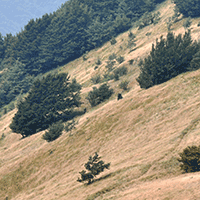
Effects of traditional coppice practices and microsite conditions on tree health in a European beech forest at its southernmost range
iForest - Biogeosciences and Forestry, Volume 9, Issue 4, Pages 673-681 (2016)
doi: https://doi.org/10.3832/ifor1603-008
Published: Mar 12, 2016 - Copyright © 2016 SISEF
Research Articles
Abstract
European beech (Fagus sylvatica) grows at the southern limit of its range in the mountain-Mediterranean vegetation belt up to the timberline. The southernmost beech forests of Sicily (southern Italy) show peculiar ecological, structural and silvicultural characteristics, growing in fragmented and isolated stands near the timberline and in topographically marginal unfavorable habitats. Past silvicultural practices increased the heterogeneity of stand structure at these sites. We compared stand structural characteristics and tree health in coppice-cut and control beech stands with respect to the local topographic gradient (bottom, slope and ridge) and canopy cover (clearing/border vs. interior trees). Our results clearly showed a correlation between declining tree health (crown and bark damage, higher percentage of dead trees and lower seedling density) and recent coppice-cuts, poor (marginal) site quality (on ridges and slopes) and reduced canopy cover (in clearing/border trees). The decrease of tree health indicate an increasing threat to the long-term viability of beech stands facing multiple environmental stress factors (such as those related to southern latitude and topographic position). Declining tree health in the control plots also supports this hypothesis. We concluded that traditional forest management practices, such as coppice-cuts applied regardless to the specific microenvironmental conditions, may pose a risk to beech forest health at the southernmost edge of the species’ range.
Keywords
Marginal Beech Sites, Site-specific Ecology, Topographic Gradient, Cover Fragmentation, Silviculture, Coppice-cuts, Tree Damage, Madonie Mts, Sicily
Introduction
Common beech (Fagus sylvatica L.) grows in Europe from the northern and temperate-Atlantic lowlands to the southern Mediterranean mountains, where it reaches the timberline or mountain tops ([3]). In Italy, beech forests represent about 12% of the high-elevation forest cover, ranging from the Alps to the species’ southern limit in Sicily. According to the National Forest Inventory ([32]), beech forests cover 1 042 129 ha in Italy, over 9% of the country’s total forest area. Beech stands are found in a wide range of environmental settings, from highly humid sites in the Alpine valleys to the dry mountaintops of Sicily. This broad distribution suggests the existence of local provenances and specific adaptations ([39], [54]).
Previous studies on climate change ([59], [26]) suggested that the survival of beech stands is significantly threatened at the southernmost limits of the species’ range ([8], [2], [5]). Kramer et al. ([36]) projected that both southern and northern limits of European beech will shift northward. In addition, climate change may deeply affect natural regeneration processes ([26], [57]), growth and productivity ([17], [22], [33], [58], [1]), and physiological performance ([23], [52], [27]).
In the Mediterranean region mean temperatures are currently increasing, rainy seasons are becoming shorter and drought periods are longer and more frequent; a shift upward of the treeline and a shrinkage of the species’ distribution in southern Europe is anticipated ([44], [33]). The Sicilian beech stands growing on the northern coastal mountaintops are expected to experience increasingly severe drought and warming, leading to decline, population decreases and potential local extinction of the species ([49], [47]).
In recent years, severe drought has affected beech stands at the southern sites, which calls for an active management of stands. Past silvicultural practices have largely influenced stand structure variability of beech forests in all Mediterranean mountains, by creating and maintaining a wide spectrum of structural forms ([6]). Coppicing to supply wood for charcoal in local markets has been the major factor affecting forest structure ([19], [16], [42], [55]). With a decreasing demand for charcoal production, the majority of the beech coppices were no longer managed, and many are currently being converted to high forests ([7], [15], [42], [37]).
At the forest stand level, climate and management practices may interact with topography, cover and edge effects. Indeed, Mediterranean mountain beech stands located on ridges or steep slopes, as well as those that are in fragmented stands or near edges, are negatively affected by the increased exposure to wind, turbulence, warmer air and higher soil temperatures ([28], [29], [4]).
This study focuses on the structural characteristics of coppice beech stands growing on the Madonie Mountains (the northern mountain chain of Sicily) in relation to the local topographic gradient and the forest cover fragmentation, with the following goals: (i) to verify whether traditional coppice practices are still suitable for sustainable management of beech at its southernmost limit; (ii) to identify silvicultural practices for maintaining beech stands in these marginal areas. To evaluate the effect of coppicing on stand structure and tree health, structural characteristics, seedling density, defoliation index, bark damage and the percentage of dead trees were compared between recently coppiced and non-cut (control) forest plots.
Material and methods
Study area
The Sicilian beech forests cover 15 924 ha ([12]) and are of particular phytogeographic and ecological interest ([25], [51], [11]). Beech grows from 1200 up to 2000 m a.s.l. on the ridges and high slopes of the Nebrodi and Madonie mountains (N and NE Sicily - Fig. 1), as well as on the slopes of Mont Etna ([21]). Annual precipitation in the area usually exceeds 1000 mm, barely fulfilling the water needs of the species, suggesting the existence of locally adapted populations ([41]). The typical Mediterranean summer drought is partly mitigated on northern slopes (facing the sea) by humid winds. The mean annual temperature ranges from 7.0 to 11.0 °C; the Rivas-Martinez bioclimatic classification is humid supra-Mediterranean.
Fig. 1 - (a) Distribution of Fagus sylvatica in Europe (after EUFORGEN project map); (b) beech forests in Sicily and (c) in the study area (Madonie mountains). Data from the Forest Map of Sicily - Regional Forest Information System 2011). Sample sites are indicated by black circles in the panel (c).
Beech stands in this area grow on quartzarenite (Numidian Flysch) substrate. Soil types include Eutric Cambisols (brown soils), Eutric Regosols and Lithosols, varying with the topography (ridges, steep slopes or summit plateaus). At higher altitudes beech cover is highly fragmented and trees are often shrub-like, with multiple stems and reduced height. Both deciduous and evergreen oaks (Quercus petraea, Q. ilex, Q. pubescens) can be found in beech stands, mainly at lower elevations (1200-1300 m a.s.l.).
Traditionally, beech forests in Sicily were mostly managed as coppice for charcoal production ([30]). All beech forests are still used for occasional grazing and wood harvesting, resulting in highly diversified structures which cannot be easily classified as standard structural managing models (simple coppice, coppice with standards or selection coppice). Wildfires are rare in the higher vegetation belt, including in beech forests.
Data collection
Six study sites (A, B, C, D, E and F) were selected in the upper part of the Madonie mountains after a review of the local literature on beech forests ([30], [12], [20]) based on the following criteria: (i) coppice stands must have been recently (last one or two decades) managed using local, traditional silvicultural methods; (ii) at least part of the stands must not have been managed in the last three or four decades (to be used as control plots).
A field survey was conducted at each site on 2 paired plots (i: recently coppiced; ii: control), totaling 12 sample plots (Fig. 2, Tab. 1). Each plot was circular, with a radius of 20 m. Plots were located in pure beech stands along an elevation (1600-1800 m a.s.l.) and topographic gradient (bottom, slope, ridge - Fig. 3). Paired plots allowed the comparison of stand characteristics (structural variables, seedling density, tree defoliation index, bark damage, and the percentage of dead trees) between recently coppices stands and controls growing under similar ecological conditions (Fig. 2, Fig. 3). Altitude, topographic position, aspect and slope of each plot were recorded, and its center georeferenced by GPS. The position of all trees in the sample area was determined by polar coordinates (distance from plot center and azimuth). To determine the maximum age of shoots in each plot, core samples were collected from the base of the six largest stems in the plot using an increment borer.
Fig. 2 - Location of the paired plots at each sample site (A, B, C, D, E, F) and location of the sample transects. (L): length of each transect in meters.
Tab. 1 - Physiographic characteristics of the study sites. The label “cut” indicates recently coppice-cut plots, while control plots are labelled as “control”. Geographic coordinates are given for the center of the two paired plots sampled at each site. Altitude, aspect, slope and stand density are given as the mean of the two plots.
Site name | Label | Latitude Longitude |
Topography | Altitude (m a.s.l.) |
Aspect | Slope (%) |
Stand density (n ha-1 ± SE) |
---|---|---|---|---|---|---|---|
Vallone Prato | A (cut) | 37° 50′ 52″.77 N 14° 02′ 14″.60 E |
bottom | 1640 | SE | 30-40 | 2769 ± 280 |
Piano Iola | B(control) | 37° 50′ 50″.14 N 14° 02′ 10″.43 E |
bottom | 1630 | S | 10 20 | 4687 ± 368 |
Piano Iola-Piano Grande | C(cut) | 37° 50′ 45″.54 N 14° 02′ 07″.63 E |
slope | 1720 | NO | 30-40 | 2984 ± 301 |
Piano Grande | D(cut) | 37° 50′ 32″.59 N 14° 02′ 06″.64 E |
ridge | 1770 | - | 0-5 | 3287 ± 316 |
Piano Grande | E(control) | 37° 50′ 29″.08 N 14° 02′ 14″.75 E |
ridge | 1760 | - | 0-5 | 14705 ± 613 |
Piano Iola-Piano Grande | F(control) | 37° 50′ 45″.43 N 14° 01′ 58″.92 E |
slope | 1700 | NO | 30-40 | 5411 ± 357 |
Fig. 3 - Location of the paired plots and the transects along the topographic and elevation gradients.
Diameter at breast height (DBH) of all living trees (all shoots >3 cm on each stool) and tree height (H) were measured. Additionally, the following structural characteristics were recorded in each sample plot: height of crown insertion (m), crown radius (mean of the radii taken at the four cardinal points), canopy cover (%), stool number and location, coppice-shoot number per stool.
The presence and location of beech regeneration was determined by recording all plants with diameter (D) < 3 cm (at ground level) and height (H) < 1 m along two transects in each sample plot; the transects were 40×2 m, oriented according along the cardinal directions. Seedling production (seedlings ha-1) was determined by counting all recruitments of the current year along the transects, while recruitments of the previous years were classified as saplings (saplings ha-1). Seedling and sapling were defined according to Borghetti & Giannini ([10]), Papalexandris & Milios ([43]) and Barbeta et al. ([4]).
Based on the above measurements, the following structural parameters were calculated for each sample plot: stem density (shoots ha-1) and stool density (stools ha-1); mean tree DBH (cm) and mean tree height (m); basal area (G, m2 ha-1); frequency distribution of trees with respect to DBH (2.5 cm classes) and H (5 m classes). The number of individuals in each size class for DBH and H was calculated based on the stand density of each plot (number of individuals per hectare). The total basal area (G, m2) from all the shoots for each individual (stool) was also calculated. The whole shoot volume (V, m3) was obtained using the mathematical models developed by the Italian National Forest Inventory ([38]) and the Sicilian Regional Forest inventory ([31]).
Tree health data collection
Damage was assessed for all beech trees in each sample plots. Crown defoliation and bark damage were estimated visually and defined as the percentage of crown leaf loss and the percentage of damaged stem bark (necrosis; cracks), respectively. Stem bark damage are to be considered as the effect of direct sunlight on the bark after the canopy opening by cutting. To assess crown damage, the shoot crown on each stool was split into 9 (3×3) quadrants (Fig. 4), and each quadrants assigned to a damage and defoliation class (based on 5% percentage of increment). Trees were observed systematically from the south side. Dead trees were recorded, and the ratio of dead to total individuals was calculated for each plot.
Fig. 4 - Schematic representation of the shoot crown on a stool, divided into 9 (3x3) quadrants in order to assess defoliation and stem damage in the different parts of the plant.
In addition to sampled plots, tree health data collection was extended to a total of three transects located along the topographic and altitudinal gradient (Fig. 2, Fig. 3). Each transect was 5 m large and 350-450 m long, and included bottom, slope and ridge segments. The same procedure of damage assessment adopted for plots was also used for the transects.
Data analysis
The non-parametric Kolmogorov-Smirnov (KS) test was used to test for differences in the diameter distribution of stems or shoots (by size class) between coppice-cut and control plots. The Student’s t-test was applied to compare structural variables (plant and stool density, no. of shoots per stool, mean tree DBH, H, G and V), regeneration density (seedlings and saplings), and damaged and dead trees between coppiced and control plots.
Differences in the measured variables among silvicultural treatments (coppice-cut plots vs. non-cut plots/controls), topography (bottom, slope and ridge plots), and canopy cover (clearing/edge trees vs. interior trees) were tested by ANOVA. Mean comparison was carried out using the Tukey’s post-hoc test (α = 0.05).
All statistical analyses were carried out using the software package STATISTICA® version 8 (StatSoft Inc., Tulsa, OK, USA).
Results
Structur characters
The analysis of core samples extracted from the larger stools in the sample plots revealed that all the studied stands have been subject to previous coppice cuts 43 and 54 years ago. Only a single stem (shoot) of age of 63 and one of age 76 years were recorded in plots E and D, respectively.
A total of 4714 stems/shoots with DBH > 3 cm were measured in the 12 sample plots. The mean values (± SE) of all measured and derived stand variables are reported in Tab. 3, for both coppice-cut plots (A, C, D) and control plots (B, E, F - Tab. 2).
Tab. 3 - Stand parameter values after cut (mean ± SE) for each sample site (two plots of each site).
Parameter | Site (mean ± SE) | |||||
---|---|---|---|---|---|---|
A(cut) | B(control) | C(cut) | D(cut) | E(control) | F(control) | |
Stand density (n ha-1) | 2769 ± 280 | 4687 ± 368 | 2984 ± 301 | 3287 ± 316 | 14705 ± 613 | 5411 ± 357 |
Stool density (n ha-1) | 637 ± 37 | 1098 ± 59 | 851 ±57 | 461 ± 41 | 509 ± 29 | 1146 ± 63 |
N.shoots/stool | 4 ± 0.26 | 4 ± 0.22 | 3 ± 0.27 | 7 ± 0.71 | 29 ± 3.23 | 5 ± 0.46 |
DBH (cm) | 10.3 | 9.2 | 6.7 | 7.8 | 6.5 | 9.2 |
H (m) | 9.3 | 9.5 | 6.7 | 5.5 | 6.3 | 9.2 |
G (m2 ha-1) | 23.1 | 31.4 | 10.5 | 15.8 | 48.4 | 36.3 |
V (m3 ha-1) | 100.1 | 133.5 | 33.3 | 38.5 | 146.6 | 163.5 |
Seedlings density (n ha-1) | 637 ± 226 | 485 ± 174 | 573 ± 278 | 294 ± 154 | 350 ± 88 | 605 ± 112 |
Saplings density (n ha-1) | 31.8 ± 12.8 | 31.8 ± 11.4 | 15.9 ± 10.9 | 23.9 ± 12.8 | 31.8 ± 9.1 | 31.8 ± 10.1 |
Defoliation index (% individual) | 14.0 ± 2.3 | 12.4 ± 1.4 | 21.6 ± 5.7 | 27.3 ± 9.7 | 17.5 ± 1.5 | 17.1 ± 4.7 |
Bark damage index (% individual) | 0.0 | 0.0 | 11.7 ± 1.9 | 7.1 ± 2.9 | 0.0 | 0.1 ± 0.04 |
trees (n ha-1) | 0.0 | 0.0 | 127.3 ± 41.3 | 0.0 | 15.9 ± 5.7 | 0.0 |
Tab. 2 - Structural characteristics of the sample plots (mean values) before and after the cutting treatments. The percentage of variation of density, basal area (G), and volume (V) after the cutting is reported. (*): include the natural mortality in control plots.
Plot | Year of cut | Density (n ha-1) | G (m2 ha-1) | V (m3 ha-1) | ||||||
---|---|---|---|---|---|---|---|---|---|---|
Before | After* | % | Before | After* | % | Before | After* | % | ||
A(cut) | 2011 | 6891 | 4122 | 60 | 30 | 7 | 22 | 121 | 21 | 17 |
B(control) | 2000 | 4862 | 175 | 4 | 32 | 1 | 2 | 136 | 3 | 2 |
C(cut) | 1997 | 5507 | 2523 | 46 | 18 | 8 | 42 | 57 | 24 | 42 |
F(control) | > 30 years ago | 5634 | 223 | 4 | 37 | 1 | 2 | 166 | 2 | 1 |
D(cut) | 1998 | 9868 | 6525 | 66 | 25 | 11 | 43 | 53 | 20 | 37 |
E(control) | > 30 years ago | 15308 | 603 | 4 | 49 | 1 | 2 | 149 | 3 | 2 |
Beech stands in coppice-cut plots were significantly less dense than control plots (3013 ± 232 vs. 8268 ± 2040 n ha-1 - Student’s t = -2.57; p < 0.05; df = 10); the number of shoots per stool was also significantly smaller (5.3 ± 0.23 vs. 6.6 ± 0.48 n ha-1 in coppice-cut and control plots, respectively - Student’s t = -2.39; p < 0.05; df = 800 - Fig. 5). Contrastingly, the stool density was similar in both stand types (Student’s t = -1.81; p = 0.09, df= 10).
Fig. 5 - Mean number of shoots per stool, basal area, regeneration (sapling) density, defoliation index, bark damage index, and percentage of dead trees for coppice-cut and control plots. Bars represent the standard error. (*): p < 0.05; (**): p < 0.01.
Coppice-cut stands had significantly lower values of both basal area (Student’s t = -6.18; p < 0.01; df = 10) and volume (Student’s t = -5.63; p < 0.001; df = 10 - Fig. 5, Tab. 3). The diameter distribution of coppice-cut and control stands showed a similar shape, but were significantly different (KS test, p = 0.029). In general, the number of individuals (stems) decreased from smaller to larger size classes. Student’s t-test applied to each diameter class revealed significant differences for the intermediate classes (DBH 10, 12, 14 cm - Fig. 6a). Similarly, the cumulative distribution of volume per individual (summing all the stems of each individual) was significantly different between coppice-cut and control plots (KS test, p = 0.001 - Fig. 6b), with significant differences in the intermediate volume classes (V 20, 40, 60, 80).
Fig. 6 - (a) Diameter distribution of living stems (shoots) per hectare in the coppice-cut and control plots. (b) Distribution of cumulative volume per individual, obtained as the sum of all shoots for each individual. Seedlings and saplings were not included. Bars represent the standard error. (*): p < 0.05; (**): p < 0.01.
Regeneration
The number of seedlings and saplings was not significantly different in coppice-cut and control stands (Fig. 5). Significant differences in regeneration were found among topographic positions after ANOVA (Fig. 7). Pooling data from coppice-cut and control plots together, regeneration was significantly lower on ridges as compared with other topographic locations (seedlings: p < 0.01; saplings: p < 0.05 - Fig. 7a). The number of seedlings showed a decrease from the lower to the higher elevation plots, while the number of saplings increased with the topographic gradient. In coppice-cut stands (Fig. 7b), the lowest regeneration level occurred on the ridges (p < 0.01), while the number of saplings decreased in the opposite direction from ridges down to the bottomlands. Contrastingly, seedling number was the highest in plots located on slopes (p < 0.05).
Fig. 7 - Mean and standard error of beech regeneration, distinguished as seedling and sapling, by topographic position of the plot analyzed. (a) All plots; (b) coppice-cut plots. (*): p < 0.05; (**): p < 0.01.
Health status and dead plants
Generally, crown defoliation was greatest in the upper and outside portions of the crown; less defoliation was observed in the lower and inside portions. By contrast, the greatest bark damage was observed in the lower part of each stem, mainly on the southern warmer exposures, and in plants bordering clearings and open areas.
Comparisons of coppice-cut and control plots revealed statistically significant differences in all the indicators of beech health (defoliation, bark damage, dead tree). The canopy defoliation index was twice as high in coppice-cut plots compared with controls (20.77% ± 1.02 vs. 9.03% ± 0.48 - Student’s t = 10.51; p < 0.01; df= 472 - Fig. 5). The bark damage index, which was negligible in control plots (0.15% ± 0.06), was relatively high in the cut stands (6.35% ± 0.56 - Student’s t = 12.24; p < 0.01; df= 472). As a consequence, a possible drought effect could be excluded. Moreover, the percentage of dead trees within sample plots was also higher in coppice-cut (6.97% ± 2.62) as compared with control areas (0.17% ± 0.41 - Student’s t = -2.49; p < 0.05; df= 10 - Fig. 5).
The analysis of the health status of trees with respect to the topographic gradient revealed that the defoliation index and bark damage index were higher on ridges and slopes than on bottom sites. Additionally, trees bordering clearings were less healthy than trees in stand interiors (Fig. 8). Indeed, beech trees in coppice-cut plots located on ridges and in clearings showed the highest defoliation index (up to 34.5% ± 4.4 - the highest value was in plot D with 41% defoliation), which was significantly higher than those found for in slope and bottom positions (Tukey’s post-hoc test, p < 0.01 - Fig. 8a). Beech trees in control plots with the same topographic position and canopy cover showed similar results, although with lower values, e.g., the defoliation index was 14.8% ± 1.6 for trees in the clearings on ridges (Fig. 8b).
Fig. 8 - Mean and standard error of health status indicators by topographic position of the plot analyzed. (a) Defoliation index of coppice-cut plots; (b) defoliation index of control plots; (c) bark damage index of coppice-cut plots. Significant differences among topographic positions after Tukey’s post-hoc test are indicated (**: p < 0.01).
The bark damage index is reported only for coppice-cut plots (Fig. 8c); in control plots, the index was close to 0%. The bark damage index was significant higher in plots on slopes for both edge and interior trees (Tukey’s post-hoc test, p < 0.01 - Fig. 8c).
In coppice-cut plots, dead beech trees were significantly more abundant on slopes and ridges (Tab. 3).
Discussion and conclusions
Southern European beech forests mainly grow in the mountain-Mediterranean vegetation belt of northeastern Spain, southern Italy and Sicily, and central Greece ([48], [45], [61]). Beech in Sicily grow at the very edge of the species’ European range ([21]). Beech stands on the Madonie mountains (about 2500 ha), as well as those located on the eastern slopes of Mount Etna (about 2000 ha), are highly fragmented as compared with the wider beech forests of the Nebrodi mountains (approximately 10 000 ha - Fig. 1b). Moreover, the latter stands are characterized by higher biomass and more growing stock as a result of deeper soils and more humid environment ([31]), while the former stands grow on less-developed shallow soils, and are mainly composed of multi-stemmed trees (several shoots on each stool), due to both environmental conditions and past coppicing.
Our results confirm that vegetative regeneration (sprouting) largely predominates in high mountains and in topographically marginal sites, which is a common reproductive strategy for beech growing under unfavorable environmental conditions ([46]), especially in the Mediterranean region ([45], [43], [9], [21]).
Past silvicultural practices carried out in the analyzed stands have fostered irregular stand structures and increased structural variability, reinforcing the importance of the adoption of a correct coppicing management ([15], [16], [19], [42]). Coppice with standards was the most common treatment of beech stands in Sicily, including the Madonie mountains, until the first half of the last century ([30]). Their subsequent abandonment or the irregular (less frequent) utilization have contributed to the current atypical stand structures and dynamics.
Our results revealed a significant impact of silvicultural practices on the studied beech forests. Felling practices carried out in the last two decades (Tab. 2), uniformly applied to a coppice-like stand structure without consideration of site-specific environmental conditions (i.e., topography and soil), negatively influenced stand development. Ciancio & Nocentini ([14]) emphasized that coppicing in harsh climates and environmental conditions produces many crooked and branched stems.
The comparison of coppice-cut and control plots indicated a high utilization rate, i.e., 20-40% of plant basal area (G) and volume (V) and up to 66% of stem (shoots) density (Tab. 2). The higher G and V observed in the control plots as compared with coppice-cut plots (Tab. 3) reflect the different architecture of individual trees in the two stand types, because stools in control plots tend to have more stems (shoots) per individual than stools in coppiced plots (Fig. 5, Fig. 6).
The analysis of regeneration did not reveal significant effects of the silvicultural treatment on seedlings and saplings density. However, seedlings tend to be less abundant in coppice-cut plots compared with controls, while the opposite was true for saplings.
Climate, topography, soil and stand structure are the main factors controlling seedling density at the microsite level ([57]). In this study, we found a considerably lower number of seedlings in plots located on ridges and mountaintops as compared with those in the bottomlands, with the largest reduction of recruitments in the coppice-cut plots (Fig. 7). On the other hand, such reduction was not found for saplings. Similar results were reported by Barbeta et al. ([4]), who compared fragmented and continuous forests at the edge of the species’ range in southeastern Spain. However, the projected increasing temperatures in the Mediterranean region are likely to reduce the frequency of successful seedling establishment ([34], [50], [62]), possibly leading to a general decline and local extinction ([49]).
The observed decrease in seedling establishment and plant health are indicative of the poor site quality at the coppice-cut stands analyzed. Our results suggest a strong effect of silvicultural treatment on habitat quality: beech trees in coppiced plots were more than twice as defoliated and had 6 times more bark damage than those in control plots. Moreover, dead trees were 7 times more abundant in coppice-cut than in control plots (Fig. 5).
The defoliation index, the bark damage index, and the percentage of dead trees were strongly correlated with local topography (Fig. 8, Tab. 3). The defoliation index of coppiced plots on ridges was nearly double (35% mean defoliation) that that of coppiced plots in bottomlands (21% - Fig. 8a). The effect of topography was also evident in the control plots, with approximately 3-4 times more damage on ridges than in bottomlands. Similar results were also observed for other indicators of tree health, such as bark damage and frequency of dead trees. These findings support the hypothesis that the negative effect of inappropriate silvicultural practices could be strengthened by the worse environmental conditions expected under the current climate change.
In summary, our results suggest that the health of beech stands on the Madonie mountains declines in more marginal sites, at higher altitudes, and on steep slopes, ridges and summit plateaus, as a consequence of the combined effect of anthropogenic and ecological factors. In particular, the shallow soils (siliceous, rocky and skeletal) and the effects of strong winds, such as increased evapotranspiration and air turbulence ([28]) are among the most limiting environmental factors. Aridity and wind speeds are expected to be higher in these marginal sites, affecting tree health through drought and mechanical damage ([4]).
In addition, habitat quality in the studied sites is strongly affected by the lack of a continuous canopy cover ([29]). The negative effects of habitat fragmentation on tree health have been attributed to edge and area effects ([24], [28]). The effect of direct sun on trees bordering clearings is particularly evident in their health indicators (defoliation, bark damage, dead trees), especially for Mediterranean beech stands at high altitudes or at the southernmost edge of the species’ range.
Environmental stress can be strongly enhanced by the adoption of different management regimes or silvicultural practices ([35], [15], [62]). Extensive coppicing in the analyzed plots A, C and D increased environmental stress. Coppicing amplified the ecological effects of forest fragmentation and canopy gap formation, affecting growth patterns, regeneration processes, and sunlight effects ( [60], [18], [13], [53]).
To mitigate the negative effects of frequent coppice clearcutting on soils, landscape and biodiversity conservation, the conversion of coppices to high forests or to natural stand dynamics with continuous cover has recently become an increasingly common management goal, especially in hilly and mountainous Mediterranean regions ([56], [15], [42], [40]). Anyway, in harsh habitats (as in the case study) where multi-stemmed shrub-like growth forms are common and conversion is not feasible, it is compulsory to maintain a high canopy cover and a large stem density. Therefore, management in such ecological context should aim at reducing the fragmentation of beech stands and increasing canopy cover by plantation.
In summary, the results of this study highlight the various ecological and management effects that influence habitat quality in outlying beech stands of the Madonie mountains. Here, beech has to be considered as a “pioneer” species surviving in extreme habitats, characterized by severe summer drought, shallow soils, strong winds, scattered canopy cover and unsuitable management practices, such as occasional wood harvesting and uncontrolled grazing. In this context, traditional silvicultural practices used in the past are no longer suitable and quite detrimental to the stands’ survival. A cautious management approach should be adopted, avoiding felling practices and other anthropogenic disturbances in the most marginal topographic sites such as ridges, summit plateaus and steep slopes.
References
Gscholar
Gscholar
Gscholar
CrossRef | Gscholar
CrossRef | Gscholar
Gscholar
CrossRef | Gscholar
Gscholar
Gscholar
Gscholar
Gscholar
CrossRef | Gscholar
CrossRef | Gscholar
Gscholar
CrossRef | Gscholar
CrossRef | Gscholar
CrossRef | Gscholar
CrossRef | Gscholar
Gscholar
Gscholar
CrossRef | Gscholar
Authors’ Info
Authors’ Affiliation
Gaetano La Placa
Federico G Maetzke
Dipartimento SAF - Scienze Agrarie e Forestali, Università degli Studi di Palermo, Viale delle Scienze, Edificio 4, Ingresso H, 90128 Palermo (Italy)
Corresponding author
Paper Info
Citation
Cullotta S, La Placa G, Maetzke FG (2016). Effects of traditional coppice practices and microsite conditions on tree health in a European beech forest at its southernmost range. iForest 9: 673-681. - doi: 10.3832/ifor1603-008
Academic Editor
Renzo Motta
Paper history
Received: Feb 16, 2015
Accepted: Nov 12, 2015
First online: Mar 12, 2016
Publication Date: Aug 09, 2016
Publication Time: 4.03 months
Copyright Information
© SISEF - The Italian Society of Silviculture and Forest Ecology 2016
Open Access
This article is distributed under the terms of the Creative Commons Attribution-Non Commercial 4.0 International (https://creativecommons.org/licenses/by-nc/4.0/), which permits unrestricted use, distribution, and reproduction in any medium, provided you give appropriate credit to the original author(s) and the source, provide a link to the Creative Commons license, and indicate if changes were made.
Web Metrics
Breakdown by View Type
Article Usage
Total Article Views: 46311
(from publication date up to now)
Breakdown by View Type
HTML Page Views: 40055
Abstract Page Views: 2089
PDF Downloads: 3076
Citation/Reference Downloads: 23
XML Downloads: 1068
Web Metrics
Days since publication: 3176
Overall contacts: 46311
Avg. contacts per week: 102.07
Article Citations
Article citations are based on data periodically collected from the Clarivate Web of Science web site
(last update: Feb 2023)
Total number of cites (since 2016): 6
Average cites per year: 0.75
Publication Metrics
by Dimensions ©
Articles citing this article
List of the papers citing this article based on CrossRef Cited-by.
Related Contents
iForest Similar Articles
Review Papers
Structure and management of beech (Fagus sylvatica L.) forests in Italy
vol. 2, pp. 105-113 (online: 10 June 2009)
Review Papers
Opportunities for coppice management at the landscape level: the Italian experience
vol. 9, pp. 775-782 (online: 04 August 2016)
Research Articles
Tree-oriented silviculture: a new approach for coppice stands
vol. 9, pp. 791-800 (online: 04 August 2016)
Review Papers
Should the silviculture of Aleppo pine (Pinus halepensis Mill.) stands in northern Africa be oriented towards wood or seed and cone production? Diagnosis and current potentiality
vol. 12, pp. 297-305 (online: 27 May 2019)
Research Articles
Optimizing silviculture in mixed uneven-aged forests to increase the recruitment of browse-sensitive tree species without intervening in ungulate population
vol. 11, pp. 227-236 (online: 12 March 2018)
Research Articles
Effects of traditional forest management on carbon storage in a Mediterranean holm oak (Quercus ilex L.) coppice
vol. 11, pp. 344-351 (online: 18 April 2018)
Research Articles
The conversion into high forest of Turkey oak coppice stands: methods, silviculture and perspectives
vol. 13, pp. 309-317 (online: 10 July 2020)
Research Articles
Small forest parcels, management diversity and valuable coppice habitats: an 18th century political compromise in the Osnabrück region (NW Germany) and its long-lasting legacy
vol. 9, pp. 518-528 (online: 17 March 2016)
Research Articles
Predicted occurrence of ancient coppice woodlands in the Czech Republic
vol. 10, pp. 788-795 (online: 16 September 2017)
Research Articles
Integrating conservation objectives into forest management: coppice management and forest habitats in Natura 2000 sites
vol. 9, pp. 560-568 (online: 12 May 2016)
iForest Database Search
Search By Author
Search By Keyword
Google Scholar Search
Citing Articles
Search By Author
Search By Keywords
PubMed Search
Search By Author
Search By Keyword