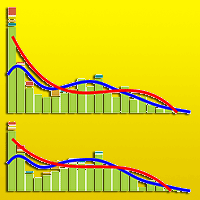
Patterns of forest dynamics in a secondary old-growth beech-dominated forest in the Jizera Mountains Beech Forest Reserve, Czech Republic
iForest - Biogeosciences and Forestry, Volume 12, Issue 1, Pages 17-26 (2019)
doi: https://doi.org/10.3832/ifor2702-011
Published: Jan 10, 2019 - Copyright © 2019 SISEF
Research Articles
Abstract
Restoring the structural characteristics of secondary old-growth forests that were previously managed is increasingly debated to help increase the area of more complex forests which provide a broader array of forest services and functions. The paucity of long-term data sets in Central Europe has limited our ability to understand the ongoing ecological processes required for effective restoration programs for old-growth forests. To address this, we used repeated census data from eight permanent plots to evaluate forest structural dynamics over a 12-year period in the largest complex of European beech (Fagus sylvatica L.) forests in the Czech Highlands without intensive forestry intervention for almost 50 years. Our results showed that previously managed forests can exhibit structural qualities typically associated with old-growth forests after management has ceased for a period. The stand structural characteristics (e.g., density of large and old trees) is comparable with protected reserves of old-growth European beech-dominated forests. The average stand age was 196 years, but the oldest tree was 289 years old. The annual mortality rate was 0.43% for all species, and the U-shaped distribution indicating size-dependent mortality is likely an important process that is balanced by the turnover of new tree recruitment. During the study period, we detected that the diameter distribution tended towards a rotated sigmoid distribution. The lasting effects of the most recent forest management are evident in the scarcity of dead wood, and a prolonged process of dead wood accumulation has begun. Thus, the abandonment of all management activities in near-natural forest reserves, including dead wood removal, will ensure that the forests will develop characteristics typical of old-growth forests.
Keywords
Dead Wood, Diameter Distribution, Fagus sylvatica, Forest Reserve, Forest Structure, Mortality, Old-growth Forest
Introduction
The term “old-growth” is usually used to describe forests in a late stage of successional development that are compositionally and structurally distinct from earlier successional stages ([19]). Structural components associated with old-growth forests include multilayered canopies, large variation in tree sizes, many large and old trees, standing dead trees, and large amounts of lying dead wood ([5]). Tree diameter distribution is also a representative structural feature often used to describe old-growth forests ([36]). The reverse J-shaped form has traditionally been considered indicative of old-growth forests in a state of equilibrium ([40], [36], [8]), a condition maintained by equal mortality rates among diameter classes across the entire range of diameters ([68]). However, other studies have indicated that other forms of diameter distributions, such as a rotated sigmoid curve ([21], [38], [2]), could also be characteristic of old-growth forests. A rotated sigmoid develops from rapid growth and low mortality in trees in the mid-range diameter classes sizes, which illustrates a U-shaped mortality distribution ([38]). Effective restoration programs require a thorough understanding of forest structure. Old-growth attributes (i.e., functional and structural features associated with old-growth forests) develop naturally over time, but appropriate forest management can also facilitate their development ([5]). Active restoration entails the implementation of various management techniques, such as planting seedlings, girdling, or thinning, and passive restoration describes a situation in which no action is taken except to reduce environmental stressors, such as dead wood removals or harvesting. There is vigorous debate as to which management strategy is most suitable to implement the development of old-growth attributes. Recent studies suggest that active restoration of old-growth attributes provides alternatives to conventional even- and uneven-aged silvicultural practices ([28], [15]); however, such approaches (e.g., generating snags by girdling diseased or dying trees, cutting to a rotated sigmoid diameter distribution, and downing logs by pulling or pushing trees over) are expensive. Alternatively, passive restoration should be considered when the restoration process can be expected to achieve the desired forest structure within a feasible time period ([35]).
In Central Europe, mountain areas constitute some of the largest remnants of primary forests that have survived well into modern times ([24], [64]). Because of their inaccessibility, most were not managed until the nineteenth century. Improvements in transportation and increasing wood fuel demands for industry increased the accessibility and value of untouched forests. However, even in remote mountainous areas, the possibility of centuries of selective logging, grazing, or charcoal burning can be recognized ([50]). Large aristocratic and monastic hunting estates of the past have contributed to the protection of many untouched forests. Nowadays, forest reserves under varying protection regimes account for only 12% of the European forest area ([48]). Although the protected area network has increased substantially in the last few decades, many allow large-scale harvesting inside their boundaries and numerous areas have been illegally harvested ([31], [67]). Thus, the concepts and strategies that integrate forest habitat requirements into the management and production of other forest goods and services are mandatory for sustainable forest management and the preservation of forest biodiversity in landscape ecosystems ([5], [49]).
In the Czech Republic, conservation programs protecting forest reserves have increasingly used passive restoration methods since 2002. More than 2.000 ha of forest areas have been entirely excluded from any forest management, but that corresponds to only 0.83 % of all forests in the Czech Republic ([66]). These protected forest areas are still very small and generally consist of smaller, isolated patches, as is common in most European countries ([47]). One of those unmanaged forests is located in the Jizera Mountains Beech Forest Reserve, the largest continuous European beech (Fagus sylvatica L.) forest complex in the Czech Highlands. This area provides a unique perspective on forest changes after the implementation of passive management. However, it is uncertain how quickly forest structural complexity will change and how the transition to old-growth status will develop afterwards. As a result of climate change, accelerating rates of change in structure, composition, and mortality have been reported worldwide ([3]). Quantifying the patterns and processes in developing forests is essential for conserving biodiversity and ecosystem services. We employed repeated census plot data to quantify changes in forest structure and mortality after the cessation of active forest management, and we addressed the following questions: (i) what is the current stand structure of forests in the Jizera Mountains Beech Forest Reserve after the cessation of management? Does it already have some old-growth attributes? How do selected structural characteristics compare to those of primary old-growth forests? (ii) How much have the selected structural characteristics changed over a 12-year study period? What are the rates of mortality and new tree recruitment? What are the size-mortality trends within the nature reserve? How has diameter distribution changed during the studied period?
Methods
Study area
The study area is located in the Jizera Mountains (Jizerské hory) within the Jizera Mountains Beech Forest Reserve (Jizerskohorské bučiny), Czech Republic (Fig. 1). The reserve is situated on the northern slopes along an altitudinal gradient ranging from 360 to 1006 m a.s.l. The climate is very humid because the Jizera Mountains are the first transcontinental barrier of the humid oceanic flow from the North Sea. Annual rainfall averages range between 800 and 1700 mm and the growing season lasts from 110 to 160 days ([60]). The average annual temperatures, which are strongly related to altitude, range from 3 to 7.5 °C. The uniform bedrock consists of a significant proportion of porphyric biotite granite to granodiorite, and the northwestern portion is composed of coarse-grained porphyric biotite granite. Cambisols and podzols, the most common soils in the reserve, are strongly acidic and have high contents of poor quality humus with highly variable depth profiles ([69]).
Fig. 1 - Location of the research area in Czech Republic (Jizera Mountains Beech Forest). Reserve and core zones in the reserve. Empty squares represent the study plots (A-H).
Land use history
We have records of at least 200 years of selective logging in the reserve prior to 1960, after which the most preserved sites of beech-dominated forests representative of the old-growth attributes were protected in small, dispersed reserves. In 1999, these isolated reserves became the core zones of a single protected area 27 km2 in size connected with buffer zones. Since 1960, soft active management, including selective logging, dead wood removal, banding trees, and also plantations in some portions, was conducted throughout the buffer zones; however, the 9.5 km2 of dispersed core zones were allowed to develop naturally without any management and spontaneous forest development persisted.
Natural vegetation includes mainly mountain acidic beech-dominated forests and small areas of high-elevation spruce forests. The main tree species in the reserve are F. sylvatica (79%) and Picea abies (L.) Karst. (13%). Acer pseudoplatanus L. (2.8%), Sorbus aucuparia L. (2.2%), and Betula pendula Roth (1.2%) are admixed throughout, and a few species, such as Larix decidua Mill. (0.64%), Fraxinus excelsior L. (0.19%), Abies alba Mill. (0.08%), and Acer platanoides L. (0.01%), occur only rarely ([69]).
Data collection
In 2003, eight 50 × 50 m permanent research plots were established at the forest reserve within the core zones (Fig. 1), a minimum distance of 50 m was maintained between all plots and any core zone edges. Plots were selected based on site conditions observed in the field in a part of the reserve comprised entirely of the most well-preserved sites that were allowed to develop naturally without any extensive forest management. The plot locations were selected to reflect more complex cover textures and forest structural variability ([61]). A description of the study plots is reported in Tab. 1. Species, diameter at breast height (DBH), status based on Kraft classification ([4]), and height were recorded for all living trees ≥ 5 cm DBH. All trees, dead wood, and rocks were mapped using a compass and diameter tape. When plots were re-measured in 2015, DBH, height, and status were recorded for all trees ≥ 5 cm DBH and ≥ 20 cm tall, as well as for standing dead trees, including snags and stumps. Dead trees were defined as being physiologically dead if the crown had been completely destroyed or if the tree was completely uprooted and dead. Lying dead wood tallied included portions of trunks and large branches with a minimum length of 1 m and a minimum diameter of 6 cm. Spatial location of all trees and dead wood were recorded with Field-Map electronic and laser measuring devices linked to GIS ([27]). Topographic attributes, including slope, aspect, and altitude, were recorded for all plots (Tab. 1). For a random subsample of trees, a single increment core at breast height (1.3 m) was extracted from living trees ≥ 5 cm DBH (n = 85) of different tree species (F. sylvatica, n = 77; Acer spp., n = 5; P. abies, n= 2; S. aucuparia, n = 1). The number of cores on each plot varied depending on the live tree density.
Tab. 1 - Characteristics of the study plots (A-H).
Plot | Area (ha) |
Altitude (m a.s.l.) |
Slope (°) |
Aspect |
---|---|---|---|---|
A | 0.30 | 590 | 35 | E |
B | 0.25 | 680 | 30 | W |
C | 0.25 | 610 | 35 | N |
D | 0.25 | 690 | 24 | NW |
E | 0.25 | 700 | 25 | NW |
F | 0.25 | 580 | 22 | NW |
G | 0.25 | 680 | 30 | S |
H | 0.25 | 840 | 40 | S |
Data processing
Increment cores were dried, cut with a core microtome, and secured on wooden mounts. The widths of the growth rings were measured according to standard dendrochronological analysis ([59]) using TSAP-Win software ([53]) and verified with CDendro software ([12]) software. We used increment cores that included or were close to the pith: 33% of all cores included the pith, 25% were within 0.5 cm of the pith, 29% were within 2 cm of the pith, and 13% were within 10 cm of the pith. The age at breast height of trees with complete cores was estimated based on the crossdated tree ring series. To determine the age of trees without the pith, a standard graphical method was applied ([17]). Only one sample did not cross-date well, and it was excluded from all further analyses.
Data analyses
We calculated density, basal area, mean diameter, standard deviation of diameter, species composition by basal area, and density of large trees with DBH > 50 cm and > 80 cm. Volume of living trees and standing dead trees was calculated using two-parameter (DBH and height) equations derived by Petráš & Pajtík ([51]). Lying dead wood volume was evaluated using Field-Map software ([27]). The decay stage was assessed according to Sippola & Renvall ([57]), whereby decay was primarily based on the depth of penetration of an iron spike. As indicators of spatial indices of structural diversity, we calculated the coefficient of variation and Gini coefficient based on basal area to characterize inequity in the size distribution among living trees ([20]). Annual mortality, m, was calculated based on the 12-year study period ([56] - eqn. 1):
where N0 and Nt are the numbers of living trees at the beginning and at the end of the interval, respectively, and t is the inventory interval in years. The mortality within size classes was evaluated for five classes (5-9.9 cm, 10-29.9 cm, 30-49.9 cm, 50-69.9 cm, and ≥ 70 cm DBH). To account for tree recruitment, new trees 5 to 20 cm DBH were measured during the 2015 inventory. Mean age of the oldest tree of each plot was estimated as an average of the five oldest trees on the plot. Tree recruitment patterns were visually explored using age distribution at the landscape level. The third-degree polynomial function was used to describe the age-diameter relationship of F. sylvatica (n = 76); relationships for other species were only visually explored. The diameter distribution of living trees was analyzed by fitting a nonparametric kernel estimation and third-degree polynomial function; temporal changes in the diameter distributions of living trees between inventories were also tested using a Kolmogorov-Smirnov test. Finally, our results were compared with other studies from old-growth European beech-dominated forest reserves. Relevant publications were identified by searching the online database of Web of Science using the following search terms: Fagus, structure or structural, old-growth or virgin or primary. After applying the search filters, a total of nine articles were identified that reported structural biometrics directly relevant to our analyses and were located within Europe. For further comparison, the mean values of our selected variables were computed from a review of European beech-dominated forests by Burrascano et al. ([6]). All calculations were performed using R statistical software ([54]).
Results
Current forest structure
An overview of structural characteristics is presented in Tab. 2. Across all study plots, F. sylvatica was the dominant tree species; it accounted for at least 85% of the basal area on all plots, and some plots contained only F. sylvatica. Among standing dead trees, F. sylvatica was also the dominant species (53% to 100% of the basal area), with the exception of one plot, where P. abies represented 53% of the basal area (Tab. 2). Tree density and basal area varied greatly among plots. The mean tree density was 204 trees ha-1 and 20 trees ha-1 for living and standing dead trees, respectively. On average, basal area was 35.5 m2 ha-1 and 4.9 m2 ha-1 for living and standing dead trees, respectively. Plots all had a relatively high mean DBH of living trees, with the exception of plot C, but the mean DBH of standing dead trees was slightly greater (Tab. 2). Frequency of large trees with DBH ≥ 50 cm was relatively high in all plots (Tab. 2). Density of larger living trees with DBH ≥ 80 cm varied within study plots, with an average of 17 trees ha-1. For standing dead trees, the density varied to a lesser extent, with an average of 4 trees ha-1. The Gini coefficient and coefficient of variation varied within study plots, but the highest values were calculated for plot C (Fig. 2). The mean volume was 558 m3 ha-1 for living trees and 69 m3 ha-1 for dead wood, both standing and lying dead wood. Dead wood was present in all plots, with 3 to 34% of the total volume as dead wood. Lying dead wood contributed an average of 64% of this volume, however their proportion varied among study plots and ranged from 29 to 90% (Tab. 3). All decay classes were present, however, lying dead wood in decay classes 3 and 4 represented almost 75% of the total volume of lying dead wood. The most common species among lying dead wood was F. sylvatica; the contribution of other tree species was negligible (Fig. 3).
Tab. 2 - Structural characteristics of the study plots (A-H). (1): Average value for all study plots; (2): trees with DBH ≥50 cm; (3): trees with DBH ≥80 cm; (4): mean age of five oldest trees in the plot; (5): age of the oldest tree in then plot (all oldest trees were F. sylvatica).
Variable | Status/ Stats |
Year | Plots | Avg (1) | |||||||
---|---|---|---|---|---|---|---|---|---|---|---|
A | B | C | D | E | F | G | H | ||||
Percentage of Fagus sylvatica (%) |
Living | 2015 | 100 | 92 | 85 | 100 | 97 | 100 | 90 | 91 | 94 |
Dead | 62 | 86 | 77 | 100 | 53 | 100 | 47 | 71 | 75 | ||
Mean Diameter (cm) |
Living | 2003 | 48.8 | 61 | 21.6 | 48.1 | 55.7 | 50 | 49.8 | 39.7 | 46.8 |
2015 | 50.4 | 49.7 | 20.5 | 44.5 | 43.3 | 50.9 | 49.8 | 39.7 | 43.6 | ||
Dead | 2015 | 29.3 | 61 | 46.4 | 58.6 | 43.3 | 38.6 | 40.5 | 51.5 | 46.2 | |
STD Diameter (cm) |
Living | 2003 | 14.5 | 17.1 | 21.2 | 27.7 | 17.5 | 25.5 | 28.4 | 18 | 21.2 |
2015 | 15.7 | 28.9 | 19.2 | 31.7 | 28.7 | 27.6 | 27.1 | 18.3 | 24.7 | ||
Dead | 2015 | 14.3 | 17.9 | 28.7 | 24 | 15.7 | 5.4 | 22.1 | 25.9 | 19.3 | |
Basal area (m2 ha-1) |
Living | 2003 | 29.8 | 33.9 | 31.3 | 36.5 | 29.9 | 40.4 | 40.1 | 29.8 | 34 |
2015 | 32 | 35 | 29.9 | 39.9 | 32.8 | 42.9 | 38.2 | 32.9 | 35.5 | ||
Dead | 2015 | 1.8 | 5.9 | 9.3 | 6.5 | 1.1 | 0.8 | 6.5 | 7.1 | 4.9 | |
Stem density (inds ha-1) |
Living | 2003 | 147 | 108 | 436 | 152 | 112 | 164 | 156 | 200 | 184 |
2015 | 147 | 136 | 484 | 172 | 156 | 164 | 152 | 220 | 204 | ||
Dead | 2015 | 20 | 16 | 28 | 20 | 4 | 8 | 36 | 28 | 20 | |
Large trees 50 (2) (inds ha-1) |
Living | 2003 | 83 | 80 | 52 | 72 | 80 | 80 | 68 | 48 | 70 |
2015 | 80 | 76 | 48 | 80 | 80 | 84 | 68 | 56 | 72 | ||
Dead | 2015 | 7 | 16 | 24 | 24 | 8 | 0 | 16 | 16 | 14 | |
Large trees 80 (3) (inds ha-1) |
Living | 2003 | 0 | 20 | 12 | 20 | 4 | 20 | 20 | 4 | 13 |
2015 | 0 | 20 | 16 | 32 | 4 | 32 | 20 | 8 | 17 | ||
Dead | 2015 | 0 | 4 | 8 | 8 | 0 | 0 | 4 | 4 | 4 | |
Age (years) |
Mean (4) | 2015 | 205 | 198 | 167 | 212 | 205 | 257 | 153 | 171 | 196 |
Max (5) | 252 | 237 | 236 | 220 | 242 | 289 | 160 | 173 | 234 |
Fig. 2 - Structural diversity indices. Gini coefficient (left panel) and coefficient of variation of tree diameters (right panel) indices characterizing the study plots (A-H) in 2003 (green) and 2015 (yellow). Dark green indicates a decrease of the index value during the study period.
Tab. 3 - Summary of dead wood volume on study plots (A-H) in 2015.
Variable | Status | A | B | C | D | E | F | G | H | Avg |
---|---|---|---|---|---|---|---|---|---|---|
Volume (m3 ha-1) |
Living | 494 | 520 | 433 | 716 | 534 | 726 | 649 | 391 | 558 |
Standing dead | 7 | 16 | 55 | 4 | 5 | 7 | 83 | 82 | 32 | |
Lying dead wood | 46 | 36 | 46 | 38 | 23 | 16 | 34 | 51 | 36 | |
Total dead wood | 53 | 52 | 101 | 42 | 28 | 23 | 117 | 133 | 69 | |
Dead wood/ living ratio (%) | 11 | 10 | 23 | 6 | 5 | 3 | 18 | 34 | 14 | |
St. dead/ dead wood ratio (%) | 13 | 31 | 54 | 10 | 18 | 30 | 71 | 62 | 36 |
Fig. 3 - Volume of lying dead wood (m3 ha-1) by decay classes, including recent dead (1) to the highly decayed wood (5), and species on study plots (A-H) in 2015. (FASY): Fagus sylvatica; (PIAB): Picea abies; (ABAL): Abies alba; (ACPS): Acer pseudoplatanus; (SOAU): Sorbus aucuparia.
The oldest tree was a 289-year old F. sylvatica (on plot F). The maximum age of A. pseudoplatanus and P. abies was estimated to be 165 and 153 years old, respectively. The average of the mean plot ages was 196 years, with a range of 153 to 257 years (Tab. 2). Tree establishment occurred irregularly throughout the last three centuries. There were four peaks observed around 1770, 1800-1810, 1850-1870, and 1960 (see Fig. S1 in Supplementary material), and relatively long periods of four decades or more with little tree establishment. The evaluation of the relationship between tree age and diameter among F. sylvatica was best described by a third polynomial regression (n = 77, R2 = 0.698 - Fig. 4).
Fig. 4 - Relationship between tree age and tree diameter from all study sites. Loess curve with the 95% confidence interval is reported for the relationship of Fagus sylvatica species. (FASY): Fagus sylvatica; (PIAB): Picea abies; (ACPS): Acer pseudoplatanus; (ACPL): Acer platanoides; (SOAU): Sorbus aucuparia.
Changes in forest structural characteristics
The most variable stand characteristic over time was the density of large trees ≥ 80 cm DBH with a mean increase of 32.0% (Tab. 2). Other structural attributes were much less variable over time; value increases were +10.6% for stem density, +4.4% for basal area, and +1.6% for density of large trees > 50 cm DBH. Standard deviation of diameter displayed a relatively sustained increase of 16.1%. No major changes in species composition were observed between the two measurements. Changes in the structural diversity indices varied greatly among study plots, whereas the overall mean increase was 13.1% for the coefficient of variation and 19.4% for the Gini coefficient (Fig. 2). The highest change of structural indices was observed on plot E. The size-mortality trend was asymmetrically U-shaped for all study sites. Annual mortality rates declined from about 0.3% in the smallest diameter class to 0.1% in the 20-cm class, and then it increased to about 0.8% in classes ≥ 70 cm DBH (Fig. 5). During the 12-year study period, the overall mortality rate was 0.43% year-1 (19 dead of 376 living trees in 2003 - see Tab. S1 in Supplementary material). Three trees were uprooted, and two trees had a broken stem, but they were still alive. The highest mortality rate was observed on plot B (0.64% year-1), and the lowest was on plot A (0.19% year-1). Mortality rates were balanced by the turnover of new tree generations. The generally high recruitment rates during the study period varied among plots; the highest observed densities were on plots C and E. The most abundant species in the recruitment layer (5 to 20 cm DBH) was F. sylvatica; P. abies, A. platanoides, and S. aucuparia were also present in low densities.
Fig. 5 - Observed annual mortality rate by size class (5-9.9 cm, 10-29.9 cm, 30-49.9 cm, 50-69.9 cm, and ≥ 70 cm) at the study plots (A-H). Numbers in white above each bar indicate the sample size (number of living trees in 2003).
Live tree diameter distribution of the study forest was dominated by F. sylvatica across all diameter classes. Most plots consisted of F. sylvatica in the upper canopy with P. abies in the mid-canopy, and other species were most common in the lower canopy. The change in the forest level diameter distribution between 2003 and 2015 reflected a convergence to a rotated sigmoid shape (Fig. 6). The plot level diameter distribution displayed a variety of structures in 2003, often with a tendency towards unimodal distributions, although the reverse J-shaped form was the best fit for study plot C. Over time, the rotated sigmoid curves were evident in most plots (B, C, D, E, F) in 2015. The other plots (A, G, H) remained stable over time and they had unimodal shapes (see Fig. S2 in Supplementary material). Moreover, the changes were not significant for any of the plots over time (Tab. S2). Size structure was adequately predicted by the third-degree polynomial function, and it proved to be a better fit than the Kernel function.
Fig. 6 - Forest level diameter distribution. Cumulative empirical diameter distributions in 2003 (left) and 2015 (right). The solid lines represent the third-degree polynomial function (red) and the Kernel function (blue) for all living trees. Individual trees are grouped into 5 cm diameter classes. (FASY): Fagus sylvatica; (PIAB): Picea abies; (ACPS): Acer pseudoplatanus; (ACPL): Acer platanoides; (SOAU): Sorbus aucuparia.
Discussion
Current forest structure
The core zones of the Jizera Mountains Beech Forest Reserve are composed of relatively structurally heterogenous forests. They contain many large and old trees, multilayered canopies, and high variation in tree size and age, all structural characteristics typically associated with old-growth forests ([5]). The structural attributes were comparable with other abandoned European beech-dominated forest reserves or primary old-growth forests in Europe (Tab. 4). With the exception of lower dead wood volumes, which are undergoing an accumulation process, the other structural parameters were within the ranges reported by other studies.
Tab. 4 - Structural data for old-growth European beech-dominated forests. (1): This study (Jizera Mountains Beech Forest); (2): [32] (Zofín); (3): [24] (Hrončecký grún); (4): [34] (Stužica); (5): [46] (Labowiec); (6): [10] (Uholka); (7): [29] (Janj); (8): [42] (Lom); (9): [10] (Sihlwald); (10): [43] (Ludrin); (11): [6] (mean for old-growth European beech-dominated forests); (12): for the study period 2001-2010; (13): standing and lying dead wood. Bedrock: (gr) granite; (an) andesite; (fl) flysch; (li) limestone. Soils: (ca) cambisols; (po) podzols; (re) rendzina. (∞): Considered as a primary forest; (Qmd): Quadratic mean diameter.
Variable | Status | Country | ||||||||||
---|---|---|---|---|---|---|---|---|---|---|---|---|
CZ1 | CZ2 | SK3 | SK4 | PL5 | UA6 | BA7 | BA8 | CH9 | IT10 | EU11 | ||
Last management | - | 1960 | 1882 | ∞ | ∞ | 1957 | ∞ | ∞ | 1956 | 1995 | 1962 | - |
Altitude (m a.s.l.) |
- | 671 | 780 | 890 | 1075 | 900 | 750 | 1330 | 1386 | 650 | 1300 | - |
Bedrock | - | gr | gr | an | fl | fl | fl, li | li | li | - | li | - |
Soils | - | ca, po | ca, po | ca | ca | ca | - | ca, re | re | ca | ca | - |
Age (years) | - | 196 | 169 | - | - | - | 150 | - | - | 150 | - | - |
Basal area (m2 ha-1) |
Living | 35.5 | 40.9 | 41.8 | 38.2 12 | 36.3 | 38.5 | 66.7 | 47.1 | 30.7 | 45.1 | 39.9 |
Qmd (cm) | Living | 47.1 | 50.3 | 46.8 | 38.1 | - | 47.3 | 40.6 | 35.0 | 38.9 | 27.8 | 64.9 |
Density (trees ha-1) |
Living | 204 | 206 | 243 | 336 | - | 219 | 516 | 489 | 259 | 741 | 447 |
Living 50 | 72 | - | - | - | 73 | - | 115 | 99 | - | 52 | 94 | |
Living 80 | 17 | - | 6 | 12 | - | 21 | 42 | 18 | - | 0 | - | |
Volume (m3 ha-1) |
Living | 558 | 695 | 724 | 560 | 543 | 770 | 1215 | 763 | 524 | 533 | - |
Standing dead | 32 | - | 25 | - | - | - | 80 | 91 | - | 8 | - | |
Lying dead | 36 | 202 13 | 281 | 133 | 383 13 | 111 | 307 | 236 | 8 13 | 70 | 193 13 | |
Dead/ living ratio (%) | 14.0 | 22.3 | - | 23.7 | - | 13.0 | - | 42.9 | 1.4 | - | - |
The importance of disturbances in dead wood dynamics is well documented ([9], [41], [39]). Each disturbance type has characteristic frequency and intensity patterns, which produces important differences in the spatial and temporal distribution of dead wood. Natural disturbance regimes in European temperate deciduous forests are characterized by mixed disturbance severity regimes, a combination of frequent, small-scale events, and occasional moderate to high-severity events, predominantly caused by storms and drought ([16], [62], [44]). Mixed disturbance severity regimes tend to generate irregular inputs of dead wood, with a wide range of sizes, shapes, and decay classes present ([9]). Christensen et al. ([9]) reported an average of 132 m3 ha-1 of dead wood for primary and long-established European beech-dominated forest reserves. Burrascano et al. ([6]) observed higher dead wood quantities (mean = 193 m3 ha-1) in a European dataset of old-growth forests. Other studies of European beech-dominated forests indicate a natural range of 78 to 387 m3 ha-1 (Tab. 4). In Poland, Paluch ([46]) observed more than 380 m3 ha-1 of dead wood in the Labowiec Forest Reserve, which was similar to findings from the Janj Forest Reserve in Bosnia and Herzegovina ([29]). In our study, forests with no management for more than 50 years had from 23 to 133 m3 ha-1 of dead wood, which is much lower than reported in other studies. Based on the dead to live wood ratio, the observed average value of 14% was lower than the mean ratio of 21% for recently-established mountain forest reserves ([9]). According to a review by Burrascano et al. ([6]), our plots fall in the lower part of the range (6-89%). This pattern is likely related to the more recent soft silvicultural practices (targeted tree gridling, dead wood removal, or understory thinning). On average, standing dead trees represented 36% of the dead wood recorded, but the proportion varied among plots (from 10 to 71%). This was consistent with the percentage of standing dead wood in other old-growth beech-dominated forests, which ranged from 16 to 47% ([9], [63]). The high volume of standing dead trees on plot G was likely due to the higher abundance of coniferous trees, which are generally larger and their decay slower than most deciduous trees ([50]). All dead wood decay classes were found among the study sites, but the most advanced class (5) was relatively infrequent. This is likely associated with the past silvicultural practices, which likely reduced overall mortality and dead wood inputs ([37]). Some studies have suggested that in the absence of major disturbances, dead wood accumulation in managed forests with no management is a slow process ([63], [41]). Peterken ([50]) suggested that accumulation of near natural volumes of dead wood could occur within a century. Comparing our results to dead wood stocks from primary old-growth forests or reserves abandoned for more than 100 years suggests that our studied forest reserve is at the beginning of a long process of dead wood accumulation ([33], [9], [41]). The retention of dead wood as well as artificial creation of dead wood have been identified as the key approaches to successful dead wood management in forests where the aim is to maintain biodiversity ([65]).
Living tree density and basal area were also comparable with other studies of old-growth European beech-dominated forests. According to a review by Burrascano et al. ([6]), the mean values of basal area and stem density were higher than the values reported in our study. Our plots had a higher quadratic mean diameter (47.1 cm) compared to other studies from Europe ([10], [24], [34], [42], [29], [43]); however, Burrascano et al. ([6]) reported slightly higher values (mean of 64.9 cm) within old-growth European beech-dominated forests. The presence of large trees is considered one of the most important features of old-growth forests. Large F. sylvatica trees play a unique and critical role in supporting diversity of other organisms, such as birds ([13]) and lichens ([23]). Burrascano et al. ([6]) reported a minimum threshold of large tree density for old-growth forests at a global scale (30 trees with DBH ≥ 50 cm), which was observed in our study, but the mean value for European beech-dominated forests is considerably higher. Frequency of larger trees with DBH ≥ 80 cm was similar to the values found in other old-growth European beech-dominated forests.
Tree age analysis revealed that the age distribution was multimodal (Fig. S1 in Supplementary material), a pattern commonly found in old-growth beech-dominated forests in Central Europe ([33], [62]). Four recruitment peaks were evident around 1770, 1800-1810, 1850-70, and 1960. The number of old trees in our sample was relatively low, so some of the oldest recruitment periods, such as 1770 to 1810, were difficult to interpret. The longer frequent recruitment period from 1850 to 1870 has also been observed in other Bohemian (Zofín) and Carpathian (Uholka) beech-dominated forest reserves; a higher frequency of strong windstorms has been identified during this period for the region ([62], [55]). The obvious rise in tree recruitment after 1960 can be linked to the mortality of older trees; as older cohorts begin dying, recruitment of a new generation of trees begins. Cautiously, we cannot exclude limited historical human impact in this area that may have influenced recruitment rates. The age distribution also helped explain the sigmoid form of the diameter distribution (Fig. 6); it displayed a more or less pronounced peak in the midsize diameters ([62]). At least one F. sylvatica more than 250 years old was found, but that is younger than the oldest tree (409 years old) previously reported for the Czech Republic ([7]). According to Di Filippo et al. ([14]), the maximum and mean age of the three to five oldest trees are good indicators to evaluate old-growth status. Thus, the observed plot mean age of the oldest trees (196 years) demonstrated a characteristic feature of studied old-growth forests, a period when the mean canopy age is at least half the maximum lifespan of the dominant tree species ([62]). The old-growth status of a stand can also be characterized by high variability in tree ages within a given diameter class (Fig. 4), which reflects the complex dynamics of uneven-aged forests ([52], [62], [14]).
Changes in forest structural characteristics
Our study highlighted the changes of old-growth features over time since the last forest management interventions; most notably, the density of very large trees, structural diversity, and diameter structure. The U-shaped mortality pattern is characteristic of old-growth forests. Indeed, the relatively high numbers of recruited trees demonstrate the dynamics of the natural regeneration process.
The greater change over time was observed in the density of large living trees with DBH ≥ 80 cm; changes in the density of smaller trees (DBH ≥ 50 cm) did not increase as much. Variability in living stem density between measurements was twice as high as that of basal area, as indicated by the relatively high change in stem numbers observed in the lower tree layers (5 to 20 cm DBH). The increased basal area could also be interpreted as another indicator of the changes towards more natural forest structures, as harvesting activities in managed forests typically prevent stand basal area from reaching such high values ([22]). Also, average temporal changes of structural indices showed slight increases (Fig. 2). The DBH differentiation, expressed as the Gini coefficient, was similar to values observed in unmanaged forests of the Carpathians ([45]). Our results reflect the general pattern that even-aged forests are more homogenous than uneven-aged forests, as suggested by Sterba & Zingg ([58]). The highest Gini indices were found within mixed uneven-aged plots, and pure even-aged plots, which were relatively homogenous, had the lowest Gini indices. Large tree size diversity may ensure a wide range of habitats and provide higher levels of biodiversity in forest ecosystems ([45], [30]).
The diameter structure of the study forest changed over time to a typical rotated sigmoid pattern. Changes in tree numbers were most evident in the smallest diameter classes compared to 2003. The higher number of trees frequently found in the midsize diameters range elucidates a common trend towards a rotated sigmoid diameter distribution. The diameter structures of individual plots indicated variability at the beginning of the study period. Most plots converged towards a rotated sigmoid diameter distribution over time, even the plot with reverse J-shaped diameter structure. In a few plots the diameter structure did not change substantially and they reflected a unimodal structure at the end of the study period. The rotated sigmoid curve of diameter distribution is commonly observed in old-growth forests dominated by F. sylvatica ([68], [2], [62]). Various studies from North America have also reported diameter distributions of a rotated sigmoid form for various mixed-species forests ([21], [38]). Westphal et al. ([68]) indicated three main mechanisms, including infrequent disturbance events, mortality, and nonlinear diameter growth rates, which likely explain the rotated sigmoid structure. A mixed disturbance severity regime and associated regeneration can create discontinuous and unbalanced diameter structures. Non-constant mortality rates with a lower mortality rate for all trees that successfully reached the upper canopy level and increased mortality rates for very large trees results in mid-size peaks in diameter distributions. The third factor that drives the development of a rotated sigmoid curve is a positive relationship between diameter increment and stem size ([68]). The empirical diameter distributions were most precisely predicted by a third-degree polynomial function, which is commonly used to model diameter distributions ([68]).
The U-shaped mortality trend with minimum mortality rates in the midsize classes corresponded to the current diameter structure that appeared to be converging towards a rotated sigmoid curve (Fig. 6). Complete U-shaped mortality has been reported in several old-growth beech-dominated forests throughout Europe ([2], [26]). Larger trees are more susceptible than medium-sized trees to insect attacks, drought, rot, and mechanical instability during storms ([25], [26]). The mortality of small-sized trees is mostly attributed to competition, whereby smaller trees have to compete against larger trees for light, water, and nutrients ([1], [11]). Annual mortality rates for all species were 0.43% on average, but that is lower than rates (more than 1%) reported for other old-growth beech-dominated forests in Central Europe ([25], [26]). Tree mortality rates are typically low in the absence of larger disturbances ([50]). Together with observed increases in the density of large trees and basal area in our study forest, the increase in mortality is predictable given the forest age. However, the mortality rates are balanced in the turnover of new tree generations, and the stand gradually again advances to the high growth rate stage of development. Species-specific mortality has been suggested to account for contrasting life history strategies, lifespan, competitiveness, and varying responses to abiotic factors ([18], [25]).
Conclusions
Our study of the Jizera Mountains Beech Forest Reserve core area over a 12-year period found persistent transition of the forest towards natural states after more than five decades without management. The study area displayed a variety of old-growth characteristics (e.g., density of large, old trees, structural heterogeneity, diameter distribution, and mortality), which is consistent with previously reported studies on primary old-growth forests or reserves that had never been managed or unmanaged for even longer time periods. The impacts of past management are still visible in the low amounts of dead wood, but the reserve is at the beginning of a long process of dead wood accumulation. As forest development progresses, the death of old trees allows for higher recruitment into the understory and it enhances the growth rates of the surviving trees; this pulse in recruitment cycles and dead wood accumulation will continue in the absence of disturbance or management. Although our study is based on a repeated census dataset covering 12 years, this time span is still very short relative to both the longevity of trees and forest succession. The monitoring of secondary old-growth forest reserves and maintaining forest ecosystem services will help ensure responsible stewardship for integrative forest management that aims to address ecological, economic, and social issues across entire forests over time.
Acknowledgements
This study was supported by IGA project (B0917) financed by the Internal Grant Agency of FFWS CULS in Prague, institutional project “EVA4.0” (CZ.02.1.01/0.0/0.0/16_019/0000803) and project INTER-COST (LTC17055) financed by Ministry of Education, Youth and Sports of Czech Republic. We especially thank to J. Trešnák for providing all data from his diploma thesis. We would like to thank P. Uzel, M. Trčka, J. Hanousek, M. Matouš, D. Hetešová, F. Chrapan, and S. Komín for assistance in the field. We would also like to thank the Jizera Mountains Protection Landscape Area authorities, especially V. Vršovský, for administrative support. Also, thanks to the anonymous reviewers for their helpful and constructive reviews.
JL designed the methods, carried out surveys, measurement and analysis, coordinated the research team, developed and wrote the manuscript, RCM performed the language revision and helped to draft the manuscript, VT helped with data analysis, PJ, RB, VC, MM, HM and JSS helped with writing the manuscript, KS, LM, MS helped with the laboratory measurement, MS conceived the study and helped to draft the manuscript.
References
Gscholar
Gscholar
Gscholar
Gscholar
Gscholar
Gscholar
Gscholar
CrossRef | Gscholar
Gscholar
Gscholar
CrossRef | Gscholar
CrossRef | Gscholar
Gscholar
CrossRef | Gscholar
Online | Gscholar
Gscholar
Gscholar
Gscholar
Gscholar
Gscholar
Gscholar
Gscholar
Gscholar
Gscholar
Authors’ Info
Authors’ Affiliation
C Morrissey Robert
Volodymyr Trotsiuk
Pavel Janda
Radek Bače
Vojtech Cada
Martin Mikoláš
Hana Mrhalová
S Schurman Jonathan
Kristýna Svobodová
Lenka Mateju
Michal Synek
Miroslav Svoboda
Czech University of Life Sciences Prague, Faculty of Forestry and Wood Sciences, Kamyeckae 129, Praha 6, Suchdol 16521 (Czech Republic)
Swiss Federal Institute for Forest, Snow and Landscape Research WSL, Zuercherstrasse 111, 8903 Birmensdorf (Switzerland)
ETH Zurich, Department of Environmental Systems Science, Institute of Agricultural Sciences, 8092 Zurich (Switzerland)
PRALES, o.z., Odtrnovie 563, Rosina, 03122 (Slovakia)
Faculty of Environmental Sciences, Czech University of Life Sciences Prague, Kamýcká 129, Prague 6, 165 21 (Czech Republic)
Corresponding author
Paper Info
Citation
Lábusová J, Morrissey Robert C, Trotsiuk V, Janda P, Bače R, Cada V, Mikoláš M, Mrhalová H, Schurman Jonathan S, Svobodová K, Mateju L, Synek M, Svoboda M (2019). Patterns of forest dynamics in a secondary old-growth beech-dominated forest in the Jizera Mountains Beech Forest Reserve, Czech Republic. iForest 12: 17-26. - doi: 10.3832/ifor2702-011
Academic Editor
Gianluca Piovesan
Paper history
Received: Nov 30, 2017
Accepted: Oct 26, 2018
First online: Jan 10, 2019
Publication Date: Feb 28, 2019
Publication Time: 2.53 months
Copyright Information
© SISEF - The Italian Society of Silviculture and Forest Ecology 2019
Open Access
This article is distributed under the terms of the Creative Commons Attribution-Non Commercial 4.0 International (https://creativecommons.org/licenses/by-nc/4.0/), which permits unrestricted use, distribution, and reproduction in any medium, provided you give appropriate credit to the original author(s) and the source, provide a link to the Creative Commons license, and indicate if changes were made.
Web Metrics
Breakdown by View Type
Article Usage
Total Article Views: 48871
(from publication date up to now)
Breakdown by View Type
HTML Page Views: 40487
Abstract Page Views: 4560
PDF Downloads: 3119
Citation/Reference Downloads: 2
XML Downloads: 703
Web Metrics
Days since publication: 2364
Overall contacts: 48871
Avg. contacts per week: 144.71
Article Citations
Article citations are based on data periodically collected from the Clarivate Web of Science web site
(last update: Mar 2025)
Total number of cites (since 2019): 6
Average cites per year: 0.86
Publication Metrics
by Dimensions ©
Articles citing this article
List of the papers citing this article based on CrossRef Cited-by.
Related Contents
iForest Similar Articles
Research Articles
A geographically weighted deep neural network model for research on the spatial distribution of the down dead wood volume in Liangshui National Nature Reserve (China)
vol. 14, pp. 353-361 (online: 27 July 2021)
Research Articles
Comparison of traits of non-colonized and colonized decaying logs by vascular plant species
vol. 11, pp. 11-16 (online: 09 January 2018)
Research Articles
Evaluation of estimation methods for fitting the three-parameter Weibull distribution to European beech forests
vol. 15, pp. 484-490 (online: 01 December 2022)
Research Articles
Improving sustainability in wood coating: testing lignin and cellulose nanocrystals as additives to commercial acrylic wood coatings for bio-building
vol. 14, pp. 499-507 (online: 11 November 2021)
Research Articles
Patterns of genetic diversity in European beech (Fagus sylvatica L.) at the eastern margins of its distribution range
vol. 10, pp. 916-922 (online: 10 December 2017)
Research Articles
Suitability of Fagus orientalis Lipsky at marginal Fagus sylvatica L. forest sites in Southern Germany
vol. 15, pp. 417-423 (online: 19 October 2022)
Research Articles
Dead wood and stand structure - relationships for forest plots across Europe
vol. 7, pp. 269-281 (online: 14 April 2014)
Research Articles
Identification and molecular characterization of LTR and LINE retrotransposable elements in Fagus sylvatica L.
vol. 2, pp. 119-126 (online: 10 June 2009)
Research Articles
Dead wood quality influences species diversity of rare cryptogams in temperate broadleaved forests
vol. 9, pp. 276-285 (online: 28 September 2015)
Research Articles
Near zero mortality in juvenile Pinus hartwegii Lindl. after a prescribed burn and comparison with mortality after a wildfire
vol. 12, pp. 397-402 (online: 31 July 2019)
iForest Database Search
Google Scholar Search
Citing Articles
Search By Author
- J Lábusová
- C Morrissey Robert
- V Trotsiuk
- P Janda
- R Bače
- V Cada
- M Mikoláš
- H Mrhalová
- S Schurman Jonathan
- K Svobodová
- L Mateju
- M Synek
- M Svoboda
Search By Keywords