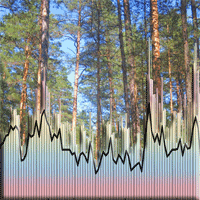
Growth patterns of Scots pine (Pinus sylvestris L.) under the current regional pollution load in Lithuania
iForest - Biogeosciences and Forestry, Volume 8, Issue 4, Pages 509-516 (2014)
doi: https://doi.org/10.3832/ifor1267-007
Published: Nov 12, 2014 - Copyright © 2014 SISEF
Research Articles
Collection/Special Issue: IUFRO 7.01.00 - Ilhéus (Brazil, 2013) & Beijing (China, 2014)
Forest Response to Climate Change and Air Pollution
Guest Editors: Paoletti E, Kozovitz A, Feng Z, Bytnerowicz A
Abstract
The belief that trees have begun growing more rapidly in recent years was examined in Scots pine (Pinus sylvestris L.) forests, a dominant forest type in Lithuania. The largest, pre-dominant pine trees, with a diameter at breast height exceeding 50 cm, were selected for analysis in this study; these were growing in three experimental overly-mature stands located in different parts of Lithuania (north-eastern, western and seaside). We hypothesized that if the annual tree increment has increased in recent years, then the largest trees in the stand should regularly demonstrate this characteristic first of all. The data collected for this study confirmed that since the 1980 growing season the annual increment of the pine trees analysed here has increased. The causes of this rapid growth were higher air temperatures during the dormant period and, to a lesser extent, the higher temperatures from May through August. The effect of precipitation was negligible. A 30-year long data set on acidifying pollutants allowed us to detect significant effect of reduced SO2 concentration and sulphur deposition as well as gradually increased ammonia deposition on the increased annual basal area increment of pine trees over the last 30 year long period. Multiple regression analysis indicated that meteorological parameters can explain up to 50% of the observed increase in the growth rate of Scots pine in Lithuania; meanwhile the presence of acidifying species can account for an additional 30%. However, the pollution data set (20-30 years) was insufficiently long to be compared with the meteorological data. Therefore we were unable to distinguish whether the recent decrease in pollution or global warming resulted in the increases in tree growth rates more significant.
Keywords
Scots Pine Growth, Pre-dominant Trees, Climate Change, Acidifying Species
Introduction
Annual tree-rings provide a valuable source of environmental information and have been widely used throughout the world to document past forest conditions ([36]). Parameters related to the annual stem increment are extremely reliable indicators of general forest health and stability ([17]). Tree rings provide unique possibilities for the retrospective assessment of growth rates over an extended period of time ([29]). Modern tree-ring analysis methods were developed at the beginning of the 1980s, when forest damage was recognized as a serious and widespread regional problem ([20], [15], [16], [28], [47]). However, a few years later an apparently opposite view on Europe’s forest conditions was presented by Spiecker et al. ([49]), based on 22 growth studies from 12 countries. The results revealed a considerable increase in forest growth over central Europe ([48]).
Many potential causes for the increase in net primary productivity have been proposed, even though forest health was simultaneously deteriorating. The relative importance of different factors has been difficult to assess because of a multitude of interacting stresses affecting trees throughout their lifetimes ([35]). Despite this, the main factors for the enhanced growth rates were attributed to increases in the photosynthetic rate, the length of the growing season and the leaf area index ([26], [11]). Meteorological variation had a major response to these changes ([10], [27], [49], [44]).
Our earlier investigations revealed that acidifying pollutants mainly resulted in changes in the rate of tree crown defoliation vs. tree stem increment when the effect of crown defoliation on increment was considered ([4]).
In this study, we attempted to detect the effects of acidifying pollutants on stem increment after taking into account the effect of meteorological parameters. Therefore, this study aimed to analyse recent changes in meteorological variables, air pollution, and acid deposition to identify the cause of changes in the stem annual increment of over-mature, pre-dominant healthy pine trees.
Material and methods
In 2010 we assessed tree conditions within national parks (NP) in different regions of Lithuania: Curonian Spit, Zemaitija and Aukštaitija NPs. These three parks are located at a seaside site, in western and north-eastern Lithuania, respectively (Fig. 1).
A hundred of predominant pine trees were selected matching the following characteristics: age exceeding 120 years, diameter at breast height larger than 50 cm, upper crown taller than the general level of the canopy, no visible crown or stem damage, healthy status (defoliation 0-15 %). Moreover, trees were selected within permanent observation plots already monitored by different methodologies: in Curonian Spit NP plots were established based on UN ECE Forest monitoring Level 1 methodology, while in Aukštaitija and Zemaitija NP based on local methodology, as described in details in earlier publications ([7]). All monitored stands represent Pinetum vacciniosum and Pinetum vacciniosum-myrtiliosum forest types which are prevailing in Lithuania. The position of largest and tallest trees, ove-topping the stand canopy allowed stand density to be ignored, since competition with neighboring trees are minimal for these trees. Forty series of tree ring width from 5 overmature permanent observation pine (POS) stands in Aukštaitija NP, 30 series from 3 POS in Zemaitija, and 30 from 10 POS in Curonian Spit NP were used to detect regional peculiarities of the effect of meteorological parameters on pine growth, as well as the effect of acidifying species in the air, their deposition and surface ozone over the last 30 year period. Such a number of monitored trees and their mean values in each NP allowed to detect statistically significant regional differences in tree ring width variations and their reactions to the integrated effect of meteorological parameters in conjunction with air pollutants and acid deposition.
A standard dendrochronological technique was used to assess tree growth rates. Radial growth was assessed by measuring the width of annual tree-rings in stem cores. Each ring was measured to the closest 0.01 mm using an electronic transducer and a binocular scope fixed over the moving stage of “Lintab6” equipment. To eliminate the effect of age on pine radial increment, we computed the stem basal area increment (BAI). For each POS, a BAI chronology was computed by averaging the BAI for each year across all trees sampled at that POS.
Because tree growth rate is strongly affected by stand density ([9]), we analyzed only the largest diameter, pre-dominant, healthy trees ([24], [19], [46]). This allowed to minimize the effects of competition and simplified cross-dating due to their few missing rings ([24], [19], [46]). Cross-dating analysis among tree ring series was carried out based on detected years of exclusively high (1905, 1949, 1975, 1990, 2008) and low (1902, 1940, 1979-80, 1992, 2006) increment rate.
Pearson correlation analysis was used to examine the relationship between radial growth and climate. Multiple regression models were applied to assess the integrated effect of meteorological parameters (mean air temperature and monthly precipitation rates) on pine stem annual increment, as well as the effect and significance of air pollutants and their deposition. All statistical analyses were carried out using the software package STATISTICA® 7.0 (StatSoft Inc., Tulsa, OK, USA).
We evaluated the effects of meteorology over a period of 60 years to detect key parameters responsible for the intensive growth of pine trees. This time period was split into two 30-year periods: the increment decreased during the first 30-year period (natural growth) and increased during the second 30-year period (affected growth). Equal lengths of time periods allowed to detect key meteorological parameters responsible for the “V” form tree growth.
Meteorological parameters collected from September to August for the last two preceding and the current seasons were used in the correlation analysis. Significance of acidifying pollutants and surface ozone was tested on the residuals of annual stem basal area increment, after subtracting the effect of key meteorological parameters by multiple regression analysis. The effect of pollutants, including surface ozone, on the natural reduction of pine increments from 1950 to 1980 could not be tested due to a lack of data. Predicting variables were included in the regression model by a stepwise method. To assess the goodness-of-fit of the models, the coefficient of determination R2 and its significance (α=0.05) were considered.
Meteorological parameters as well as data related to the airborne concentration of acidifying pollutants, their deposition rates and surface ozone were obtained from the Aukštaitija and Zemaitija integrated monitoring stations, as well as from the Preila European Monitoring and Evaluation Programme (EMEP) station in Curonian Spit NP. These data were presented in details in earlier studies ([5], [6], [8]).
Results
Growth patterns of pre-dominant and over mature healthy pine trees
Tree-ring width data of the monitored pine trees revealed a stable or slightly decreasing tendency in the basal area increment until 1980 (p>0.05), while significant trends of decreasing ring width were found for Aukštaitija, Zemaitija and Curonian Spit NPs by almost -0.003, -0.007, and -0.0176 mm/year (p<0.05), respectively. Such changes reflected natural pine growth related to tree aging and increasing bole diameter. After 1980, a significant increase in annual increment of stem basal area and ring width was recorded in Aukštaitija (33 mm2 and 0.016 mm, respectively), Zemaitija (24 mm2 and 0.015 mm) and Curonian Spit NPs (25 mm2 and 0.013 mm - Fig. 2).
Changes mean annual temperature and precipitation in NP
Long-term data analysis of mean annual temperature and precipitation revealed a decrease of both the above parameters moving from the coast towards inner Lithuania. Mean annual temperature over 60 years in Curonian Spit NP (seaside) was 7.5 °C, while it was 6.2 and 6.1 °C in western and eastern Lithuania, respectively. The trend for mean annual precipitation over the same period was slightly different: in Curonian Spit NP was 709.5 mm, in the western part increased up to 757.5 mm, while decreased to 652.2 mm in the east. Notwithstanding these differences, precipitation over 60 years tended to increase in the seaside by 3.3 mm year-1, by 2.9 mm year-1 in the western part and by approximately 1.8 mm year-1 in the eastern part of Lithuania (Fig. 3, Fig. 4). A similar pattern of regular increase was detected for mean annual temperature (3.2 °C year-1 in seaside and western part of Lithuania, 2.9 °C year-1 in the eastern part). All the trends of mean annual precipitation and temperature in the considered sites were statistically significant (p<0.05).
Fig. 3 - Variation in mean annual temperature (°C) and precipitation (mm), and their tendencies over the 1950-2010 period.
Fig. 4 - Variation of the mean monthly temperature and precipitation (B coefficient) over the 1950-1980 and 1981-2010 periods and their significance. (dotted line: p = 0.05).
The intensity of variation of mean monthly temperature over 1950-1980 and 1981-2010 periods revealed the same regular patterns described above in all the sites considered. Over the last period (1981-2010) mean monthly air temperature increased significantly in April, June, August and September up to 0.07-0.08 °C year-1. Instead, variation in precipitation over the same period was not obvious. Only increase in the rainfall of February in Curonian Spit and Aukštaitija NP, as well as increase in October and decrease in December in Aukštaitija NP were statistically significant. The effect of these meteorological parameters on pine growth was evaluated first in the study.
Effects of meteorology on pine tree growth
The effect of mean temperature on growth of Scots pine trees in Lithuania was more significant than that of precipitation (Fig. 5). Indeed, pine growth is limited by cold temperatures from September to April in the north-eastern part of Lithuania (Aukštaitija NP) and from December through April in the western and seaside parts (Zemaitija and Curonian Spit NP, respectively). In contrast, the effect of precipitation during the dormant period was more significant than that during the growing season in western and seaside part of Lithuania. In fact, only more abundant precipitation in June was positively correlated with an increased annual increment of Scots pine in Lithuania.
Fig. 5 - Relationship between meteorological parameters and tree annual basal area over the period 1950-1980 and 1981-2010 in the considered National Parks in Lithuania. In columns: the effect of precipitation on stem basal area increment. In lines: effect of temperature on stem basal area increment. Significance levels: p < 0.05 when r = 0.35 (black horizontal lines).
Correlation analysis revealed that in Aukštaitija NP the increased mean temperatures in winter over the period 1981-2010 had a significant positive effect on tree growth, while the observed decrease in precipitation by September (both the previous and the current seasons) showed a significant negative effect (Fig. 5).
The strong positive effect of the higher mean temperatures during the dormant (December to April) and the growth (June to July) periods are mainly responsible for the intensive growth of pine trees over the years 1981-2010 in Zemaitija NP. Analogously, the same two factors (along with the positive effects of increased precipitation in November and July) are mainly responsible for the intensive growth of trees over the same period in Curonian Spit NP (Fig. 5).
Based on the above data, it can be inferred that the recent enhanced growth of pine trees in Lithuania can primarily be attributed to warmer winters. Moreover, the effect of the reduction in precipitation on pine growth seems to be more significant over the dormant than over the vegetation period.
Approximately 52% of the variation in the basal area increment (Zq
, mm2) of pine trees analyzed in the Curonian Spit NP was accounted for by the precipitation of July-August of the previous seasons (Pr
-1VII-VIII, mm), and by mean temperatures of April (Tm
IV, °C), of the period from May through September of the previous season (Tm
-1V-IX, °C) and of December through March of the current season (Tm
XII-III, °C), according to the following model (R² = 0.522, F[4, 56] = 15.3, p < 0.0001, SEE = 327.9 - eqn. 1):
In the Zemaitija NP (western part of Lithuania), mean temperatures from December to April (Tm
XII-IV, °C) and from May to August of the previous and current seasons (Tm
-1V-VIII and Tm
V-VIII, respectively, °C) were responsible for the more intensive pine growth. These parameters accounted for about 41% of the variation in the annual basal area (Zq
, mm2) increment, according to the following model (R² = 0.415, F[4, 55]=9.74, p < 0.0001, SEE = 349.6 - eqn. 2):
Changes in temperature and precipitation also explained the variation of annual basal area (Zq
, mm2) in Aukštaitija NP. Growth rate changes were explained by the mean temperatures of July-August of two growing seasons (Tm
-1VII-VIII and Tm
VII-VIII, °C) and from December to March of the current season (Tm
XII-III, °C) along with the precipitation from July through August of the current year (Pr
VII-VIII, mm) and of September of the current and previous seasons (Pr
IX, and Pr
-1IX, respectively, mm), according to the following model (R² = 0.416, F[6, 54] = 6.54, p < 0.0001, SEE = 277.9 - eqn. 3).
However, the meteorological parameters considered in this analysis were not able to explain the peaks in stem growth in 2003 and 2007 in the Aukštaitija NP, and in 1990 in Zemaitija and Curonian Spit NPs (Fig. 6).
Fig. 6 - Data series of measured and simulated basal area, as well as residuals of tree stem basal area after subtracting the effect of meteorological parameters.
Changes in acidifying , their deposition and effect on radial increment
Analysis of the IMS (Integrated Monitoring Station) dataset revealed a significant decrease in pollutant load until the year 2000. The air concentration of SO2 at Aukštaitija IMS (LT-01) decreased by 82% (2.73 to 0.49 μgS m-3) and at Zemaitija IMS (LT-03) by 79% (2.22 to 0.47 μgS m-3). Thereafter, the concentration was stable at 0.5-1.0 μgS m-3. Air concentration of aerosolic SO42- changed in a similar way as SO2 air concentration.
The most significant decrease in ΣNH4+ air concentration lasted until 2001 and was 86% in LT-03 (8.55 to 1.15 μgN m-3) and 77% in LT-01 (4.44 to 1.02 μgN m-3). During the 2001-2005 period a stabilization of ΣNH4+ air concentration at 1.1-1.3 μgN m-3 in both LT-01 and LT-03 was observed. Annual means of ΣNO3- concentration in the air were stable at 0.5-0.7 μgN m-3 in all the stations considered.
Changes in annual wet deposition had a very similar pattern to that of the air. The wet deposition of sulphur for the period 1994-2000 at the Aukštaitija NP decreased by 58% (600 to 250 mgS m-2) and by 60% at the Zemaitija NP (750 to 300 mgS m-2). Over the period 2001-2010, sulphur deposition at LT-01 station further decreased up to 190 mgS m-2, and up to 280 mgS m-2 at LT-03.
A decrease in annual wet deposition of NH4+ from 492 to 198 mgN m-2 at LT-01 and from 537 to 303 mgN m-2 at LT-03 were observed until 2001. Afterwards, a gradual increase in deposition of NH4+ was observed in all the stations. Annual wet deposition values for NO3- ranged from 241 to 211 mgN m-2 at LT-01 and from 414 to 342 mgN m-2 at LT-03. Despite this, the total N deposition since 2001 started to increase again mainly due to the increase in ammonium depositions.
The dataset obtained from the Preila EMEP station in Curonian Spit NP showed higher pollution levels. A significant decreasing trend in the concentration of main acidifying pollutants in the air and in precipitation was detected until the year 2000 ([8]). Over this period, air concentrations of both sulphur compounds (SO2 and SO42-) decreased by approximately 75-80% (from 4.0 to 0.85 μgS m-3 for SO2 and from 3.0 to 0.83 μgS m-3 for SO42-). Sulphur concentration in precipitation decreased by 70% (2.5 mg l-1 to 0.8 mg l-1) and by 85% in wet deposition (2100 mg m2 to 310 mg m2 - Fig. 7). This significant decrease (p<0.05) in annual sulphur compounds was most likely the result of a reduction in SO2 emissions in Europe, including Lithuania.
Fig. 7 - Main acidifying pollutants in the air and precipitation, and their changes over the 30 year period. Source: Augustaitis et al. ([8]).
Data on NO3- and NH4+ in the precipitation and in wet deposition revealed that the highest concentration of ammonia was reached in the period 1987-1993 (1992: NH4+ 2.0 mgN l-1 and 1120 mgN m2, respectively). Afterward, these pollutants significantly decreased down to 0.6 mgN l-1 and 200 mgN m2 in the year 2000, that is, a reduction of 70% and 82%, respectively. Changes in NO3- deposition were minor, while some decrease in wet concentration and wet deposition was detected. Until 2000 NO3- concentration in precipitation decreased by 53% (1.3 to 0.6 mgN l-1) and wet deposition by 72% (700 to 200 mgN m2).
Between 2000-2007 a further decrease up to 0.26 and 0.40 μgS m3 was observed in SO2 and aerosol SO42- concentrations, respectively (Fig. 7). Those were the lowest values of this acidifying pollutants over the whole observation period. Afterward, a gradual increase in their air concentration has begun, reaching 0.31 μgS m3 and 1.02 μgS m3, respectively, in the year 2010. The same trend of variation was observed in the SO42- wet concentration and deposition data series.
The 30-year dataset obtained from the Preila EMEP station ([8]) allowed to detect a significant effect of the reduction in concentration of acidifying pollutants on the tree growth in Curonian Spit NP, according to the following model (R² = 0.390, F[3, 26] = 5.539, p = 0.0044, SEE = 260.6 - eqn. 4):
where SO42-dep is the annual sulphur deposition (mgS m-2), NH4+dep is the annual ammonia deposition (mgN m-2) and SO2 is the sulphur air concentration (μgS m-3).
Ammonium and sulphur deposition, along with the SO2 concentration in the air, accounted for about 40% of variation in the residuals of stem basal area increment after subtracting the effects of meteorological parameters in Curonian Spit NP. Such relationship was statistically significant (p<0.05). Overall, the combined effects of meteorological parameters and acid deposition on pine growth can explain up to 80% of the variation (p<0.05) in the recent growth of pre-dominant, over-matured, healthy pine trees analyzed in this study (Fig. 8). Notwithstanding, neither changes in air concentrations of acidifying pollutants nor their deposition fully explained the observed peaks in annual increment, which exceeded 2500 mm2.
Fig. 8 - Integrated effect of meteorological parameters and acidifying species on annual increment of pine stem basal area in Curonian Spit National Park.
No significant effects of acidifying pollutant concentration on variation in residuals of basal area increment were detected for Aukštaitija or Zemaitija NPs.
Discussion
In the traditional dendroecology, the effects of climate on tree growth are usually studied by response function analysis ([24]). Tree-rings, as an indicator of the radial growth of trees, are highly sensitive to climate change. Air temperature and moisture clearly influence forest conditions and growth, and both are predicted to change with changing climate ([23]). In northern Fennoscandia summer temperatures, especially mean temperatures of July, affect variations in growth rates ([13], [41]), while in central ([21]) and eastern Europe ([25], [35]) precipitation affects variations in tree growth rates.
In this study, we used the correlative method which has proven to be effective in the analysis of the response of forest growth to climate change ([14], [32], [50], [51]).
Our earlier investigations showed that temperatures of late winter (February), early spring (March, April) and those of late summer (August) mostly affect pine growth rates at our latitudes ([3], [4]). In some cases, temperatures of autumn months (September, October) of the previous year had a dramatic impact on tree radial increments. In contrast, the effect of precipitation was less pronounced ([29], [30]). The results obtained here are in full agreement with previous recent analyses in this area and revealed no significant negative effect of the meteorological parameters considered on pine increments.
State-of-the-art climatic reports clearly demonstrate that global warming is already occurring ([31]), leading to changes in forest growth by extending the growing season and increasing rates of photosynthesis, mainly at northern latitudes ([34], [51]). In such regions, growth is mainly limited by the availability of water in sandy soils under global warming conditions ([12]). However, in our study tree growth increment was positively correlated to warming in the dormant period. Evergreen conifers can photosynthesize all year around, and warm winters can increase the synthesis and storage of carbohydrates to be used in the next growing season ([33]). Indeed, this promoted the earlywood formation in spring when combined with abundant precipitation, which increases the water availability in the soil. ([40]).
Soil water shortage is a major limiting factor for tree growth ([40]), making trees growing on sites with lower water availability more sensitive to climatic variations ([33]). The lack of precipitation may be considered a limiting factor for tree growth at seaside in Lithuania, where forest stands are located on sandy soils.
The combined effects of increased atmospheric CO2 levels and elevated N deposition may also account for a 15-20% of the observed increase in forest net primary production ([45]). In this study, we examined the effect of acidifying pollutants (including N compounds) in combination with several environmental factors on the basal area increment of dominant, old-growth pine trees.
Results obtained in the Curonian Spit NP confirmed previous evidence about the positive effect of the load of N deposition on trees’ growth and health. This positive effect of ammonia is consistent with the hypothesis of enrichment/fertilization of poor forest soils by N pollutants. Plant species belonging to the same community may differ in their capacity to up-take different forms of N ([22], [39], [42]). Therefore, NH4+ or NO3- may alter the competition among plant species by favoring those capable of exploiting N depositions ([43]). However, a depression effect due to deposition in N saturated systems has been reported by several authors ([1], [2], [18]).
The results obtained in the Curonian Spit NP were also in full agreement with previous studies showing that the concentration of sulphur deposition negatively affects the growth and health of trees ([3], [5], [6], [4], [7], [29], [30]).
Similar results were expected for the other sites considered in this analysis. However, the limited dataset available (covering the last 15-17 years) may have hampered the detection of any significant effect of acidifying pollutants on pine growth. Indeed, studies at the monitoring stations in Aukštaitija NP started in 1994, and in Zemaitija NP in 1996.
No phytotoxic effect of surface ozone on tree increment was detected in the National Parks of Lithuania. However, assessment of the cause-and-effect related O3 risk on tree growth, study of the function of stomatal O3 influx, analysis of the yield of phytotoxically relevant O3 uptake over time are highly recommended ([37], [38]).
Further studies are needed to explain the negative effects of sulphur compounds on the pine increments at nutrient poor sites, as well as the positive effect of ammonia and the negative effect of more abundant precipitation in September. Moreover, the more intensive tree growth observed in recent years was detected only for tallest healthy trees over-topping the forest canopy. Whether this reflects a general trend for Scot pine in Lithuania is a matter deserving further investigations.
Conclusion
Main factors influencing the increased growth of pre-dominant healthy trees observed since the beginning of the 1980s are mainly meteorological factors. Specifically, air temperature of the winter period (December-April) and of the growing season (May-August) mostly affected pine tree growth. Such parameters accounted for about 50% of the variation in annual basal area increment of dominant pine trees. The effect of precipitation is less conspicuous, though its effect on pine growth seems more relevant over the dormant period. Higher rainfall in July and August enhances pine stem increment, particularly in the seaside part of Lithuania, where pines grow on sandy soils. No negative effect of higher air temperature during the growing season was observed.
A decrease in the concentration and deposition of sulphur together with the soil enrichment by N pollutants in the growing season boost the growth rate of dominant pine trees in Lithuania. However, it is worth to stress that our results concerned only the tallest healthy trees in the studied forests: the hypothesis that the growth of pine stands in the last 30 years is faster than before in Lithuania could not be confirmed based on the results of this investigation.
Acknowledgments
The study was carried out within the framework of the Lithuanian national project no. VP1-3.1-ŠMM-08-K-01-025 “Specific, genetic diversity and sustainable development of Scots pine forest to mitigate the negative effects of increased human pressure and climate change”, supported by the EU Social Fund.
References
Gscholar
Gscholar
Gscholar
Gscholar
CrossRef | Gscholar
Gscholar
Gscholar
Gscholar
CrossRef | Gscholar
CrossRef | Gscholar
Online | Gscholar
Online | Gscholar
Gscholar
Online | Gscholar
CrossRef | Gscholar
Gscholar
Authors’ Info
Authors’ Affiliation
Ingrida Augustaitiene
Gintautas Mozgeris
Aleksandras Stulginskis University, Akademia, LT-53362 Kaunas (Lithuania)
Center for Physical Sciences and Technology, LT-02300 Vilnius (Lithuania)
Corresponding author
Paper Info
Citation
Augustaitis A, Augustaitiene I, Mozgeris G, Juknys R, Vitas A, Jasinevičiene D (2014). Growth patterns of Scots pine (Pinus sylvestris L.) under the current regional pollution load in Lithuania. iForest 8: 509-516. - doi: 10.3832/ifor1267-007
Academic Editor
Silvano Fares
Paper history
Received: Feb 11, 2014
Accepted: Jul 30, 2014
First online: Nov 12, 2014
Publication Date: Aug 02, 2015
Publication Time: 3.50 months
Copyright Information
© SISEF - The Italian Society of Silviculture and Forest Ecology 2014
Open Access
This article is distributed under the terms of the Creative Commons Attribution-Non Commercial 4.0 International (https://creativecommons.org/licenses/by-nc/4.0/), which permits unrestricted use, distribution, and reproduction in any medium, provided you give appropriate credit to the original author(s) and the source, provide a link to the Creative Commons license, and indicate if changes were made.
Web Metrics
Breakdown by View Type
Article Usage
Total Article Views: 49761
(from publication date up to now)
Breakdown by View Type
HTML Page Views: 42316
Abstract Page Views: 2484
PDF Downloads: 3670
Citation/Reference Downloads: 19
XML Downloads: 1272
Web Metrics
Days since publication: 3662
Overall contacts: 49761
Avg. contacts per week: 95.12
Article Citations
Article citations are based on data periodically collected from the Clarivate Web of Science web site
(last update: Feb 2023)
Total number of cites (since 2015): 14
Average cites per year: 1.56
Publication Metrics
by Dimensions ©
Articles citing this article
List of the papers citing this article based on CrossRef Cited-by.
Related Contents
iForest Similar Articles
Editorials
COST Action FP0903: “Research, monitoring and modelling in the study of climate change and air pollution impacts on forest ecosystems”
vol. 4, pp. 160-161 (online: 11 August 2011)
Research Articles
A fast screening approach for genetic tolerance to air pollution in Scots pine field tests
vol. 6, pp. 262-267 (online: 01 July 2013)
Research Articles
The influence of age and crown position on growth efficiency along a Scots pine chronosequence
vol. 12, pp. 474-479 (online: 14 October 2019)
Research Articles
Impact of climate change on radial growth of Siberian spruce and Scots pine in North-western Russia
vol. 1, pp. 13-21 (online: 28 February 2008)
Technical Reports
Air pollution regulations in Turkey and harmonization with the EU legislation
vol. 4, pp. 181-185 (online: 11 August 2011)
Review Papers
Monitoring the effects of air pollution on forest condition in Europe: is crown defoliation an adequate indicator?
vol. 3, pp. 86-88 (online: 15 July 2010)
Research Articles
Identification and allelochemical activity of phenolic compounds in extracts from the dominant plant species established in clear-cuts of Scots pine stands
vol. 10, pp. 309-314 (online: 23 February 2017)
Research Articles
Scots pine’s capacity to adapt to climate change in hemi-boreal forests in relation to dominating tree increment and site condition
vol. 14, pp. 473-482 (online: 18 October 2021)
Research Articles
The growth dynamics of East European Scots pine (Pinus sylvestris L.) populations - a Lithuanian field trial
vol. 17, pp. 59-68 (online: 06 March 2024)
Review Papers
Impacts of climate change on the establishment, distribution, growth and mortality of Swiss stone pine (Pinus cembra L.)
vol. 3, pp. 82-85 (online: 15 July 2010)
iForest Database Search
Search By Author
Search By Keyword
Google Scholar Search
Citing Articles
Search By Author
Search By Keywords
PubMed Search
Search By Author
Search By Keyword