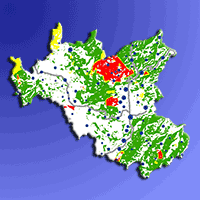
Identifying priority conservation areas for above-ground carbon sequestration in Central Mexico
iForest - Biogeosciences and Forestry, Volume 10, Issue 6, Pages 923-929 (2017)
doi: https://doi.org/10.3832/ifor1980-010
Published: Dec 07, 2017 - Copyright © 2017 SISEF
Research Articles
Abstract
Identifying forest ecosystems with significant ecological, social, and/or economic values is an important first-step in conserving landscape function. Here, we identify priority conservation areas in the municipalities of Chignahuapan and Zacatlan, Puebla (Mexico), based on: (i) their capacity to sequester atmospheric CO2; and (ii) risk of future deforestation. We also explore management strategies for priority-lands conservation in the Mexican context. Above-ground C sequestration was estimated using wood density and biomass expansion-factor data available from local forestry sources. Deforestation risk was estimated by a probabilistic model of land use change using socioeconomic and biophysical variables. Carbon sequestration estimates ranged from 14 to 531 Mg ha-1 for Chignahuapan and Zacatlan, respectively. An estimated 11 746 and 4 406 ha of forest was determined to be at risk of deforestation in each municipality. Of these at-risk lands, 2 421 and 1 798 ha were determined to be at high risk. In combination, we determined that 10 687 and 4 319 ha, respectively, are priority lands for carbon sequestration in Chignahuapan and Zacatlan, of which 628 and 310 ha were determined to have high conservation priority. Identifying priority conservation areas through the integrated assessment of carbon sequestration and deforestation risk can enhance efforts to target land management strategies to mitigate climate change impacts. This approach can serve as a model for other forested regions in Mexico and other countries.
Keywords
Forest Carbon Sinks, REDD, Climate Change, Deforestation Risk, Priority Conservation, Probabilistic Model, Land Use, Development
Introduction
The role of forests in mitigating climate change has taken on increased attention in recent years. Initially, international efforts focused on reducing greenhouse gas (GHG) emissions from industrialized countries (e.g., Kyoto Protocol, Paris COP). Early work sought to establish baseline GHG inventories under the Intergovernmental Panel on Climate Change (IPCC). Data show that from 1970 to 2014, emissions increased by 90% (49 MMTCO2 yr-1 - [4]). More recently, efforts have broadened to include a portfolio of mitigation strategies, including flexible mechanisms such as emissions trading, clean development, and carbon accounting, the latter of which seeks to quantify the amount of carbon absorbed by forests and other natural carbon sinks ([5]).
Mexico emits more than 553 million tons per year of greenhouse gases (GHG) as a result of industry, farming, and forest degradation and deforestation. This total represents 1.5% of global emissions. Of this, degradation and deforestation account for 12.4% of Mexico’s total GHG emissions. In response, Mexico pledged in 2009 to limit 2050 GHG emissions to 50% of year 2000 emissions through its newly established Special Climate Change Program or PECC, for its Spanish acronym ([25]).
To reduce emissions, governmental and non-governmental institutions initiated various measures, such as sustainable forest management, Reducing Emissions from Deforestation and forest Degradation (REDD) pilot projects, payment for environmental services, wildlife management units, protected natural areas in forest ecosystems, sustainable planned grazing, and commercial forest plantations ([22], [10], [11]).
To optimize resources and ensure effectiveness, a strategic forest planning approach is needed to identify and protect forested areas with high carbon sequestration ([18]). These priority conservation areas are spatial representations of the territory defined by environmental, social and cultural characteristics whose presence is threatened by human activities, natural causes, or both ([9], [38]). The designation of priority conservation areas for carbon sequestration have already been used to promote the reduction of high rates of deforestation and restore degraded areas ([43], [1]) - a strategy that arose from collaboration between foreign research groups and Mexican researchers ([6], [41]).
Here, we develop an integrated methodology to quantify both existing forest-level carbon sequestration, and risk of deforestation. This combination is then used to identify priority conservation areas for natural resource protection.
Materials and methods
Our research comprised three stages: (1) determining carbon sequestration; (2) estimating risk of deforestation; and (3) delineation and comparative analysis of priority conservation areas for carbon sequestration. We focus on the Chignahuapan-Zacatlan region because it represents the primary forest area of the Sierra Norte mountain range of the State of Puebla (Mexico).
Study area
The municipalities of Chignahuapan and Zacatlan are located in the Sierra Norte de Puebla, a mountainous, upland region of Central Mexico (19° 39′ 42″ - 20° 08′ 12″ N and 97° 51′ 06″ - 98° 18’ 06″ W). The 1250 km2 study area is part of the Trans-Mexican volcanic belt composed of rugged mountain ranges with some intermediate plains. Peaks range from 2300 to 4200 m. The study area has a cold, temperate climate, with annual precipitation ranging from 600 to 1500 mm ([14]). The region is dominated by pine-oak forests, with 27.2% covered by coniferous forests.
The combined population in both municipalities is 115.456 inhabitants, of which 15% belong to an ethnic indigenous group that generally reside within areas of mountainous forest. The two municipalities include 289 discrete settlements, of which five are considered urban areas. The marginalization and human development indices for the study area are 0.16 and 0.74, respectively, indicating limited social capital and opportunities, and low levels of education, income, and life expectancy ([12]). For comparison, at the State level, these indexes are 0.71 and 0.77, respectively. Agriculture and forestry are dominant land uses, comprising the bulk of economic activity. Most agricultural and forest lands are under ejido tenure regimes. At the State level, Chignahuapan accounts for 45% of total timber harvests, while Zacatlan contributes another 9%. Combined, the two municipalities support over half of the forest sector, including many jobs.
Timber harvests in the study area were prohibited by federal law from 1947 to 1975. The policy was intended to limit deforestation and protect important environmental functions. Unfortunately, in the absence of management, deforestation rates increased, although data for the region from that era are lacking. After 1975, forests were managed under two systems to meet multiple use objectives including timber production: the “Mexican Method of Forest Regulation” (MMOM), and the “Method for Silvicultural Development” (MDS - [45]). Management led to reduced deforestation rates, estimated at around 3.3% per year today (for comparison, deforestation at the country level is 0.24% per year). Deforestation pressures are greatest near existing settlements, where forests are cleared for agriculture and residential use.
Determining carbon sequestration
Above-ground carbon sequestration in the forests of the study area was determined using forest inventory data from lands with forest management programs, avoiding the need for primary data or destructive sampling ([36], [40], [17]). Inventory data on 24 and 28 Chignahuapan and Zacatlan forest lands covering an area of 20.187 and 6.124 ha, respectively, was collected. Most lands were covered by pine forests dominated by Pinus patula, although forests included sacred fir (Abies religiosa), mixed pine-oak (Pinus spp. - Quercus spp.), and oak forests.
Following the documentary and spatial identification of forest types, the carbon in the above-ground biomass was calculated as follows ([36] - eqn. 1):
where C
AB is the carbon content in above-ground biomass (Kg CO2 ha-1); V
is the wood volume (m3 ha-1); WD
is the wood density (Kg m-3); BEF
is the biomass expansion factor; and CF
is the carbon fraction.
Eqn. 1 is conservative in its estimates of carbon because it only includes volume from tree species with marketable timber diameters, excluding aboveground biomass from unmerchantable species, trees with diameters less than 15 cm DBH, brushwood, and foliage ([15]). Other important forest compartments not considered include deadwood, litterfall, below-ground plant biomass and soil carbon ([48], [42]).
The expansion factor, BEF
, was used to represent biomass components that were difficult to obtain from direct measurement (e.g., branches and leaves). The BEF
reference values, based on previous studies by Caballero et al. ([5]) and Silva & Návar ([44]), were as follows: Pinus spp. (1.38), Quercus spp. (1.5), Abies spp. (1.3), and broadleaf species (1.3). Wood density (WD
) for each species in eqn. 1 was obtained from a variety of sources reported in Tab. 1 (i.e., [19], [23], [10], [3]).
Tab. 1 - Wood density of timber species in the municipalities of Chignahuapan and Zacatlan, Puebla (Sources: [19], [23], [10], [3]).
Species | Density (kg m-3) |
---|---|
Abies religiosa | 380 |
Broadleaf species | 600 |
Pinus pseudostrobus | 550 |
Pinus patula | 430 |
Pinus ayacahuite | 340 |
Pinus montezumae | 460 |
Pinus hartwegii | 496 |
Pinus leiophylla | 485 |
Pinus teocote | 480 |
Pinus rudis | 530 |
Quercus spp. | 760 |
As the dry weight of carbon in biomass ranges from 0.45 to 0.55 ([30]), our research used a mid-range value of carbon fraction (CF
) of 0.50 ([24]). However, this default value could underestimate the overall carbon fraction ([31]). Finally, carbon values were then converted to CO2 using the conversion factor (44/12). Once above-ground biomass by species was established, it was expressed as carbon units per hectare by municipality using Microsoft Office Excel®.
The Mg CO2 database was joined to the polygon database of forest lands using the Join command in ArcGIS® ver. 9.3; it was deployed in ArcGis ArcMapTM ver. 9.3 and interpolated to the total forest area of Chignahuapan (29 401 ha) and Zacatlan (28 039 ha) using the Kriging method ([39]). The magnitude of the stored carbon was categorized into three intervals or classes using the Reclassify command located in the Spatial Analyst module within ArcGis ver. 9.3. The final product was a map with average carbon sequestration categories: (1) high (from 218 to 531 Mg CO2 ha-1); (2) medium (from 96 to 218 Mg CO2 ha-1); and (3) low (from 0 to 96 Mg CO2 ha-1). These categories were established according to the data distribution without using thresholds criteria.
Estimating risk of deforestation
A probabilistic model of land use (eqn. 2) was used to estimate deforestation risk (dependent variable) based on 21 socioeconomic and biophysical variables (independent variables, see Tab. S1 in Supplementary material - [21], [37]). These variables were selected based on their importance as drivers of land use change between 1986 and 2011 in the study area ([14] - eqn. 2):
where Ŷi is the probability that a hectare (100 × 100 m) changes from forest use to another use; i = forest, agriculture, livestock or residential use; e
is the base of the natural logarithm; β
0, β
i are intercept and estimators of the independent variables (x
i); and X
i are independent variables (X
1, X
2, …, X
21).
The stepwise procedure of SAS® ver. 9.0 was used to construct the model. Once the probabilistic model was specified, it was used to estimate (in maps) the probabilities of land-use changes for the forest area of 2011 in each municipality.
Identification and comparative analysis of priority conservation areas for carbon sequestration
Priority areas for carbon sequestration were identified using the map algebra technique of the Spatial Analyst module and the Raster Calculator tool ([47]). This technique allows calculating scenarios from two combined criteria. For example, a high-priority CO2 conservation area results from combining an area that has high carbon sequestration and a high risk of deforestation. The normalized criteria for carbon sequestration were: (1) high (from 0.66 to 1); (2) medium (from 0.33 to 0.66); and (3) low (from 0 to 0.33). The same classes were used for categorizing deforestation risk ([13], [14]), although remote areas without risk of deforestation were not considered in the model. Tab. 2 shows the different combinations obtained.
Tab. 2 - Prioritization matrix of deforestation risk, carbon, and conservation. Risk of deforestation: high (from 0.66 to 1), medium (from 0.33 to 0.66), and low (from 0 to 0.33); Current carbon sequestration: high (from 218 to 531 Mg CO2 ha-1), medium (from 96 to 218 Mg CO2 ha-1), and low (from 0 to 96 Mg CO2 ha-1). (‡): Conservation areas priority is derived from the risk of deforestation and current carbon sequestration levels.
Risk of deforestation |
Current carbon sequestration |
Conservation areas priority (‡) |
---|---|---|
High | High | High |
High | Medium | High |
Medium | High | High |
High | Low | Medium |
Medium | Medium | Medium |
Low | High | Medium |
Medium | Low | Low |
Low | Medium | Low |
Low | Low | Low |
Finally, a comparative spatial analysis was conducted on deforestation risk area, carbon sequestration, and priority conservation areas for carbon sequestration in order to provide a quantitative overview for resource planners in our two study area municipalities.
Results and discussion
Estimating above-ground carbon sequestration
The municipality of Chignahuapan had CO2 sequestration estimates ranging from 14 Mg ha-1 to 410 Mg ha-1. The highest estimates were found in areas with oak forests (Quercus spp.), not because they capture more carbon, but rather because of selective silvicultural practices (e.g., harvesting, thinning, etc.) in pine stands that decreased carbon content. While some authors suggest that carbon sequestration is mainly a function of forest composition and age ([42]), we found no direct correlation (r = 0.049) between carbon sequestration and species richness (Tab. S2 in Supplementary material). This suggests that in our study area, carbon sequestration is dependent on tree age, size and volume rather than species. In Zacatlan, forests presented CO2 sequestration estimates ranging from 41 Mg ha-1 to 531 Mg ha-1, with an average of 158 Mg CO2 ha-1 and standard deviation of 8.7 (Tab. S2). Once again, the correlation between carbon sequestered and the number of species per management unit (Tab. S2) was low (r = 0.02).
An earlier study by Castellanos et al. ([8]) used a series of allometric equations to estimate that forests of Chignahuapan and Zacatlan store on average 81 to 150 Mg CO2 ha-1. Ruiz-Peinado et al. ([42]) estimated the total carbon present in the unthinned, moderately thinned and heavily thinned plots in Mediterranean maritime pines obtaining 317.256 and 234 Mg ha-1, respectively, estimates consistent with our findings.
Above-ground carbon sequestration
In the municipality of Chignahuapan, there are 29 401 ha of forest area, of which 3 480 ha (11.8%) were determined to have high carbon sequestrations suitability, i.e., current carbon sequestered from 218 to 531 Mg CO2 ha-1 (Tab. 3, Fig. 1a). In contrast, 17.5% of the forested area (5 157 ha) held 96 to 218 Mg CO2 ha-1 and was classified as medium. The balance (70.6 % or 20 764 ha of the total) was classified as low and held on average 82 Mg CO2 ha-1. These results are consistent with previous research by Angeles et al. ([2]) who found low carbon sequestration in forests with young trees and/or sparse vegetation, oftentimes as a result of harvesting activities (Fig. 1a).
Tab. 3 - Comparative data on current carbon sequestration, deforestation risk, and priority areas in the municipalities of Chignahuapan and Zacatlan, Puebla (Mexico). (‡): Differences between risk of deforestation and priority for CO2 sequestration areas are due to the use of buffers in the processing and categorization of satellite images and the models. For example, remote areas without development pressure were not considered in the modeling.
Criteria | Chignahuapan Area (ha and %) |
Zacatlan Area (ha and %) |
||||
---|---|---|---|---|---|---|
High | Medium | Low | High | Medium | Low | |
CO2 sequestration | 3 480 (11.8%) | 5 157 (17.5%) | 20 764 (70.6%) | 3 188 (11.4%) | 2 804 (10.0%) | 22 045 (78.6%) |
Deforestation risk (‡) | 2 421 (8.2%) | 4 585 (15.6%) | 4 740 (16.1%) | 1 798 (6.4%) | 2 271 (8.1%) | 337 (1.2%) |
CO2 sequestration priority (‡) | 628 (2.1%) | 2.876 (9.8%) | 7 183 (24.4%) | 310 (1.1%) | 1 744 (6.2%) | 2 265 (8.1%) |
Total forest area | 29 401 | 28 039 |
Fig. 1 - Above-ground C sequestration (CO2 Mg ha-1 - top), deforestation risk (middle), and priority conservation areas (bottom) for above-ground carbon sequestration in the municipality of Chignahuapan, Puebla (Mexico).
In Zacatlan, Fig. 2a shows that areas with high carbon sequestration are located in the north and center of the municipality, capturing around to 531 Mg CO2 ha-1. These areas are covered by trees of the genera Pinus and Quercus. Species, age and density affect CO2 sequestration rates; however, earlier research by Castañeda et al. ([7]) found that Quercus spp. forests stores on average 664 Mg CO2 ha-1. This finding that oak forests hold greater levels of carbon than pine forests is not surprising given the silvicultural regimes applied, where oaks are not intensively managed due to low commercial value. Areas with low sequestration averaged about 68 CO2 Mg ha-1, while areas with medium sequestration captured 164 CO2 Mg ha-1, based on a 50-year-old stand. Figueroa et al. ([16]) found that on average a 25-year-old stand of Pinus patula captures 86 CO2 Mg ha-1, which is similar to the values found in this study.
Fig. 2 - Above-ground C sequestration (CO2 Mg ha-1 - top), deforestation risk (middle), and priority conservation areas (bottom) for above-ground carbon sequestration in the municipality of Zacatlan, Puebla (Mexico).
Based on our analysis, managed forests in Chignahuapan and Zacatlan can function efficiently as carbon sequestration lands (sinks), since 12% of the forest area is classified as high carbon sequestration and 73% as low-current carbon sequestration, ranging from 2.7 Mg CO2 ha-1 to 164 Mg CO2 ha-1 (Fig. 2a). Finally, although both municipalities include areas classified as high, medium and low current carbon sequestration, their current carbon levels exhibit a wide variation due to the structure, management and volume of present species (Tab. 3).
Deforestation risk
Deforestation risk in Chignahuapan and Zacatlan was determined by a probabilistic model of land-use change. The model for Zacatlan included 21 significant variables (p≤0.05), while Chignahuapan included 16 significant variables. Discarded variables included: population density, distance to trails, distance to low marginalization, and land tenure. It is important to note that within the two models, the independent variables have different relative importance, though for both municipalities the minimum distance to agricultural areas (+), elevation (-), and slope (-) had the greatest impact on deforestation (Tab. S1 in Supplementary material).
There was no correlation between independent variables in the deforestation risk model, nor was the model affected by multicollinearity, having tolerances > 1 and variance inflation factors < 10. As a consequence, there is no linear relationship between the model’s independent variables ([33]). The adj-R2 presented in the model was > 20%, which indicates a low amount of variance explained. However, on average, about 80 % concordant observations were obtained by the model (Tab. 4), indicating that the independent and dependent variables are ordered in the same direction; therefore, the model is appropriate for predicting the phenomenon of interest ([20]). Moreover, due to the broad inclusion of both biophysical and socioeconomic variables, we deemed the model’s goodness-of-fit as adequate.
Tab. 4 - Measures of association of predicted probabilities and observed responses for the deforestation risk model for both municipalities. (VIF): Variance inflation factor.
Variable | Parameter/ Index |
Municipality | |
---|---|---|---|
Chignahuapan | Zacatlan | ||
Measures of association | Concordant | 85.9 | 76.3 |
Discordant | 13.2 | 22.9 | |
Bound | 0.9 | 0.8 | |
Pairs | - | 75 418 437 | 94 900 581 |
Correlation indices | Somers’ D | 0.72 | 0.53 |
Gamma | 0.73 | 0.53 | |
Tau-a | 0.16 | 0.15 | |
c | 0.86 | 0.76 | |
Adj-R2 (%) | - | 37.33 | 20.12 |
Multicollinearity | Tolerance | 0.63 | 0.8 |
VIF | 1.6 | 1.25 |
The map of deforestation risk for Chignahuapan (Fig. 1b) shows that 8.2% (2421 ha) of the total forest area (29.401 ha) in the municipality is at high risk of deforestation; these areas border agricultural and livestock areas (Fig. 1b). Also, 15.6% (4585 ha) of the total forest area was found to have medium risk, and just 16.1% (4740 ha) is at low risk. In Zacatlan, 1798, 2271 and 337 ha were assessed to be at high, medium and low risk of deforestation, respectively (Fig. 2b). The greatest deforestation threats are due to the conversion from forest to agriculture (forest-agriculture), grazing (forest-livestock), and development (forest-residential - [14]).
High-risk deforestation areas are located in the northwestern part of the municipality, while medium-risk areas are distributed throughout the municipality (Fig. 2b). In contrast, low-risk areas are located in the northern part of the municipality. This is likely due to forest fragmentation from agriculture and livestock activities, which increases the risk of increasing deforestation in the municipality. Finally, it is important to note that wildfire is a limited risk on managed forests within each municipality.
Priority areas for above-ground carbon sequestration
We integrated current carbon sequestration and deforestation risk to determine priority areas for conservation efforts (see Tab. 2). For Chignahuapan, we identified 628, 2876 and 7183 ha with high, medium and low conservation priority, respectively (Fig. 1c). Low-priority areas tended to be located in remote forest zones with little disturbance, while medium-priority areas were located near agricultural limits. High-priority areas are located in forest zones situated near livestock pasture, agricultural lands, and residential settlements. Our finding that the largest forest area is classified as having low conservation priority for carbon sequestration may be due in part to sound management practices by forest managers and their efforts to preserve healthy forests that provide sustained flows of environmental goods and services ([45]). As a result, both deforestation risk and current carbon sequestration are low or medium, largely due to periodic harvests which have reduced biomass levels.
In Zacatlan, 310, 1744 and 2265 ha were identified as high, medium and low conservation priority for carbon sequestration, respectively (Fig. 2c). These lands, distributed throughout the municipality, represent land areas that are roughly half in size of what we found in Chignahuapan (Fig. 1c).
Integrating current carbon sequestration and deforestation risk to identify conservation priority areas (Tab. 3) identified Chignahuapan as a focal region for conservation. This is due in part because forests in the municipality are at greater risk of deforestation due to high population densities for agricultural, livestock and residential uses to meet food, clothing, housing and future energy needs. For example, the population density (residents ha-1) for Chignahuapan and Zacatlan in the agricultural, livestock and residential areas was 1.1, 90.3, 70.8 in 1985 and rose up to 4.5, 11.6, 327.5 in 2010, respectively. Zacatlan’s population density is more than four times that of Chignahuapan, with the population of the former more concentrated ([12]).
By analyzing the forest carbon sequestration data in relation to the total forest area of the two municipalities, the results were found to be very similar because stands under forest use were analyzed. However, areas with high conservation priority for carbon sequestration in the municipality of Chignahuapan occupy 628 ha, representing 2% of the forest area of the municipality (Tab. 3). For comparison, Zacatlan has 310 ha identified as high priority, accounting for only 1% of the forest area.
The high, medium and low conservation priority categories for different forest management units were validated through field trips and consultation with Technical Forest Service Providers. Some authors agree that validating priority conservation areas requires considering specific mapping points and then undertaking field visits ([34]). Our field verification and consultation with forest managers revealed that our high-priority conservation areas have a high probability of deforestation since they are surrounded by agricultural and livestock areas, as well as small patches of urban areas. In fact, areas with high conservation priority for carbon sequestration were located in forest clusters with mature trees, fragmentation by agricultural areas with corn crops, livestock areas, and urban areas, which are all indicators of high carbon sequestration and high deforestation risk. Areas identified for medium conservation priority were typically pine-oak forest, with small patches of farmland within the forest area. Finally, low-priority areas showed little fragmentation by any land use. These areas were generally located away from the agricultural frontiers, or were located in managed forest areas.
Conservation strategies for priority areas
The identification and management of priority conservation areas must consider economic, social and environmental factors to ensure sustainability ([46]). Tab. S3 (Supplementary material) outlines some key management strategies and responsible implementing agencies for the study area. The development of management strategies at different administrative and geographic levels has been considered to foster efficiency in conservation programs and actions, seeking to obtain maximum impact with the resources available in the study area ([32], [35]).
Future studies should focus on a thorough quantification of both above- and below-ground carbon components in order to better determine total carbon sequestration and how these levels are affected by various forest management regimes. To better understand deforestation risk, we suggest the development of a series of future scenarios of land use change. These could serve as the basis for analyzing the impacts and opportunity costs of carbon sequestration and other ecosystem services. For priority carbon areas, we recommend to test various forest management practices designed to both protect stored forest carbon and facilitate future carbon sequestration at the forest level. These practices should carefully consider both conservation and management goals, seeking to provide a blueprint for mitigating climate change impacts on Mexico’s forest resources.
Conclusions
We estimated above-ground carbon sequestration in forests of Chignahuapan and Zacatlan municipalities, Puebla (Mexico) using volume information from forest management programs and the kriging interpolation method. Our results showed that both municipalities have similar current carbon sequestration, with carbon estimates ranging from 14 to 531 Mg CO2 ha-1. Based on this, we classified areas as high current carbon sequestration (218 to 531 Mg CO2 ha-1), medium (96 to 218 Mg CO2 ha-1), and low sequestration (from 0 to 96 Mg CO2 ha-1). Next, we applied a probabilistic model of land use in order to identify future risk of deforestation based on a host of socioeconomic and biophysical variables. Combining current carbon sequestration with deforestation risk allowed to identify priority lands for conservation. In Chignahuapan and Zacatlan, 628 and 310 ha, respectively, were determined to have high priority for carbon sequestration.
It is important to note that in this study the potential of forests to sequester additional carbon in the future was not considered. For example, a young forest on a productive site may have little stored carbon today, but may sequester large quantities in the future. In contrast, an older stand comprised of many large trees may have high levels of stored carbon, but may not store much additional carbon in the future as stand growth slows. In fact, age -or density- induced mortality could transition the stand into a net carbon emitter as large trees die and decompose.
Despite this, the approach developed here represents an important first-step in identifying priority conservation areas through the integrated assessment of carbon sequestration and deforestation risk. This knowledge can enhance efforts to efficiently target land management strategies aimed to mitigate climate change impacts. In doing so, the approach can serve as a model for other forested regions in Mexico and other countries.
Acknowledgments
We thank the Colegio de Postgraduados for funding this study under Priority Research Line 1 (LPI1): Sustainable Management of Natural Resources. We also thank Dr. Robert J. Lilieholm and Dr. H.M. de los Santos-Posadas for technical review and statistical support.
References
Gscholar
Online | Gscholar
Gscholar
Gscholar
Gscholar
Gscholar
Gscholar
Gscholar
Gscholar
Gscholar
Gscholar
Gscholar
Gscholar
Gscholar
Gscholar
Gscholar
Gscholar
Gscholar
Gscholar
Gscholar
Gscholar
Gscholar
Gscholar
Gscholar
Gscholar
Gscholar
Gscholar
Authors’ Info
Authors’ Affiliation
Manuel De Jesús González-Guillén
Tomás Martínez-Trinidad
Miguel Escalona-Maurice
Colegio de Postgraduados, Carretera México-Texcoco Km. 36.5, Montecillo, Edo. de México, C.P. 56230 (Mexico)
Corresponding author
Paper Info
Citation
Cruz-Huerta C, González-Guillén MDJ, Martínez-Trinidad T, Escalona-Maurice M (2017). Identifying priority conservation areas for above-ground carbon sequestration in Central Mexico. iForest 10: 923-929. - doi: 10.3832/ifor1980-010
Academic Editor
Francesco Ripullone
Paper history
Received: Jan 12, 2016
Accepted: Sep 08, 2017
First online: Dec 07, 2017
Publication Date: Dec 31, 2017
Publication Time: 3.00 months
Copyright Information
© SISEF - The Italian Society of Silviculture and Forest Ecology 2017
Open Access
This article is distributed under the terms of the Creative Commons Attribution-Non Commercial 4.0 International (https://creativecommons.org/licenses/by-nc/4.0/), which permits unrestricted use, distribution, and reproduction in any medium, provided you give appropriate credit to the original author(s) and the source, provide a link to the Creative Commons license, and indicate if changes were made.
Web Metrics
Breakdown by View Type
Article Usage
Total Article Views: 40569
(from publication date up to now)
Breakdown by View Type
HTML Page Views: 34609
Abstract Page Views: 2056
PDF Downloads: 3201
Citation/Reference Downloads: 18
XML Downloads: 685
Web Metrics
Days since publication: 2424
Overall contacts: 40569
Avg. contacts per week: 117.15
Article Citations
Article citations are based on data periodically collected from the Clarivate Web of Science web site
(last update: Feb 2023)
Total number of cites (since 2017): 3
Average cites per year: 0.43
Publication Metrics
by Dimensions ©
Articles citing this article
List of the papers citing this article based on CrossRef Cited-by.
Related Contents
iForest Similar Articles
Review Papers
A review of the environmental Kuznets curve hypothesis for deforestation policy in Bangladesh
vol. 4, pp. 16-24 (online: 27 January 2011)
Research Articles
Approaches to classifying and restoring degraded tropical forests for the anticipated REDD+ climate change mitigation mechanism
vol. 4, pp. 1-6 (online: 27 January 2011)
Research Articles
An assessment of climate change impacts on the tropical forests of Central America using the Holdridge Life Zone (HLZ) land classification system
vol. 6, pp. 183-189 (online: 08 May 2013)
Commentaries & Perspectives
What happened to forests in Copenhagen?
vol. 3, pp. 30-32 (online: 02 March 2010)
Technical Reports
Deforestation, land conversion and illegal logging in Bangladesh: the case of the Sal (Shorea robusta) forests
vol. 5, pp. 171-178 (online: 25 June 2012)
Research Articles
Do the rubber plantations in tropical China act as large carbon sinks?
vol. 7, pp. 42-47 (online: 21 October 2013)
Research Articles
Environmental Kuznets curve for deforestation: evidence using GMM estimation for OECD and non-OECD regions
vol. 10, pp. 196-203 (online: 13 December 2016)
Research Articles
An approach to estimate carbon stocks change in forest carbon pools under the UNFCCC: the Italian case
vol. 1, pp. 86-95 (online: 20 May 2008)
Technical Reports
The treatment of land use, land use change and forestry in the post-2012 climate agreement: a perspective from non-Annex I Parties
vol. 3, pp. 56-58 (online: 17 May 2010)
Editorials
Change is in the air: future challenges for applied forest research
vol. 2, pp. 56-58 (online: 18 March 2009)
iForest Database Search
Google Scholar Search
Citing Articles
Search By Author
Search By Keywords
PubMed Search
Search By Author
Search By Keyword