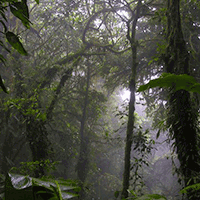
Can traditional selective logging secure tree regeneration in cloud forest?
iForest - Biogeosciences and Forestry, Volume 10, Issue 2, Pages 369-375 (2017)
doi: https://doi.org/10.3832/ifor1937-009
Published: Mar 07, 2017 - Copyright © 2017 SISEF
Research Articles
Abstract
Unplanned selective logging for charcoal and firewood is a common practice in tropical montane cloud forest (TMCF), a high priority ecosystem for biodiversity conservation at the global scale. However, limited information is available regarding the impact of such logging on forest regeneration. We evaluated the abundance and composition of tree regeneration in four TMCF sites subject to traditional selective logging in southern Mexico. At each site, we calculated a tree extraction index based on the number of stumps, logs and charcoal kilns and established six 200 m2 plots where the abundance of adult, sapling and seedling trees were recorded and canopy cover estimated. Based on the extraction index and estimated basal area values, two sites each were classified as being of low (L) and high (H) logging intensity; the extraction index was three times lower in L (7.5 and 9.2) than in H (35 and 35) sites, while basal area was significantly higher in L than in H sites (80.2 ± 10.2 vs. 41.9 ± 4.96 m2 ha-1, respectively). No significant differences were found among sites in terms of canopy cover, diameter and density of adult trees or in the density of saplings and seedlings (0.72 individuals m-2). In all sites, species of intermediate shade-tolerance dominated the regeneration (76%), followed by the shade-tolerant (23%) and pioneer (1%) species. Regeneration of Quercus spp. (four species) dominated at all sites (50.5%); this is a group of particular interest to the local communities because of its utility for firewood and charcoal. The similarity in composition between adult and regenerating tree species was relatively high in all of the sites (Morisita-Horn Index L1=0.86, L2=0.64, H1=0.69 and H2=0.71). These results indicate that, under the evaluated selective logging intensities, TMCF can sustain sufficient regeneration of Quercus spp. and thus presents an opportunity for sustainable management. The legacy effects of traditional selective logging on TMCF tree regeneration are discussed.
Keywords
Firewood, Forest Management, Mexico, Quercus, Seedlings, Timber Harvesting, Tropical Montane Cloud Forest, Disturbance
Introduction
Secondary and degraded forests currently form the dominant components in tropical landscapes, covering an area larger than that of primary forests ([4], [13]). The degree of contribution of degraded forests to biodiversity conservation depends on the type, intensity and frequency of disturbance to which these have been subjected ([16], [40]). A common cause of degradation in tropical forests worldwide is selective logging to obtain timber, firewood and charcoal ([5]). This unplanned practice consists of the isolated extraction of trees above a certain trunk diameter, and involves no measures to reduce the consequent negative impacts on diversity. Compared to other types of disturbance, such as the conversion of forest to agricultural and livestock production uses, selective logging has considerably less impact on the diversity of species in tropical forests ([16], [3]); however, the impacts of traditional selective logging on the regeneration of different tropical forests are poorly understood because of the particular nature of the characteristics and traditional uses in each ecosystem.
Chronic selective logging is an activity historically practiced in the tropical montane cloud forest (TMCF), one of the most threatened tropical ecosystems worldwide ([45]). This ecosystem has high conservation priority status because of its high alpha and beta diversity, as well as the range of environmental services it provides ([28], [45]). The TMCF are frequently located in inaccessible gullies and on steep slopes, with trees of variable commercial quality and low productivity ([45]) that present low potential for timber forestry ([48]). Nevertheless, these forests are exploited daily by local communities through unregulated traditional extraction, whose production is essentially of subsistence ([44]). Despite the wide distribution of forest management practices in Mexico, there are no records of TMCF under authorized forest management ([8]). In addition to other factors of change, selective logging threatens the persistence of populations of tree species that are exclusive to this ecosystem. It is estimated that more than 60% of TMCF tree species in Mexico are threatened and many of these species are affected by a decline in their natural regeneration ([19]).
Regeneration is a key process, since the establishment and survival of seedlings is crucial to the reproductive success and population dynamics of species. Among the main effects of selective logging is increased canopy openness, which affects germination and growth of established seedlings ([22], [30], [38]). Studies of the effect of selective logging on the diversity of tree regeneration in tropical forests under planned forestry management have produced contrasting results. While selective logging can negatively affect the diversity and regeneration of tropical and temperate forests ([12], [6]), opening of the canopy as a consequence of planned extraction can also have positive effects on the regeneration of certain timber species ([23], [20], [11]). In a recent meta-analysis of tropical forests, Martin et al. ([33]) found that tree richness increased with low logging intensities and vice versa. Most studies agree that selective logging has a significant influence on species composition ([10], [12]), while other studies report effects on functional guilds or ecological groups ([3], [26]).
Understanding the regeneration dynamics in response to tree extraction is therefore essential for effective TMCF management. However, there are very few studies that address selective logging in TMCF and its effects on the structure and composition of arboreal regeneration ([44]). The objectives of this study were therefore: (i) to evaluate the extent of extraction in TMCF fragments subjected to chronic selective logging; (ii) to determine the nature of its relationship with tree structure and composition, as well as its influence on tree regeneration.
Methods
Study area
The study was conducted in the sub-basin of the River Pixquiac, within the La Antigua basin, in Veracruz, Mexico. This is a region of high priority for the conservation of TMCF ([48]). The landscape is dominated by patches of secondary and degraded TMCF, livestock pastures and agricultural land ([37]). Total annual precipitation is 1650 mm and the annual average temperature is 14 °C ([49]). Selective extraction of trees in this region varies in intensity and frequency, but it is a widely distributed practice that follows no planned method. Trees ≥40 cm in diameter and species of high dendroenergetic value are selected for extraction. These mainly belong to the genus Quercus and are mainly used to produce firewood and charcoal ([24]). In order to produce charcoal, logs are cut within the forest and processed in rudimentary kilns constructed in the forest soil.
Site selection and degree of extraction
In order to evaluate the status of regeneration with selective logging, four TMCF sites were chosen with a history of frequent selective logging over recent decades, but with no other form of disturbance (e.g., grazing in the forest, fires, introduction of exotic species - Tab. 1). Since no undisturbed forests were present within the study area (due to selective logging or other perturbations, such as grazing and unpaved forest roads), it was not possible to select a control treatment as reference. In the studied forests, clandestine tree extraction continues to occur with no authorization from the landowners. Analysis of panchromatic aerial photographs (1975, 1995 and 2004 - 1:20.000 scale, INEGI) confirmed that the four study sites had maintained continuous forest cover over the preceding 40 years. Slope varied from 58 to 69% among the sites.
Tab. 1 - Cloud forest structural variables (mean ± standard error) and tree diversity in sites with low (L1 and L2) and high (H1 and H2) selective logging intensities in Veracruz, Mexico. Data pertaining to seedlings (0.20-1 m height), saplings (>1 m height and <5 cm DBH) and adult trees (> 10 cm DBH) are presented. (DBH): diameter at breast height; (H′): Shannon-Wiener index, calculated for adult trees. Different letters denote significant differences between logging levels after Tukey’s post hoc analysis (P < 0.05).
Parameter | Logging Level | |||
---|---|---|---|---|
L1 | L2 | H1 | H2 | |
Coordinates | 19° 31′ 36″ N 96° 59′ 30″ W |
19° 31′ 59″ N 97° 00′ 08″ W |
19° 30′ 58″ N 97° 00′ 27″ W |
19° 31′ 04″ N 97° 00′ 16″ W |
Altitude (m) | 1522 | 1630 | 1661 | 1651 |
Seedlings (no m-2) | 0.79 ± 0.26 | 1.17 ± 0.24 | 1.13 ± 0.36 | 0.79 ± 0.16 |
Seedling height (m) | 0.59 ± 0.06 | 0.46 ± 0.05 | 0.50 ± 0.05 | 0.39 ± 0.05 |
Saplings (no m-2) | 0.50 ± 3.76 | 0.39 ± 1.61 | 0.53 ± 1.19 | 0.43 ± 1.01 |
Sapling height (m) | 2.06 ± 0.13 | 2.23 ± 0.15 | 2.45 ± 0.14 | 2.22 ± 0.15 |
Adult trees (no ha-1) | 1158.33 ± 26.48 | 991.67 ± 16.30 | 1191.67 ± 15.28 | 1025.00 ± 12.31 |
Basal area (m2 ha-1) | 87.80 ± 16.1a | 72.50 ± 13.1a | 44.86 ± 8.59 b | 38.98 ± 5.57 b |
DBH (cm) | 37.49 ± 4.08 | 34.99 ± 3.89 | 28.92 ± 2.66 | 30.00 ± 2.68 |
Canopy height (m) | 25.02 ± 2.55 | 24.95 ± 2.00 | 21.30 ± 1.15 | 23.28 ± 0.51 |
Canopy cover (%) | 85.09 ± 1.49 | 88.43 ± 1.26 | 89.56 ± 0.74 | 85.92 ± 0.76 |
H′ | 2.69 | 2.86 | 3.11 | 2.85 |
An extraction index (modified from [42]) was developed in order to characterize each site. An area of ~1.2 ha within and around the vegetation measurement plots (described below) was examined, and the numbers of tree stumps, logs and charcoal kilns recorded. Tree stump diameter was measured at the base (10 cm above ground level).
Woody vegetation and canopy coverage
In each site, six plots of 10×20 m (200 m2) were established at least 15 m far from the edge of the forest fragment and were distributed equidistantly. In each plot, the diameter at breast height (DBH, 1.3 m above the ground) of all trees with DBH ≥ 10 cm was recorded, while the height of the five trees with the largest trunks was determined with a clinometer. A subplot of 5×5 m (25 m2) was randomly established in one of the corners of each 200 m2 plot in order to record all saplings >1 m in height and <10 cm DBH. The height and DBH values of all the individual saplings were recorded. In addition, four subplots of 1×1 m were established along the central axis of each 200 m2 plot (two at each side in an alternating pattern) in order to record tree seedlings > 0.2 m and ≤ 1 m in height. The 1 m2 subplots were located at a distance of 3 m from the extremes of the plot and were equidistantly distributed. Maximum seedling height and stem basal diameter were recorded. In order to evaluate the relationship between canopy coverage and regeneration, the canopy coverage in the center of each 1 m2 plot was measured with a convex densiometer.
Specimens were identified with the help of specialists and voucher specimens were deposited in the IE-XAL herbarium in Xalapa, Mexico. Most of the seedlings were only identified to genus due to the lack of morphological characteristics enabling further identification. Where previous information on the shade-tolerance of the species was available, these were classified into three groups: shade-intolerant or pioneer, intermediate and shade-tolerant (Tab. S1 in Supplementary material).
Data analysis
A timber extraction index was constructed based on the sum of three variables: the numbers of cut tree stumps, logs and kilns. Moreover, the tree stumps were also categorized into diametric size-classes. Since extraction data was recorded in only one sampling event per site, no measurements of variation were calculated. Based on the obtained values of the extraction index, the sites were grouped into two categories: low (L) and high (H) selective logging.
A mixed general linear model (GLM) was applied to evaluate the differences among the levels of extraction in terms of tree densities (≥10 cm DBH), DBH, basal area and canopy coverage. The extraction intensity was considered a fixed factor within the model with two levels (L and H), while the site was included as random factor ([41]). Adult tree density values were Box-Cox (λ=0) transformed in order to comply with the assumptions of normality ([9]). To assess differences in the density of seedlings and saplings between the two extraction intensities, a generalized linear model was used with a log-link function and a Poisson type distribution ([41]) using the software package SPSS® ver. 20.0 (IBM Corp., Armonk, NY, USA). Linear regression was performed to determine whether a relationship existed between canopy coverage and total density of seedlings. For this analysis, the density of seedlings was Box-Cox (λ=0) transformed and the percentage of canopy coverage was arcsine square root transformed ([9]). This analysis was performed with the software MiniTab® ver. 16 (Minitab Inc., State College, PA, USA).
To evaluate the composition of the vegetation and determine species dominance, the importance value index (IVI) was calculated for trees with DBH >10 cm. This index measures the ecological value of the species through the sum of three variables: relative number of individuals, relative frequency and relative dominance (basal area) per species ([35] - see also Tab. S2 in Supplementary material). Relative values were calculated dividing the observed value of each species by the total of species.
To calculate the diversity of adult trees in each site, the Shannon-Wiener index was applied ([32]) using the software EstimateS 9.1.0 ([7]). In the case of regeneration, an index of diversity was not determined because many individuals could not be reliably identified to the species level. Similarity between tree species in the mature canopy and in the understory layer was evaluated in each site with the Morisita-Horn index. This index is based on abundances and is not affected by bias due to sample size ([32]). Relative abundance curves were generated per genus or per species in order to compare the community of adults with the regeneration.
Results
Structure and diversity of cloud forest after traditional selective logging
Based on the extraction index, the surveyed sites were classified into two selective logging intensities: low (L) and high (H). Index values were approximately three times lower in L than in H (L1=7.5, L2= 9.2, H1=35 and H2=35, respectively). In the L sites, all of the tree stump diametric categories were underrepresented, as compared with the H sites (Fig. 1). The basal area of standing trees was significantly higher in L than in H sites (F=11.35, df=1, P=0.003). No significant differences were found between the extraction levels in terms of DBH, density of adult individuals (DBH>10 cm) and canopy coverage (P >0.05 - Tab. 1). The distribution of diametric classes among all tree species of DBH ≥10 cm in the four sites followed an inverted J pattern, but many diametric classes were not present in the H sites (Fig. 2).
Fig. 1 - Density of tree stumps by diameter class in tropical montane cloud forest fragments with low (L1 and L2) and high (L1 and L2) selective logging intensities in Veracruz, Mexico.
Fig. 2 - Percentage of trees (DBH ≥ 5cm) classified according to shade tolerance (shade tolerant, intermediate and shade intolerant) in tropical montane cloud forest with low (L1 and L2) and high (H1 and H2) selective logging intensities in Veracruz, Mexico.
A total of 37 tree species were found over all sites, belonging to 32 genera and 25 families, of which 16 species are threatened and/or included in conservation priority lists ([19]). Of the species recorded in this study, eight are used for timber; three of these are endemic to Mexico (Quercus delgadoana, Q. sartorii and Turpinia insignis) and classified as threatened ([19]).
The genus Quercus was dominant in the canopy at all sites, with a higher dominance in the L (56.4% and 67.4%) than in the H (39.9% and 60.2%) sites (Tab. S2 in supplementary material). Other dominant species at the L sites were Carpinus caroliniana (IVI = 13.6), Liquidambar styraciflua (IVI = 11.3) and T. insignis (IVI = 6.11), while at the H sites these were Clethra macrophylla (IVI = 11.5), L. styraciflua (IVI = 11.1) and Zanthoxylum melanostictum (IVI = 7.9 - Tab. S2 in the Supplementary material).
Of the 37 species recorded, 29 are reported in the literature within one of the three functional groups of shade-tolerance. Species of intermediate tolerance were the most abundant in all sites (L1 = 87.1%, L2 = 55.7%, H1 = 64.5% and H2 = 55.6%), followed by the shade-tolerant species (L1 = 12.9%, L2 = 44.3%, H1 = 27.1% and H2 = 40.0% - Fig. 2). Pioneer species were absent in the L sites and showed low relative abundance at the H sites (H1 =8.4 % and H2=4.4 % - Fig. 2).
Tree regeneration in traditionally logged cloud forests
Seedling and sapling densities did not differ between levels of extraction (seedlings: Wald X²= 1.7, df=1, P=0.19; saplings: Wald X²=0.14, df=1, P=0.70). The mean seedling density per treatment (0.97 ± 2.46 m-2 - mean ± 1 SE) was approximately double that of the saplings (0.46 ± 4.80 m-2) in all of the sites (Tab. 1). The relationship between canopy coverage and seedling density was not significant in any site (R2: L1=0.05, L2= 0.46, H1= 0.07, H2=0.11; P > 0.05). The most abundant species among seedlings were Quercus spp. in all sites (four species representing together 50.5%), followed by Ocotea psychotrioides (13%) and Cinnamomum effusum (7.3% - Fig. 3). Of the total number of seedlings and saplings, 76% belonged to the group of intermediate tolerance, 23% to that of shade-tolerance and 1% to the pioneers. According to the Morisita-Horn index, similitude among the genera and species of the canopy and those of the regeneration was relatively high in the four sites (L1= 0.86, L2 = 0.64, H1=0.69 and H2=0.71).
Fig. 3 - Relative abundance (%) of adult trees (DBH ≥ 5cm) and regeneration (seedlings and saplings) in tropical montane cloud forest with low (L1 and L2) and high (H1 and H2) selective logging in Veracruz, Mexico. (Al): Alchornea latifolia; (Aspp): Ardisia spp.; (Bm): Bernardia macrocarpa; (Cc): Carpinus caroliniana; (Ce): Cinnamomum effusum; (Cspp): Citharexylum spp.; (Cm): Clethra macrophylla; (Ca): Cojoba arborea; (Cona): Conostegia arborea; (Dspp): Deppea spp.; (Gspp): Guarea spp.; (Hm): Hedyosmum mexicanum; (Ispp): Inga spp.; (Ls): Liquidambar styraciflua; (Lo): Lonchocarpus orizabensis; (Ma): Meliosma alba; (Mspp): Miconia spp.; (Mc): Myrsine coriacea; (Op): Ocotea psychotrioides; (Ox): Oreopanax xalapensis; (Ospp): Osmanthus spp.; (Pb): Prunus brachybotrya; (Pt): Prunus tetradenia; (Pspp): Psychotria spp.; (Qspp): Quercus spp.; (Sl): Saurauia leucocarpa; (Sg): Styrax glabrescens; (Sli): Symplocos limoncillo; (Tspp): Trophis spp.; (Ti): Turpinia insignis; (Uspp): Ulmus spp.; (Zm): Zanthoxylum melanostictum.
Discussion
Effects of selective logging on cloud forest structure and diversity
Traditional selective logging is a common though illegal practice in the TMCF, with no records that could allow to quantify its intensity, frequency and extent of extraction. This study shows that the degraded TMCF in the studied plots differ in terms of the accumulated impact of selective timber extraction. In comparison with other TMCF in Mexico, even the sites with highest extraction (H) showed a relatively low exploitation ([49], [43]). In tropical montane rain forests subject to planned extraction, its intensity varies from 1 to 7 trees ha-1 year-1; in Costa Rica, this is 1.8 trees ha-1 year-1 at low intensity, 3 trees ha-1 year-1 at medium intensity and 5 trees ha-1 year-1 at high intensity ([2]), while 2 to 7 trees ha-1 year-1 are extracted in the Central African Republic ([10]). The number of tree stumps per ha in this study was between 3.3 and 29.1. However, given that the values reported are cumulative, it is likely that the actual annual rate may be less than the figures reported for other countries. Even in the sites with higher selective logging (H), a high basal area value has been maintained that is still within the interval reported for mature or conserved TMCF. For the primary TMCF of Costa Rica, basal area values of 60.7 m2 ha-1 have been reported ([28]), with values of 29.6 to 40.7 m2 ha-1 reported in the conserved TMCF of Peru ([31]).
While the TMCF are characterized by high beta diversity and elevated structural and compositional heterogeneity ([49]), the overall richness of trees found in this study (13 to 18 species per site) was lower than that reported for other TMCF in Mexico (41 to 56 species - [18], [36], [14]). The lower richness found could be the result of species local extinction, due to the legacy effects of historical over-extraction which led to the long-term reduction of diversity ([12]). In this study, the greatest diversity of adult trees was recorded in the forest with the highest intensity of extraction (H1); however, this stems from the lower dominance of shade-tolerant species and the greater contribution of pioneer species. The opening of clearings by extraction can reduce the competitive exclusion of shade-tolerant species, thus favoring pioneer species ([46]).
Chronic selective logging: threat to cloud forest regeneration?
The density of seedlings and saplings found below the stands was unaffected by the intensity of logging and was lower than that reported in other TMCF with illegal selective logging in Mexico (1-5 stems m-2 - [1]) but similar to that reported in tropical montane forests with high selective logging in East Africa (0.5-1.93 stems m-2 - [29]). Regeneration in the studied sites was mainly represented by seedlings <1 m in height, while saplings were less represented. This indicates that around 50% of the seedlings do not reach the sapling stage. However, the survival of tree seedlings could be affected by biotic and abiotic factors at the site level and requires further evaluation.
The species composition of seedlings and saplings was dominated by Quercus spp., the group of greatest interest for the production of firewood and charcoal. Growth and survival of seedlings of the genus Quercus is favored by moderate disturbance of the TMCF or partial opening of the canopy ([15], [23]) and simulated conditions of intermediate shade ([43]). Oaks also have a high capacity of resprouting from the root, allowing for their persistance in degraded sites ([27]). In contrast, the lower regeneration abundance of species with higher requirements of solar radiation (e.g., L. styraciflua and C. caroliniana) could be limited by the elevated canopy coverage observed at the study sites (85.09-89.56%). This could be due to a relatively fast canopy closure that masks the effect of selective logging on canopy ([47]).
The high similarity found between the diversity of adults and that of regeneration could be the result of the fact that the dominant species make a greater contribution to the Morisita-Horn similarity index, thus leading to underestimation of the effect of less abundant species ([32]). For example, species such as Meliosma alba, Lonchocarpus orizabensis, Hedyosmum mexicanum, Symplocos limoncillo and Ulmus mexicana were not abundant in the canopy and were not recorded in the regeneration. The little or null regeneration of rare species in the canopy can be related to various causes that include, among others: a low density of adult individuals that hampers their sexual reproduction, high rates of seed predation, low rates of germination, damage by pathogens or herbivores following germination and excessive competition with herbaceous plants or lianas ([34], [25]). Moreover, some tree populations have locally disappeared in the study region because of uncontrolled exploitation. These are mainly shade-tolerant hardwood species such as Taxus globosa and Ocotea disjunta ([39]). However, more investigations are needed to understand whether the low abundance of the rare species is due to their patterns of natural distribution in tropical forests ([25]) or is a consequence of over extraction.
Planned forestry management in tropical montane cloud forest
The development of effective forest management strategies in TMCF is complex due to its high heterogeneity and diversity and the insufficient availability of ecological information on the attributes and requirements of each species. We focused on the group Quercus spp. because: (a) these are the trees of choice for the production of firewood and charcoal; (b) their dominance in the canopy with several species; (c) their dominance in the regeneration; (d) their high capacity for resprouting; and (e) information is available about their management in other regions ([23], [21]). Planning of selective extraction of Quercus spp. for the production of firewood and charcoal could be a viable strategy, as partial cutting could also favor the growth of the remnant trees ([21]). In other forestry systems, it has been reported that extraction stimulates residual tree growth, germination and recruitment ([23], [11]).
At the landscape scale, low impact exploitation could be a viable option if complemented by the maintenance of no-felling zones within the forest (retention forestry) in order to increase and diversify tree regeneration. A scenario of low disturbance typical of fuel wood extraction in the TMCF was simulated by Golicher & Newton ([17]), who found only slight fluctuations over time in the total number of individuals and basal area. Furthermore, charcoal production has been associated with forest degradation, though not necessarily cause deforestation ([5]). Traditional selective logging in TMCF contributes to the provision of firewood and charcoal, which play an important role in energy provision to local human populations. Considering the high value of TMCF for biodiversity conservation and the increasing demand for fuel wood from tropical forests, it is essential that the planned management of TMCF includes practices such as enrichment plantations using rare and vulnerable species in order to secure the maintenance of their populations.
Conclusions
In this study, traditional selective logging in the TMCF varied across sites in terms of extraction intensity, but this variation did not impact the density and composition of tree regeneration. Oaks (Quercus spp.) were the dominant species in both the canopy and the regeneration. Tree species which were rare in the canopy and threatened at regional level were not represented in the regeneration. Therefore, we recommend the establishment of conservation areas within the TMCF with the aim of maintaining its high tree diversity and counterbalancing the detrimental effects of selective logging at the landscape level. The results of this study suggest that planned selective logging (in particular on species belonging to the genus Quercus) could be a viable management strategy compatible with the maintenance of these priority forests.
Acnowledgements
POC carried out field measurements, statistical analysis, and manuscript writing, TTA conceived the study, assisted with statistical analysis and wrote the manuscript, FLB contributed to the experimental design and drafting the manuscript, PGF contributed to the experimental design and drafting the manuscript. POC thanks CONACYT for the grant awarded (no. 280416) for a Masters degree.
This project was funded by INECOL A.C. (no. 20030-11218). We thank R. Hernández, T. Guillen, F. Lorea, C. Gallardo, P. Barret and R. García for permission to work on their properties. We thank C. Gallardo for species identification and M. Sangabriel and A. Cortés for their help with fieldwork and K. Macmillan for English revision. L. Sánchez and M. Bonilla provided helpful comments to a previous version of the manuscript.
References
Gscholar
Gscholar
Gscholar
CrossRef | Gscholar
Gscholar
CrossRef | Gscholar
Online | Gscholar
Gscholar
Gscholar
Gscholar
Gscholar
Gscholar
Gscholar
Authors’ Info
Authors’ Affiliation
Tarin Toledo-Aceves
Fabiola López-Barrera
Instituto de Ecología A. C., Carretera antigua a Coatepec 351, El Haya, Xalapa 91070, Veracruz (México)
Instituto de Biotecnología y Ecología Aplicada, Universidad Veracruzana, Zona Universitaria, Xalapa 91090, Veracruz (México)
Corresponding author
Paper Info
Citation
Ortiz-Colín P, Toledo-Aceves T, López-Barrera F, Gerez-Fernández P (2017). Can traditional selective logging secure tree regeneration in cloud forest?. iForest 10: 369-375. - doi: 10.3832/ifor1937-009
Academic Editor
Mike Perks
Paper history
Received: Dec 04, 2015
Accepted: Oct 20, 2016
First online: Mar 07, 2017
Publication Date: Apr 30, 2017
Publication Time: 4.60 months
Copyright Information
© SISEF - The Italian Society of Silviculture and Forest Ecology 2017
Open Access
This article is distributed under the terms of the Creative Commons Attribution-Non Commercial 4.0 International (https://creativecommons.org/licenses/by-nc/4.0/), which permits unrestricted use, distribution, and reproduction in any medium, provided you give appropriate credit to the original author(s) and the source, provide a link to the Creative Commons license, and indicate if changes were made.
Web Metrics
Breakdown by View Type
Article Usage
Total Article Views: 46732
(from publication date up to now)
Breakdown by View Type
HTML Page Views: 40438
Abstract Page Views: 2100
PDF Downloads: 3284
Citation/Reference Downloads: 22
XML Downloads: 888
Web Metrics
Days since publication: 2816
Overall contacts: 46732
Avg. contacts per week: 116.17
Article Citations
Article citations are based on data periodically collected from the Clarivate Web of Science web site
(last update: Feb 2023)
Total number of cites (since 2017): 15
Average cites per year: 2.14
Publication Metrics
by Dimensions ©
Articles citing this article
List of the papers citing this article based on CrossRef Cited-by.
Related Contents
iForest Similar Articles
Research Articles
Use of terrestrial laser scanning to evaluate the spatial distribution of soil disturbance by skidding operations
vol. 8, pp. 386-393 (online: 08 October 2014)
Research Articles
Modifying harvesting time as a tool to reduce nutrient export by timber extraction: a case study in planted teak (Tectona grandis L.f.) forests in Costa Rica
vol. 9, pp. 729-735 (online: 03 June 2016)
Research Articles
Shear modulus of old timber
vol. 10, pp. 446-450 (online: 24 March 2017)
Research Articles
The analytic hierarchy process for selection of suitable trees for Mexico City
vol. 13, pp. 541-547 (online: 18 November 2020)
Research Articles
Deploying an early-stage Cyber-Physical System for the implementation of Forestry 4.0 in a New Zealand timber harvesting context
vol. 17, pp. 353-359 (online: 13 November 2024)
Research Articles
Comparison of timber-house technologies and initiatives supporting use of timber in Slovenia and in Sweden - the state of the art
vol. 10, pp. 930-938 (online: 07 December 2017)
Research Articles
Shrub facilitation of Quercus ilex and Quercus pubescens regeneration in a wooded pasture in central Sardinia (Italy)
vol. 3, pp. 16-22 (online: 22 January 2010)
Review Papers
Remote sensing of selective logging in tropical forests: current state and future directions
vol. 13, pp. 286-300 (online: 10 July 2020)
Research Articles
Forest management with carbon scenarios in the central region of Mexico
vol. 14, pp. 413-420 (online: 15 September 2021)
Research Articles
Comparison of alternative harvesting systems for selective thinning in a Mediterranean pine afforestation (Pinus halepensis Mill.) for bioenergy use
vol. 14, pp. 465-472 (online: 16 October 2021)
iForest Database Search
Search By Author
Search By Keyword
Google Scholar Search
Citing Articles
Search By Author
Search By Keywords
PubMed Search
Search By Author
Search By Keyword