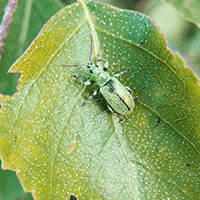
Response of artificially defoliated Betula pendula seedlings to additional soil nutrient supply
iForest - Biogeosciences and Forestry, Volume 10, Issue 1, Pages 281-287 (2016)
doi: https://doi.org/10.3832/ifor2086-009
Published: Dec 13, 2016 - Copyright © 2016 SISEF
Research Articles
Abstract
The impact of leaf damage on the growth of young silver birch seedlings with and without additional nutrient supply was investigated by simulating leaf-insect damage and applying different levels (25%, 50% and 75%) of artificial defoliation. Based on field-practical and cost-effective methods, we determined how fertilization practices compensate for foliage loss, and the combined effect on silver birch seedling growth. The mineral fertilizers applied to the 25-75%-defoliated silver birch seedlings reduced the growth in aboveground biomass compared to the fertilized but undamaged seedlings. Our results showed that when the birch seedlings received more nutrients they did not compensate for the loss of foliar mass. However, the seedlings loosing part of their foliar mass and receiving no additional fertilizers did compensate for the foliage loss and their root growth was not weakened, using soil nutrients more effectively. Mineral fertilization up to optimal nutritional balance could be a beneficial tool for increasing growth rate and biomass accumulation in the short-term period. However, our study demonstrated that additional fertilization does not necessarily lead to growth compensation of partly defoliated young birch trees.
Keywords
Betula pendula, Artificial Defoliation, Fertilization, Aboveground Biomass, Photosynthesis
Introduction
Numerous studies have investigated the effects on vegetation growth of environmental stresses due to different combinations of abiotic and biotic agents ([3], [41], [5], [6], [26], [19]). Several decades ago, Vasechko ([39]) argued that pest outbreaks are the consequence of decreased forest resistance and ecosystem stability. Nevertheless, the decline of forest condition may be induced by several environmental factors; in an ecological perspective, the stability of forests lies on their capacity to face unfavorable conditions ([39]). Recently, climate change and the occurrence of new invasive species have been considered additional factors further threatening forest stability ([30], [24], [15]).
Plant response to herbivores is affected by various environmental factors, including the soil nutrient status. Mutikainen et al. ([25]) reviewed the carbon-nutrient balance hypothesis, which explains the variation of herbivore-induced resistance in terms of different soil fertility and light regimes. Defoliation can affect the carbon-nutrient balance of plants by removing more nutrients than carbon, as observed both at the ecosystem level ([10], [27], [18]) and under a controlled environment ([17], [15], [20], [38]). Moreover, it has been suggested that fertilization is required for more intensive mineralization rates of organic nitrogen in soil under climate warming or increased N depositions ([21], [11], [40]).
The capacity of trees to allocate elements within their tissues has been widely discussed ([10], [4], [35]). Huttunen et al. ([16]) observed that defoliation might weaken the ability of roots to store resources, which is caused by changes in carbon allocation to aboveground tissues. Previous studies indicated that the ability of defoliated silver birch seedlings to recover from and compensate for the loss of leaf biomass was improved by fertilization ([25], [8]). For example, fertilization has increased the height increment and biomass growth of defoliated silver birch seedlings ([17]). As an opposite interaction, intensive photosynthesis and growth caused by defoliation may induce soil nutrient disproportions ([43], [36]).
The capability of birch species to exhibit compensatory growth and recover after severe defoliation was found to improve after increasing the nutrient supply ([31], [25]). Furthermore, it was reported that nutrient availability may affect how the total biomass responded to defoliation; however, no interaction between defoliation and fertilization was found in the second growing season after defoliation ([1]).
The study by Eyles et al. ([8]) examined the interactive effects of belowground resource limitations by varying nutrient and water availability, and aboveground carbon limitation imposed by a single 40% defoliation on stem growth, below- and aboveground biomass allocation patterns in 8-month-old, field-grown Eucalyptus globulus Labill. saplings. This study assumed that in the short term the trees grown with adequate water and nutrient supplies, and those grown with low-nutrient supply, were able to compensate for the foliage loss. However, the effect of defoliation on biomass and resource allocation patterns had not been fully realized after five months of recovery time ([8]).
Contrary to the widely discussed compensatory continuum hypothesis, which predicts lower tolerance under low soil nutrient supply ([22]), several studies have demonstrated that trees are less tolerant to defoliation under high rather than low soil nutrient supply ([12], [42]). The tolerance is also related to other environmental factors, such as damage frequency and severity ([14], [42], [29]). Stevens et al. ([33]) reported a positive correlation of aspen tolerance with the proportion of biomass in stems under low-nutrient conditions and no defoliation event; however, it was correlated with greater allocation to stems under high-nutrient conditions and defoliation event.
Erbilgin et al. ([7]) focused on the compensatory growth of trembling aspen seedlings under varying defoliations, with or without nutrient-enriched substrate, suggesting interactions between frequency and intensity of defoliation and nutrient availability. According to Erbilgin et al. ([7]), lower defoliation induced biomass accumulation of defoliated seedlings, regardless of nutrient availability. Otherwise, under higher defoliation, compensatory growth varied depending on nutrient availability.
This paper attempts to analyze the impact of simulated leaf damage, which was chosen to demonstrate leaf-insect damage and artificial defoliation up to 75% on the growth of young silver birch seedlings with and without additional nutrient (N, P, K and Mg) supply. We hypothesized that mechanical defoliation is an appropriate method for the compensatory growth studies of trees ([2]) and that the type of insect damage, such as leaf-chewing or leaf perforation, is more important than how this damage was conducted ([23]). Although silver birch grows on a wide range of soil conditions ([37]), the increase of final silver birch biomass could be improved by soil nutrient supply. In parallel, this could prevent foliar biomass loss caused by insects. If soil fertilization leads to a positive outcome, the following technique could be applied in the field when birch plantations are planned or managed.
Material and methods
Field experiment
Throughout the vegetation season of 2015, silver birch (Betula pendula Roth) seedlings that originated from central Lithuania (54° 51′ N, 24° 03′ E) were grown at the Dubrava Experimental and Training Forest Enterprise. One-year-old silver birch seedlings were planted into individual 4-L plastic pots filled with a mixed substrate (mixture of sand and neutralized peat in a ratio 1:5, respectively) at the beginning of April 2015. The mean height of selected birch seedlings was 45.7 ± 0.2 cm and mean diameter at 2 cm above ground level was 4.17 ± 0.04 mm, normally developed, with no visual damage. All seedlings were irrigated as needed throughout the summer. Pots had perforations in the base to allow excess water to drain.
The seedlings were randomly assigned into fertilized, which received 120 kg ha-1 nitrogen, 90 kg ha-1 phosphorus, 135 kg ha-1 potassium and 35 kg ha-1 magnesium, and unfertilized seedlings. The applied amount of NPKMg fertilizers corresponded to the optimal amount of fertilizers commonly used for one-year-old birch seedlings. The optimal fertilizer dosage for the particular substrate was experimentally defined at the Agrochemical laboratory of Lithuanian Research Center for Agriculture and Forestry. Granulated NPK fertilizer was mixed to substrate as raw material, leaving it to dissolve gradually during the vegetation season, while Mg fertilizer was dissolved in water. All fertilizers were applied once over a period of six-week after planting of birch seedlings.
In the middle of June 2015, fertilized and unfertilized seedlings were allocated to 25, 50 and 75% artificial defoliation, and three treatments simulating insect damage (three or six holes per leaf, or clipping one-third off each leaf) were conducted. The defoliation was conducted using scissors, each leaf was damaged with three or six non-overlapping holes (0.33 cm2) using a steel hole-punch, or one-third of each leaf was removed using scissors. Defoliation treatments were applied once. In total, seven treatments were conducted, including a non-defoliated control. No visible injury caused by biotic or abiotic agents was observed during the experimental period. Each treatment had 10 replicates, a total of 140 seedlings were grown during the experiment.
The potted trees were placed adjacent to each other in rows mixing the treatments, covering an experimental area of 130 × 360 cm. The artificial defoliation was designed to approximate the actual levels of insect defoliation during the active vegetation period in Lithuania. The mean temperature of the 2015 growing season in the study area was 14.4 °C, ranging from 7.3 °C in April to 20.1 °C in August (data from the meteorological station situated 0.5 km away from the study area). The mean precipitation was 23.8 mm, with 43.2 mm in April, 42.0 mm in May, 10.0 mm in June, 21.2 mm in July and 2.6 mm in August. These meteorological values were close to the standard climatic norm.
Measurements
The height of birch seedlings was measured periodically 1, 5 and 10 weeks post-defoliation on June 29, July 30 and August 30, respectively.
The net photosynthetic rate of the remnant leaves was measured two weeks post-defoliation (July 10) with a portable photosynthetic system LCpro-SD® (ADC BioScientific Ltd, England). The measurements were conducted between 10:00 AM and 12:00 PM, having similar environmental conditions for all samples. Three seedlings were systematically selected and measured from all fertilized and unfertilized treatments. The photosynthetic rate was measured for three leaves from each seedling, yielding nine leaves per treatment. We measured mature, sunlit, naturally undamaged leaves in each treatment.
The diameter of the main stem of each individual seedling was measured at a distance of 2 cm above the stump base using an electronic digital caliper before final harvesting.
The above- and belowground biomass of seedlings was estimated at the end of the experiment, i.e., on September 02, or approximately 10 weeks post-defoliation. For the determination of total dry mass, samples were removed from the following tree compartments: leaves, living and dead shoots, branches, stems and roots. Roots were rinsed free of soil and oven-dried to determine the total belowground biomass. The samples were transported to the laboratory in paper bags and stored in a ventilated room. The oven drying took place in the weeks immediately after sampling. The removed biomass from each treatment, as well as the final live biomass, was collected and oven-dried to a constant weight at 60 °C to determine the accumulated aboveground biomass.
Calculations and statistical analysis
The total aboveground biomass of each tree was calculated by summing the masses of dried shoots, branches and leaves. The total biomass of each seedling was calculated by summing the above- and belowground biomasses. Leaf, aboveground, and cumulative dry mass were calculated by summing the dry mass at harvest plus the leaf mass removed during defoliation. Root biomass was expressed in relation to the aboveground biomass, and the root-to-shoot ratio was calculated. Cumulative leaf dry mass in relation to the root was calculated, and the ratio of leaf production-to-root ratio was obtained.
Growth compensation of seedlings (i.e., whether they partially or fully compensated for the removal of leaf biomass) was assessed by comparing the height increment, photosynthetic rate and final biomass of defoliated seedlings with the same variables measured in the control seedlings both during the growing period and at final harvest.
Normal distribution of the above variables was tested using the non-parametric Lilliefors and Kolmogorov-Smirnov tests. Due to non-normal distribution of data, we used the Kruskal-Wallis analysis of variance (KW-ANOVA) test to ascertain the significance of differences in dry mass between treatments. Treatment means are presented throughout the study with the standard error of the mean (±SE).
All statistical analyses were conducted using the software package STATISTICA® ver. 7.0 (StatSoft Inc., Tulsa, OK, USA) with α = 0.05 in all cases.
Results
Effect on height and stem growth
To compare the growth of fertilized and unfertilized one-year-old silver birch seedlings, the height increment was measured just before harvesting. The increment in height of fertilized seedlings was 1.5 times higher than that of unfertilized seedlings, decreasing from 32.1 ± 1.2 (the control) by 20-23% (25-50% defoliation). In the case of fertilized birch trees, 25-75% defoliated seedlings reached a lower height increment compared with the fertilized control (Fig. 1). These results suggest a slight height decrease with more severe damage.
Fig. 1 - Relationship between the percentage change in height from control (0% is equal to control) and defoliation (%) in fertilized and not fertilized silver birch seedlings at harvest.
Similar tendencies were recorded for the stem increment. The fertilization increased stem growth by 3-4 times compared to unfertilized seedlings (Fig. 2). In the case of unfertilized seedlings, both artificial insect damage (three, six holes and clipped one-third leaf, which correspond to 10, 20 and 27% defoliation, respectively) and all defoliation treatments (25, 50 and 75%) did not significantly influence the stem growth at harvest. However, in the case of fertilized trees, defoliation of about 25% significantly increased stem growth by 20-35% compared to fertilized control.
Fig. 2 - Relationship between the percentage change in stem diameter from control (0% is equal to control) and defoliation (%) in fertilized and non-fertilized silver birch seedlings at harvest.
Effect on leaf photosynthesis intensity
Photosynthesis intensity of unfertilized seedlings varied depending on the damage intensity, with a tendency to increase with increasing defoliation (Fig. 3). Three weeks post-treatment, the photosynthetic rate of unfertilized seedlings was 7.8 times higher in the 75% defoliated unfertilized seedlings compared to non-defoliated unfertilized control. However, no significant impact of defoliation on photosynthesis intensity was found in the fertilized seedlings.
Fig. 3 - Leaf photosynthetic rate (μmol m-2 s-1) of fertilized and unfertilized silver birch seedlings at increasing defoliation percentage.
Effect on final above- and belowground biomass
At harvest, differences between the total production of fertilized and unfertilized seedlings were the most significant (Tab. 1). According to the treatment intensity, total productivity of fertilized seedlings was 2.1 (50% defoliation) to 4.9 (control) times higher than the final productivity of unfertilized seedlings. Total productivity of unfertilized seedlings varied in a narrow range from 5.55 ± 0.51 (75% defoliation) to 6.72 ± 0.66 g (50% defoliation). In the case of unfertilized seedlings, no significant differences between the treatments (all defoliation levels) were recorded. All unfertilized seedlings grew at a similar intensity, and were able to compensate for leaf biomass loss at final harvesting.
Tab. 1 - Dry mass (g) of leaves, shoots, stems and roots of fertilized and non-fertilized B. pendula seedlings at harvest. Values are means ± SE (n=10). Different letters within a row indicate significant differences in dry mass between treatments after Kruskal-Wallis ANOVA (p<0.05). (1): Leaf production include leaf lost in defoliation (cumulative dry mass); (2): Total production by summing the dry mass at harvest plus the leaf mass removed in defoliation events; (3): Gram roots / (gram stems + gram shoots + gram leaf).
Variable | Fertilizers | Control | Artificial defoliation / simulated insect damage |
Artificial defoliation | ||||
---|---|---|---|---|---|---|---|---|
10% / 3 holes per leaf |
20% / 6 holes per leaf |
27% / clipped 1/3 of leaf |
25% | 50% | 75% | |||
Leaf mass (g) | Yes | 6.03 ± 0.54 c | 4.57 ± 0.50 bc | 3.29 ± 0.36 abc | 3.77 ± 0.41 abc | 3.79 ± 0.61 abc | 2.41 ± 0.32 ab | 2.26 ± 0.23 ab |
No | 0.85 ± 0.32 ab | 1.06 ± 0.18 b | 0.62 ± 0.06 ab | 0.86 ± 0.09 ab | 0.71 ± 0.08 ab | 0.75 ± 0.13 ab | 0.48 ± 0.12 a | |
Leaf production (g)1 | Yes | 6.03 ± 0.54 b | 4.91 ± 0.50 ab | 3.92 ± 0.36 ab | 4.38 ± 0.41 ab | 4.44 ± 0.61 ab | 3.63 ± 0.32 a | 3.51 ± 0.23 a |
No | 0.85 ± 0.32 b | 1.19 ± 0.18 ab | 0.83 ± 0.06 ab | 1.03 ± 0.09 ab | 0.81 ± 0.08 ab | 1.28 ± 0.13 ab | 1.26 ± 0.12 a | |
Shoot mass (g) | Yes | 2.18 ± 0.27 b | 1.26 ± 0.19 ab | 0.85 ± 0.10 a | 1.02 ± 0.14 ab | 0.89 ± 0.13 a | 0.77 ± 0.14 a | 0.93 ± 0.13 a |
No | 0.11 ± 0.04 a | 0.16 ± 0.03 a | 0.11 ± 0.01 a | 0.13 ± 0.02 a | 0.09 ± 0.04 a | 0.15 ± 0.06 a | 0.10 ± 0.02 a | |
Stem mass (g) | Yes | 8.91 ± 0.54 b | 7.10 ± 0.69 ab | 5.80 ± 0.61 ab | 5.98 ± 0.52 ab | 5.95 ± 0.70 ab | 4.68 ± 0.38 a | 5.44 ± 0.46 a |
No | 2.43 ± 0.36 a | 2.59 ± 0.18 a | 2.29 ± 0.17 a | 2.47 ± 0.15 a | 2.71 ± 0.21 a | 2.58 ± 0.20 a | 2.18 ± 0.12 a | |
Aboveground mass (g) | Yes | 17.13 ± 1.31 b | 12.93 ± 1.33 ab | 9.95 ± 1.04 a | 10.77 ± 0.98 ab | 10.63 ± 1.42 ab | 7.85 ± 0.75 a | 8.63 ± 0.80 a |
No | 3.39 ± 0.72 a | 3.79 ± 0.34 a | 3.01 ± 0.22 a | 3.46 ± 0.26 a | 3.51 ± 0.28 a | 3.48 ± 0.36 a | 2.76 ± 0.23 a | |
Aboveground production (g) | Yes | 17.13 ± 1.31 b | 13.28 ± 1.33 ab | 10.57 ± 1.04 ab | 11.38 ± 0.98 ab | 11.28 ± 1.42 ab | 9.07 ± 0.75 a | 9.88 ± 0.80 a |
No | 3.39 ± 0.72 a | 3.39 ± 0.34 a | 3.22 ± 0.22 a | 3.63 ± 0.26 a | 3.61 ± 0.28 a | 4.01 ± 0.36 a | 3.54 ± 0.23 a | |
Root mass (g) | Yes | 10.34 ± 0.72 b | 7.84 ± 0.74 ab | 6.11 ± 0.72 a | 6.99 ± 0.53 ab | 6.54 ± 0.74 ab | 4.87 ± 0.46 a | 5.31 ± 0.50 a |
No | 2.23 ± 0.42 a | 2.75 ± 0.24 a | 2.19 ± 0.17 a | 2.41 ± 0.24 a | 2.69 ± 0.27 a | 2.71 ± 0.31 a | 2.01 ± 0.30 a | |
Total mass (g) | Yes | 27.47 ± 2.02 b | 20.77 ± 2.06 ab | 16.05 ± 1.73 a | 17.76 ± 1.48 ab | 17.18 ± 2.07 ab | 12.73 ± 1.18 a | 13.94 ± 1.28 a |
No | 5.62 ± 1.12 a | 6.54 ± 0.57 a | 4.96 ± 0.29 a | 5.87 ± 0.48 a | 6.20 ± 0.53 a | 6.19 ± 0.66 a | 4.77 ± 0.51 a | |
Total production (g)2 | Yes | 27.47 ± 2.02 b | 21.11 ± 2.06 ab | 16.68 ± 1.73 a | 18.37 ± 1.48 ab | 17.83 ± 2.07 ab | 13.95 ± 1.18 a | 15.19 ± 1.28 a |
No | 5.62 ± 1.12 a | 6.67 ± 0.57 a | 5.17 ± 0.29 a | 6.04 ± 0.48 a | 6.30 ± 0.53 a | 6.72 ± 0.66 a | 5.55 ± 0.51 a | |
Root/shoot ratio3 | Yes | 0.61 ± 0.02 a | 0.60 ± 0.02 a | 0.57 ± 0.04 a | 0.62 ± 0.02 a | 0.59 ± 0.03 a | 0.54 ± 0.02 a | 0.53 ± 0.02 a |
No | 0.67 ± 0.05 a | 0.70 ± 0.02 a | 0.64 ± 0.09 a | 0.66 ± 0.03 a | 0.74 ± 0.04 a | 0.67 ± 0.04 a | 0.56 ± 0.04 a |
Because tree foliage was damaged - seedlings lost up to 75% of leaves - it could be expected that main direct changes were observed in the leaf or aboveground biomass. However, indirect effects could also occur in the roots or even in the total tree biomass. The total leaf mass of unfertilized seedlings that were artificially damaged by perforation with three holes per leaf (or 10% defoliated) exceeded the leaf mass of the control seedlings by 1.2 times, while leaf production was exceeded by 1.4 times. Seedlings defoliated by 75% were particularly able to compensate for leaf mass loss, damaged seedlings exceeding the control by 1.5 times (Fig. 4, Fig. 5). In all other cases (all defoliation treatments), growth compensation was also evident: no significant difference from the control indicated positive growth response.
Fig. 4 - Relationship between dry leaf mass (g) and defoliation (%) in fertilized and non-fertilized silver birch seedlings at harvest. Grey boxes represent the range of dry leaf mass values observed for control seedlings.
Fig. 5 - Relationship between the percentage change in leaf production from control (0% is equal to control) and defoliation (%) in fertilized and non-fertilized silver birch seedlings at harvest.
The highest total production of fertilized seedlings was found in the control treatment (Fig. 4). Opposite to unfertilized seedlings, fertilized trees were not able to compensate for leaf mass loss in all cases. Severe defoliation of 50-75% induced about 1.8-2.0 times lower total production compared to the control. Only weak damage (three holes per leaf, which corresponded to 20% defoliation; also 25% defoliation) induced final biomass similar to the control. However, such growth response could be evaluated only as partial compensation. Similar patterns were found in leaf biomass: at final harvest, the leaf production in 50-75% damaged seedlings remained lower than in the control (Fig. 5).
Biomass growth and allocation to different tree compartments of fertilized and unfertilized silver birch seedlings were quite similar for both damaged and control seedlings (Fig. 6a, Fig. 6b). In the case of applied fertilizers, the biomass was allocated more to stems and roots compared to unfertilized seedlings. The percentage of leaf biomass depended on damage intensity and varied from 13-15% to 23%. Similarly, a wider range of biomass allocated to stems was recorded in the case of no fertilization.
Fig. 6 - Final biomass allocation of fertilized (A) and unfertilized (B) silver birch seedlings at harvest.
Discussion
This study clearly demonstrated that additional nutrient supply is the most important factor for the growth of birch seedlings, both in height and diameter. This is in agreement with most general assertions, which emphasize optimal nutrition as a limiting factor for vegetation. Several previous studies have confirmed the essential role of N as a key nutrient for growth ([34], [9], [13], [35], [27]), but the addition of other macronutrients could induce a higher incremental response than N alone ([9], [32], [28]). In this study, height and stem diameter of birch seedlings increased by several times as a response to fertilization. Measurement of aboveground biomass and root at harvest also showed very similar trends. Although we found that soil fertilization induced a positive growth response in silver birch seedlings, this cannot be extended to older trees, short-term responses being different to long-term change in tree vitality.
We initially assumed that fertilized trees respond differently to various damage or foliage loss. To this purpose, we applied various defoliation levels (10-75%) to silver birch seedlings. In addition, 10, 20, and 27% defoliation was conducted by simulating leaf perforations and clipping part of all leaves per tree, corresponding to three and six holes per leaf and one-third clipped off each leaf, respectively. When the latter leaf damages (leaf holes and clipping) were compared with simple defoliation treatments, when 25, 50, and 75% of leaves were removed, it was obvious that no significant differences between the two groups of treatments were recorded.
In our study, we determined how lost foliage together with fertilization affects growth compensation in silver birch seedlings. Following Maschinski & Whitham ([22]), Mutikainen et al. ([25]), Huttunen et al. ([17]) and Eyles et al. ([8]), we presumed that defoliated silver birch seedlings grow more intensively and had higher compensation potential when the soil was fertilized. However, our findings did not prove that mineral fertilization up to optimal soil fertility for birch species does compensate the lost foliage at harvest. When the seedlings were fertilized, the increase of both the above- and belowground biomasses showed under compensation compared to the unfertilized control. The seedlings that were artificially defoliated up to 30-40% did compensate for the lost foliage more intensively than those that had higher defoliation. This non-conformity was very clear when estimating the percentage change of leaf production in comparison with the control (Fig. 5). The reduced compensation was followed by defoliation increase (R2 = 0.704); a similar trend was found for root biomass (R2 = 0.648).
Nonetheless, the unfertilized birch seedlings under the possible synergetic influence of high defoliation and nutrient limitation showed relatively good growth potential. Indeed, the severe 50-75% defoliation resulted in higher photosynthesis, and the seedlings grown without additional fertilization showed higher compensation potential. In particular, this effect was significant for higher damage levels. However, we did not measure the impact of repeated damage during the next vegetation periods, which could cause quite different growth variations, especially if seedlings had severe damage during the previous growth season.
These patterns indicate that seedlings of fast growing tree species, such as silver birch, have relatively high potential to respond to various environmental disturbances. However, even in the case of severe damage, additional fertilization does not necessarily lead to more intensive growth. Mineral fertilization up to optimal nutritional balance could be a beneficial tool causing more intensive growth, higher biomass during the vegetation season, short-term response and partial compensation for lost foliage in the case of insect outbreak.
Conclusions
The mineral fertilizers applied for the 25-75%-defoliated silver birch seedlings reduced the growth of aboveground biomass compared with the fertilized but undamaged seedlings. Our results showed that when seedlings received more nutrients they did not compensate for the loss of foliar mass. Meanwhile, the seedlings that lost a part of their foliar mass but received no additional fertilizers, compensated for the lost foliage and have not weakened the root growth, using soil nutrients more effectively.
References
Gscholar
Gscholar
Gscholar
Gscholar
Authors’ Info
Authors’ Affiliation
Iveta Varnagiryte-Kabašinskiene
Vidas Stakenas
Institute of Forestry, Lithuanian Research Centre for Agriculture and Forestry, Liepu str. 1, Girionys, LT-53101, Kaunas district (Lithuania)
Corresponding author
Paper Info
Citation
Araminiene V, Varnagiryte-Kabašinskiene I, Stakenas V (2016). Response of artificially defoliated Betula pendula seedlings to additional soil nutrient supply. iForest 10: 281-287. - doi: 10.3832/ifor2086-009
Academic Editor
Gianfranco Minotta
Paper history
Received: Apr 12, 2016
Accepted: Nov 13, 2016
First online: Dec 13, 2016
Publication Date: Feb 28, 2017
Publication Time: 1.00 months
Copyright Information
© SISEF - The Italian Society of Silviculture and Forest Ecology 2016
Open Access
This article is distributed under the terms of the Creative Commons Attribution-Non Commercial 4.0 International (https://creativecommons.org/licenses/by-nc/4.0/), which permits unrestricted use, distribution, and reproduction in any medium, provided you give appropriate credit to the original author(s) and the source, provide a link to the Creative Commons license, and indicate if changes were made.
Web Metrics
Breakdown by View Type
Article Usage
Total Article Views: 46324
(from publication date up to now)
Breakdown by View Type
HTML Page Views: 40238
Abstract Page Views: 2058
PDF Downloads: 2889
Citation/Reference Downloads: 48
XML Downloads: 1091
Web Metrics
Days since publication: 3133
Overall contacts: 46324
Avg. contacts per week: 103.50
Article Citations
Article citations are based on data periodically collected from the Clarivate Web of Science web site
(last update: Mar 2025)
Total number of cites (since 2017): 1
Average cites per year: 0.11
Publication Metrics
by Dimensions ©
Articles citing this article
List of the papers citing this article based on CrossRef Cited-by.
Related Contents
iForest Similar Articles
Research Articles
Effects of artificial defoliation and simulated insect damage on the growth of Betula pendula saplings
vol. 9, pp. 95-100 (online: 15 July 2015)
Research Articles
Individual tree mortality of silver birch (Betula pendula Roth) in Estonia
vol. 9, pp. 643-651 (online: 04 April 2016)
Research Articles
Arbuscular mycorrhizal colonization in black poplar roots after defoliation by a non-native and a native insect
vol. 9, pp. 868-874 (online: 29 August 2016)
Research Articles
Equations for estimating belowground biomass of Silver Birch, Oak and Scots Pine in Germany
vol. 12, pp. 166-172 (online: 15 March 2019)
Research Articles
Combined pre-hardening and fall fertilization facilitates N storage and field performance of Pinus tabulaeformis seedlings
vol. 9, pp. 483-489 (online: 07 January 2016)
Research Articles
Nursery fertilization affected field performance and nutrient resorption of Populus tomentosa Carr. ploidy levels
vol. 15, pp. 16-23 (online: 24 January 2022)
Research Articles
Effects of defoliation by the pine processionary moth Thaumetopoea pityocampa on biomass growth of young stands of Pinus pinaster in northern Portugal
vol. 3, pp. 159-162 (online: 15 November 2010)
Research Articles
Influence of climate on tree health evaluated by defoliation in the ICP level I network (Romania)
vol. 10, pp. 554-560 (online: 05 May 2017)
Short Communications
Variation in growth, photosynthesis and water-soluble polysaccharide of Cyclocarya paliurus under different light regimes
vol. 10, pp. 468-474 (online: 04 April 2017)
Research Articles
Conservation of Betula oycoviensis, an endangered rare taxon, using vegetative propagation methods
vol. 13, pp. 107-113 (online: 23 March 2020)
iForest Database Search
Search By Author
Search By Keyword
Google Scholar Search
Citing Articles
Search By Author
Search By Keywords
PubMed Search
Search By Author
Search By Keyword