490 | E |
28 | 22 | 13.54 | 3 | 1770 | W |
29 | 20 | 11.85 | 3 | 1680 | N |
30 | 16 | 10.57 | 3 | 1720 | N |
31 | 32 | 17.42 | 3 | 1405 | N |
32 | 23 | 14.25 | 3 | 1388 | N |
33 | 14 | 10.54 | 3 | 1680 | NW |
34 | 18 | 11.45 | 3 | 1497 | E |
35 | 9 | 8.9 | 3 | 1424 | SW |
36 | 37 | 18.3 | 2 | 1479 | N |
Each sample tree was selected at random from those that had no damage, and cut very close to soil level after cleaning the surrounding area. The entire length of cut trees and the diameter at breast height (to the nearest mm and bidirectional) were measured. The branches of the cut sample trees were then removed from the stem, weighed and grouped as follows: (a) thinner than 4 cm (non-commercial); (b) thicker than 4 cm (with commercial value). Branch samples at average thickness were taken from branch groups thinner than 4 cm and thicker than 4 cm. In addition, needle samples were taken with shoots. The stem was divided into 2.05-m sections, and the diameters of sections at both ends and the root collar diameter and height of the end piece were measured to determine the stem volume by using Smalian’s formula. Each stem section was weighted, and 5-cm-thick stem samples were taken from the middle of these sections. All samples were then labeled and preserved in plastic bags.
Stem, branch and needle samples were brought to the laboratory; needles were separated from the shoots; bark was separated from the wood; and fresh weights were determined. Samples were first air-dried, then oven-dried at 65 ± 3 °C until the weight stabilized, and the final dry weights were determined.
Modeling the above-ground biomass values
The biomass of tree components, such as the stem, branches, leaves, bark, coarse root and fine root, were generally estimated using different allometric regression models, based on DBH or DBH-H ([1], [15], [24], [28], [33], [34], [38]). The present study tested different models in determining biomass as a function of DBH or DBH and H. Appropriate functions were chosen and used in the estimation of biomass.
Measuring carbon concentrations
The composition of vegetation carbon (C) is found by applying a carbon conversion factor to dry weight ([14]). According to previous studies, the value of this factor varies between 43.7 and 55.7%, and a deviation of 10% may occur in calculations ([19], [12], [20], [4], [39]). As the size of deviation may be large, it would be beneficial to reduce the uncertainties in the calculation of biomass carbon components. In calculating the carbon cycle of forest ecosystems in Turkey, generally accepted factors for the conversion of biomass to carbon were used. As these factors may show a considerable degree of variation, the determination of carbon concentrations of tree components for common tree species is of utmost importance.
Dried samples were first weighed, then divided into small pieces, and converted into powder as appropriate for carbon analysis. Samples were dried again in order to prevent the effect of moisture, and carbon contents were determined via a CN analyzer as the amount of C for a dry weight of 100 g (%).
Checking the compliance of models
During the determination of the most appropriate functions, five different compliance measures were utilized. Calculations were made by using MS Excel. These measures were as follows: coefficient of determination (R2), standard error of estimate (SE), total error [TE (%)], mean deviation (Dmean), and absolute mean deviation (|Dmean|). Average difference, average absolute difference, standard error, total error and average absolute error values should be small and the coefficient of determination value should be large in order to obtain a reliable model; however, a function that provides reliable results according to one or more of these values may give inconsistent results according to other variables. In such situations, a “success range”, comprising all of the measured values, should be prepared in place of comparing biomass functions according to measure values ([30]). All of these measures were taken into consideration in the selection of appropriate models in this study.
Results
To determine single-tree aboveground biomass amounts, the most suitable allometric models were chosen in accordance with compliance measures. These models are given in Tab. 2.
Tab. 2 - Best-fitting models and their fitting statistics obtained using the diameter at breast height (d1.30) as predictor variable. (S): stem biomass; (SB): stem bark biomass; (CB): commercial branch biomass; (CBB): commercial branch bark biomass; (NB): non-commercial branch biomass; (NBB): non-commercial branch bark biomass; (T): twig biomass; (N): needle biomass; (TC): total crown biomass; (WT): whole tree biomass.
Single-Tree Biomass Equations | R2 | F | SE | TE (%) | Dmean | |Dmean| | eqn. |
---|---|---|---|---|---|---|---|
S = -31.0516 + (0.303619 d1.302) | 0.93 | 430 | 34 | 0.00022 | 0.00027 | 27.57 | 1 |
SB = -0.71530 + (0.056879 d1.302) | 0.9 | 312 | 7.5 | -0.000095 | -0.000027 | 5.15 | 2 |
CB = -34.7618 + (1.974415 d1.30) | 0.81 | 78 | 6.6 | -0.00013 | -0.000022 | 4.81 | 3 |
CBB = -14.5495 + (0.828923 d1.30) | 0.84 | 95 | 2.5 | 0.00018 | 0.000013 | 1.87 | 4 |
NB = 9.692722 + (-1.1675 d1.30) + (0.046302 d1.302) | 0.88 | 119 | 3.5 | 0.00157 | 0.000139 | 2.54 | 5 |
NBB = 9.999136 + (-1.22839 d1.30) + (0.041916 d1.302) | 0.88 | 116 | 2.7 | -0.407 | -0.0234 | 1.92 | 6 |
T = -0.27283 + (0.013135 d1.302) | 0.83 | 163 | 2.4 | -0.0035 | -0.00024 | 1.47 | 7 |
N = 0.817584 + (0.019014 d1.302) | 0.84 | 177 | 3.4 | 0.00218 | 0.000229 | 2.36 | 8 |
TC = 20.73819 + (-3.36526 d1.30) + (0.186172 d1.302) | 0.95 | 340 | 10 | -0.00017 | -0.000075 | 6.8 | 9 |
WT = 37.21449 + (-8.08322 d1.30) + (0.644812 d1.302) | 0.96 | 360 | 42 | 0.00007 | 0.000138 | 27 | 10 |
Above-ground biomass equations
The models using the diameter at breast height (d
1.30) as an independent variable were tested, and those providing the best goodness-of-fit were determined based on their compliance statistics. Within the biomass equations, the following units of measurement were used: oven-dry weight (kg); diameter at breast height (d
, cm); and tree height (h
, m). The best fitting models obtained (eqns. 1 to 10) are shown in Tab. 2.
The models that use diameter at breast height (d
1.30) and tree height (h
) as independent variables were tested, and those showing the best goodness-of-fit were determinined nased on their fit statistics (eqns. 11 to 20 - Tab. 3).
Tab. 3 - Best-fitting models and their fitting statistics obtained using the diameter at breast height (d1.30) and tree height (h) as predictors. Labels are as in Tab. 2.
Single-Tree Biomass Equations | R2 | F | SE | TE (%) | Dmean | |Dmean| | eqn. |
---|---|---|---|---|---|---|---|
S = 18.18743 + (-8.10728 d1.30) + (0.06557 h) + (0.3364 d1.302) + (0.571319 h2) |
0.95 | 148 | 29.5 | 0.00006 | 0.00007 | 17.6 | 11 |
SB = 8.265324 + (-0.33769 d1.30) + (-1.90362 h) + (0.036965 d1.302) + (0.18222 h2) |
0.93 | 93 | 6.9 | -0.00013 | -0.00004 | 4.3 | 12 |
CB = 236.1674 + (-19.7481 d1.30) + (1.348633 d1.30 h) + (0.389027 d1.302) + (-16.9361 h) + (-0.02394 d1.302) |
0.91 | 29 | 5 | -0.34 | -0.05 | 3.1 | 13 |
CBB = 106.0697 + (-9.62108 d1.30) + (0.578656 d1.30 h) + (0.216057 d1.302) + (-6.80524 h) + (-0.01174d1.302 h) |
0.93 | 36 | 1.9 | -0.36 | -0.025 | 1.2 | 14 |
NB = - 26.5548 + (1.808129 d1.30) + (-0.283 d1.30 h) + (-0.00297 d1.302) + (3.55587 h) + (0.00478 d1.302 h) |
0.92 | 66 | 3 | 0.041 | 0.0036 | 1.9 | 15 |
NBB = -25.8203 + (2.4104 d1.30) + (-0.26829 d1.30 h) + (-0.04056 d1.302) + (2.880058 h) + (0.00566 d1.302 h2) |
0.94 | 92.8 | 1.98 | -0.0117 | -0.00066 | 1.37 | 16 |
T = 1.05288 + (-0.20535 d1.30) + (-0.00673 h) + (0.013945 d1.302) + (0.014775 h2) |
0.84 | 39 | 2.5 | 0.0049 | 0.00032 | 1.5 | 17 |
N = -11.6569 + (2.584704 d1.30) + (-0.07867 d1.30 h2) + (-0.08286 d1.302) + (0.004274 d1.302 h) |
0.86 | 49 | 3.2 | 0.0113 | 0.0011 | 2.3 | 18 |
TC = 8.978191 + (-1.4041 d1.30) + (0.0333 d1.30 h) + (0.044973 d1.302) + (0.004129 d1.302 h) |
0.96 | 179 | 10.4 | -0.00587 | -0.00263 | 6.3 | 19 |
WT = 51.38543 + (-12.2998 d1.30) + (-2.75361 h) + (0.543984 d1.302) + (0.896138 h2) |
0.97 | 264 | 35.1 | -0.00001 | -0.00002 | 21.1 | 20 |
Single entry volume equations
For forestry practice in Turkey, stands within a forest ecosystem are classified according to tree species, diameter class, and canopy closure. Standing stock is expressed as barked stem volume. In the determination of how much C is sequestered in particular stands, biomass values of single tree components are first computed by biomass models for the related tree species, using median stand diameter values or median stand diameter - median stand height values. The resultant value is multiplied by the number of trees per hectare, and thus the total biomass of the stand is found. Such procedures generally complicate the calculation process. The process may be facilitated considerably by the estimation of stand biomass from standing stem volumes.
In order to model the relationship between standing stem volume and biomass and carbon storage capacities, a volume equation is required. For forestry practice in Turkey, standing stem volumes are determined according to diameter at breast height. Therefore, the function of volume was determined on the basis of diameter at breast height. For this purpose, various models were checked according to compliance criteria, and the following model was adopted:
(R2 = 0.977) where V
is the stem volume (m3) and d
1.30 is the diameter at breast height (cm).
Carbon concentrations of tree components
Determining the carbon content of tree components was achieved using samples from 36 sample trees. To produce usable carbon determination samples, dried samples of all tree components from 36 sample trees were divided into small pieces and then converted into powder as appropriate for carbon analysis.
Carbon contents were determined via a CN analyzer as the amount of C (%) for a dry weight of 100 g. Carbon contents of components are shown in Tab. 4, as minimum, maximum and mean values.
Tab. 4 - Carbon concentrations of tree components.
Tree components | Min (%) |
Max (%) |
Mean (%) |
---|---|---|---|
Stem wood | 50.1 | 52 | 50.9 |
Stem bark | 50 | 52.8 | 51.1 |
Commercial branch | 50.3 | 53.2 | 50.9 |
Commercial branch bark | 48 | 50.6 | 49.5 |
Non-commercial branch | 50 | 51.7 | 50.6 |
Non-commercial branch bark | 48 | 51.9 | 49.5 |
Twig | 48.5 | 51.4 | 50.1 |
Needle | 51.6 | 54.1 | 52.8 |
Relationships between standing stem volume and biomass
Various models were tested in order to enable the determination of biomass amounts from standing stem volumes, and those that yielded the best results with regard to their goodness-of-fit were identified. The models (eqns. 21 to 30) enabling the determination of biomass amounts from standing stem volumes of single trees and stand basis are given in Tab. 5 along with the compliance criteria for these models.
Tab. 5 - Best-fitting biomass models and their statistics using the standing stem volume (V) as predictor. (S): stem biomass; (SB): stem bark biomass; (CB): commercial branch biomass, (CBB): commercial branch bark biomass; (NB): non-commercial branch biomass; (NBB): non-commercial branch bark biomass; (T): twig biomass; (N): needle biomass; (TC): total crown biomass; (WT): whole tree biomass.
Single-Tree Biomass Equations | R2 | F | SE | TE (%) | Dmean | |Dmean| | eqn. |
---|---|---|---|---|---|---|---|
S = -1.21525 + (439.8813 ) | 0.98 | 1660 | 34 | -0.0000095 | -0.0000067 | 10.1 | 21 |
SB = 5.066058 + (81.7774 ) | 0.94 | 516 | 33 | -0.0000012 | -0.00000033 | 3.86 | 22 |
CB = -2.04429 + (44.42235 ) | 0.78 | 64 | 18 | -0.000023 | -0.0000022 | 2.76 | 23 |
CBB = -0.84186 + (18.71417 ) | 0.82 | 80 | 18 | 0.0000471 | 0.0000019 | 1.03 | 24 |
NB = -0.0987 + (31.36708 ) | 0.83 | 163 | 33 | 0.000041 | 0.0000035 | 2.28 | 25 |
NBB = -0.96709 + (23.2273 ) | 0.77 | 111 | 33 | -0.000018 | -0.000001 | 1.96 | 26 |
T = -1.351584 + | 0.78 | 117 | 34 | -0.000014 | -0.25 | 1.41 | 27 |
N = 3.02316 + (26.43525 ) | 0.82 | 152 | 34 | -0.000011 | -0.181 | 2.51 | 28 |
TC = -1.58508 + (164.3801 ) | 0.93 | 473 | 34 | -0.000012 | -0.536 | 7.73 | 29 |
WT = 1.641103 + (686.902 ) | 0.99 | 2558 | 34 | -0.0000025 | -0.0000049 | 13.39 | 30 |
Relationships between standing stem volume and carbon
For forestry practice in Turkey, it is required that the amount of sequestered carbon to be determined from the standing stem vo- lume. Therefore, models were established that enable the determination of sequestered carbon amounts considering the values of standing stem volume. These models (eqns. 31 to 40) and relevant compliance criteria are given in Tab. 6. Relations between standing stem volume and tree components are shown in Fig. 2.
Tab. 6 - Best-fitting carbon models and their fitting statistics using the standing stem volume (V) as predictor. (S): stem carbon; (SB): stem bark carbon; (CB): commercial branch carbon; (CBB): commercial branch bark carbon; (NB): non-commercial branch carbon; (NBB): non-commercial branch bark carbon; (T): twig carbon, (N): needle carbon; (TC): total crown carbon, (WT): whole tree carbon.
Single-Tree Carbon Content Equations | R2 | F | SE | TE (%) | Dmean | |Dmean| | eqn. |
---|---|---|---|---|---|---|---|
S = -1.55537 + (226.4176 ) | 0.99 | 3219 | 26 | -0.000009 | -0.0000052 | 3.74 | 31 |
SB = 2.2043 + (44.88568 ) | 0.93 | 322 | 25 | -0.000026 | -0.0000035 | 1.97 | 32 |
CB = -2.48641 + (25.50714 ) | 0.87 | 87 | 13 | 0.0000338 | 0.00000137 | 1.02 | 33 |
CBB = -1.0229 + (10.99776 ) | 0.87 | 83 | 13 | -0.000097 | -0.0000017 | 0.42 | 34 |
NB = 0.739696 + (11.8609 ) | 0.76 | 78 | 25 | 0.0000091 | 0.00000033 | 1.059 | 35 |
NBB = 0.397799 + (7.15052 ) | 0.82 | 110 | 25 | 0.0000134 | 0.00000029 | 0.515 | 36 |
T = 1.184463 + (5.734686 ) | 0.71 | 64 | 26 | -0.0000028 | -0.00000007 | 0.716 | 37 |
N = 2.2186 + (10.94115 ) | 0.7 | 60 | 26 | 0.0000049 | 0.00000025 | 1.31 | 38 |
TC = 1.282092 + (71.20486 ) | 0.92 | 283 | 26 | 0.0000039 | 0.00000074 | 3.35 | 39 |
WT = 1.518083 + (343.1626 ) | 0.99 | 4136 | 26 | 0.0000023 | 0.00000199 | 4.53 | 40 |
Discussion
Mass-based carbon concentrations are widely used for the conversion of biomass to the amount of stored carbon. A study by Zhang et al. ([39]) found the average amount of carbon in the stem to be 49.9% ± 1.3 (mean ± SE) for 10 different species, varying between 43.7 and 55.6% according to species. A study by Lamlom & Savidge ([20]) of 41 species reported this value to be in the range of 46.3 to 55.2%. The generally accepted method is to determine the amount of stored carbon by multiplying the total dry weight of trees by a coefficient of 0.5 ([26]). In the present study, the carbon content of stem wood was found to be an average of 50.9%. Carbon concentrations were found to be lowest in branch barks (49.5%) and highest in needles (52.8%). When carbon concentrations are evaluated as a whole, it can be seen that these values are quite close to the generally accepted level of 50% ([7]).
McPherson et al. ([23]) conducted a literature review on the conversion of fresh biomass to dry biomass and adopted an average coefficient of 0.56 for deciduous trees and 0.48 for coniferous trees. According to the results of the present study, the conversion factor from fresh weight to dry weight for Taurus Cedar was calculated as an average of 0.51 for above-ground components. This coefficient is higher than that predicted for coniferous species.
One of the main aims of the study is to determine the amounts of commercial and non-commercial parts of Taurus Cedar. Non-commercial parts are left to forests and decompose within a few years. Thus, for long-term carbon cycle forecasting, it is also necessary to determine the amount of parts left. According to the study’s findings, 15.5% of a Taurus Cedar tree is left to forest on average. This ratio vary 1% maximum between young and old trees.
The study also offers a way to estimate the sequestered biomass and carbon amounts by using the standing stem volume variable. The results of the study reveal that 70.27 tons of biomass and 35.56 tons of carbon are stored for 100 m3 of standing stem volume.
The change of total single-tree biomass amounts estimated for some tree species in Turkey according to DBH is seen in Fig. 3. As can be seen, beech has the highest single tree weight value according to DBH, and black pine has the lowest. It is observed that Taurus Cedar has the second highest single tree oven-dried weight compared with scots pine, black pine, beech, oak and chestnut.
Fig. 3 - Biomass changes according to diameter at breast height in single trees according to species.
Conclusions
In order to accurately determine the amount of carbon sequestered in forests, it is more appropriate to conduct an individual study of each species, rather than basing calculations on non-specific conversion factors. As seen in the literature, carbon concentrations differ considerably according to various tree species and components.
For forestry practice in Turkey, stands are defined according to tree species, tree diameter class and canopy closure. Tree diameter classes are termed “development ages” and represent a considerably wider range of diameters. Therefore, it is impossible to utilize biomass and carbon models that are based on tree diameter or height alone by only using data in the management plan. Therefore, additional studies are required. The results of the present study make it possible to attain above-ground biomass and sequestered carbon values safely and without any auxiliary operation by using the standing stem volume, which is the most practical element in management plans. Using present models, it is also possible to estimate the above-ground biomass, the amount removed from the forest (commercially valuable), and the amount left to the forest (no commercial value).
Within the scope of this study, above-ground modeling was performed, whereas no study of below-ground carbon sequestration capacities was conducted due to lack of study opportunities. If these shortcomings are addressed in future studies, a major knowledge gap will be filled.
References
Gscholar
Gscholar
Gscholar
Gscholar
Gscholar
CrossRef | Gscholar
Gscholar
Gscholar
Gscholar
CrossRef | Gscholar
Gscholar
Authors’ Info
Authors’ Affiliation
Istanbul University, Faculty of Forestry, 34473 Bahçeköy-Istanbul (Turkey)
Regional Forestry Directorate, 07000 Antalya (Turkey)
Corresponding author
Paper Info
Citation
Durkaya B, Durkaya A, Makineci E, Ülküdür M (2013). Estimation of above-ground biomass and sequestered carbon of Taurus Cedar (Cedrus libani L.) in Antalya, Turkey. iForest 6: 278-284. - doi: 10.3832/ifor0899-006
Academic Editor
Elena Paoletti
Paper history
Received: Nov 16, 2012
Accepted: Mar 19, 2013
First online: Jul 01, 2013
Publication Date: Oct 01, 2013
Publication Time: 3.47 months
Copyright Information
© SISEF - The Italian Society of Silviculture and Forest Ecology 2013
Open Access
This article is distributed under the terms of the Creative Commons Attribution-Non Commercial 4.0 International (https://creativecommons.org/licenses/by-nc/4.0/), which permits unrestricted use, distribution, and reproduction in any medium, provided you give appropriate credit to the original author(s) and the source, provide a link to the Creative Commons license, and indicate if changes were made.
Web Metrics
Breakdown by View Type
Article Usage
Total Article Views: 54127
(from publication date up to now)
Breakdown by View Type
HTML Page Views: 45459
Abstract Page Views: 2902
PDF Downloads: 4444
Citation/Reference Downloads: 32
XML Downloads: 1290
Web Metrics
Days since publication: 4161
Overall contacts: 54127
Avg. contacts per week: 91.06
Article Citations
Article citations are based on data periodically collected from the Clarivate Web of Science web site
(last update: Feb 2023)
Total number of cites (since 2013): 15
Average cites per year: 1.36
Publication Metrics
by Dimensions ©
Articles citing this article
List of the papers citing this article based on CrossRef Cited-by.
Related Contents
iForest Similar Articles
Research Articles
Stand structure and regeneration of Cedrus libani (A. Rich) in Tannourine Cedar Forest Reserve (Lebanon) affected by cedar web-spinning sawfly (Cephalcia tannourinensis, Hymenoptera: Pamphiliidae).
vol. 11, pp. 300-307 (online: 13 April 2018)
Research Articles
Tree biomass and carbon density estimation in the tropical dry forest of Southern Western Ghats, India
vol. 11, pp. 534-541 (online: 01 August 2018)
Research Articles
Effects of traditional forest management on carbon storage in a Mediterranean holm oak (Quercus ilex L.) coppice
vol. 11, pp. 344-351 (online: 18 April 2018)
Research Articles
Carbon stock in Kolli forests, Eastern Ghats (India) with emphasis on aboveground biomass, litter, woody debris and soils
vol. 4, pp. 61-65 (online: 05 April 2011)
Research Articles
Allometric relationships for predicting the stem volume in a Dalbergia sissoo Roxb. plantation in Bangladesh
vol. 3, pp. 153-158 (online: 15 November 2010)
Research Articles
Carbon storage in degraded cork oak (Quercus suber) forests on flat lowlands in Morocco
vol. 9, pp. 125-137 (online: 08 August 2015)
Research Articles
Allometric relationships for volume and biomass for stone pine (Pinus pinea L.) in Italian coastal stands
vol. 6, pp. 331-335 (online: 29 August 2013)
Research Articles
Effects of planting density on the distribution of biomass in a douglas-fir plantation in southern Italy
vol. 8, pp. 368-376 (online: 09 September 2014)
Research Articles
Estimating changes in soil organic carbon storage due to land use changes using a modified calculation method
vol. 8, pp. 45-52 (online: 17 June 2014)
Research Articles
Allometric models for estimating biomass, carbon and nutrient stock in the Sal zone of Bangladesh
vol. 12, pp. 69-75 (online: 24 January 2019)
iForest Database Search
Search By Author
Search By Keyword
Google Scholar Search
Citing Articles
Search By Author
Search By Keywords
PubMed Search
Search By Author
Search By Keyword