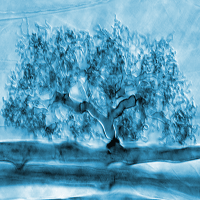
Arbuscular mycorrhizal fungi as a tool to ameliorate the phytoremediation potential of poplar: biochemical and molecular aspects
iForest - Biogeosciences and Forestry, Volume 7, Issue 5, Pages 333-341 (2014)
doi: https://doi.org/10.3832/ifor1045-007
Published: Apr 17, 2014 - Copyright © 2014 SISEF
Review Papers
Abstract
Poplar is a suitable species for phytoremediation, able to tolerate high concentrations of heavy metals (HMs). Arbuscular mycorrhizal fungi (AMF) form symbiotic associations with the roots of most land plants; they improve nutrient uptake and enhance phytoextraction of HMs while alleviating stress in the host plant. This review summarizes previous results from field and greenhouse studies conducted by us and dealing with this topic. In a field trial on a highly Zn- and Cu-contaminated site, differences in plant survival and growth were observed among 168 clones originating from natural populations of Populus alba L. and Populus nigra L. from northern Italy. After two and a half years from planting, the density, activity and metabolic versatility of the culturable fraction of the soil bacteria in the HM-polluted field was higher in the soil close to where larger poplar plants were growing, in spite of comparable HM concentrations recorded in these soils. One well-performing clone of P. alba (AL35), which accumulated a higher concentration of both metals and had high foliar polyamine (PA) levels, was used for further investigation. In a greenhouse study, AL35 cuttings pre-inoculated with AMF (Glomus mosseae or Glomus intraradices) and then transferred to pots containing soil, collected from the HM-polluted site, displayed growth comparable to that of controls grown on unpolluted soil, in spite of higher Cu and Zn accumulation. Such plants also showed an overall up-regulation of metallothionein (MT) and PA biosynthetic genes, together with increased PA levels. A genome-wide transcriptomic (cDNA-AFLP) analysis allowed the identification of a number of genes, mostly belonging to stress-related functional categories of defense and secondary metabolism, that were differentially regulated in mycorrhizal vs. non mycorrhizal plants. A proteomic analysis revealed that, depending on sampling time, changes in protein profiles were differentially affected by AMF and/or HMs. It is concluded that soil-borne microorganisms affect plant performance on HM-polluted soil. In particular, mycorrhizal plants exhibited increased capacity for phytostabilization of HMs, together with improved growth. Their greater stress tolerance may derive from the protective role of PAs, and from the strong modulation in the expression profiles of stress-related genes and proteins.
Keywords
Arbuscular Mycorrhizae, Copper, Phytoremediation, Poplar, Proteome, Soil Bacteria, Transcriptome, Zinc
Introduction
Many large areas around the world are contaminated with heavy metals (HMs) and/or organic compounds; most of these have not been remediated due to the high cost and technical drawbacks of currently available technologies. HMs tend to accumulate in soils and aquatic sediments and can enter the food chain leading to the biomagnification phenomenon thereby representing a risk to the environment and to human health ([20]). Some essential elements, such as copper (Cu) and zinc (Zn), may be present in soils and waters at potentially toxic levels mainly as a result of agricultural and industrial practices ([4]).
Alternative techniques for the clean-up of polluted soil and water, such as the cost-effective and less disruptive phytoremediation, have gained acceptance in recent years ([63], [81]). Trees have been suggested as suitable for phytoremediation due to their high biomass production ([25]) and because tree plantations can be multi-purpose ([83]). Poplar has many characteristics suitable for phytoremediation: a fast rate of growth, a deep and wide-spreading root system and a metal-resistance trait ([7], [24], [66], [73]). In Italy, P. alba (white poplar) and P. nigra (black poplar) populations of the Ticino river valley constitute a hot-spot of biodiversity ([14], [13], [30]). Their genetic variability is being exploited for the selection of genotypes having interesting traits, such as tolerance to pollutants. The remarkable clonal variability of poplar allows to identify genotypes with a greater ability to accumulate/tolerate pollutants including heavy metals ([27], [46], [50], [66], [89]). Finally, poplar offers the advantage that, being the first “model tree species” whose genome has been sequenced ([86]), physiological and molecular mechanisms at the basis of metal tolerance can be more easily investigated at the transcriptomic level ([24]).
Plant symbiotic fungi, such as mycorrhizae, and soil bacteria can confer increased tolerance to stress ([34]). Arbuscular mycorrhizal fungi (AMF) form associations with the roots of the vast majority of land plants; the fungus colonizes the roots and forms arbuscules within root cortical cells thus improving plant nutrient uptake, especially phosphorus ([76]). Moreover, increasing evidence shows that symbiotic fungi contribute to plant adaptation to multiple biotic and abiotic stresses ([35], [51], [53], [56], [70], [77]). In the case of HMs, the beneficial effect varies according to plant and fungal species, metal and concentration ([11], [51], [80], [82]). The mechanisms by which AMF offer protection from stress have not been clarified, although decreased metal uptake has been reported in some cases ([16], [59]). The potential of plant-microbe interactions in enhancing phytoremediation potential has been reviewed extensively elsewhere ([28], [51], [68]). Also in poplar, the effects of bacterial endophytes ([87]), and of endo- and ectomycorrhiza ([59]) on phytoremediation capacity have been described.
Information regarding basic molecular processes underlying metal detoxification/tolerance is scarce especially in tree species. Metallothioneins (MTs) are among the plant components that respond to metal stress. MTs are small proteins encoded by a multigene family whose members appear to be differentially regulated in relation to organ and developmental stage, and in response to a number of stimuli including HMs ([21]). A role for MTs in HM detoxification and homeostasis has been proposed either because they bind to HMs or because they function as antioxidants ([2]). The evidence is largely based on MT gene expression studies and yeast complementation experiments with plant MT genes, and some of it comes from studies on poplar species or hybrids ([9], [15], [41], [45]).
Polyamines (PAs) are organic polycations regarded as plant growth regulators ([8]); putrescine (Put
), spermidine (Spd
) and spermine (Spm
), the most abundant PAs in plants, occur both in free and conjugated forms, the latter mainly represented by phenylamides (products of the covalent binding of PAs with hydroxycinnamic acids - [58]). Due to their transcriptional and translational effects free PAs are essential for normal growth and development of eukaryotic organisms ([48]). In addition, there is abundant evidence for a stress protective role of PAs. Such evidence clearly arises from increased tolerance to multiple types of abiotic stress in plants that over-express PA biosynthetic genes ([1], [3], [36], [65]), and from experiments in which plants were treated with exogenous PAs ([37], [65], [88]). Although the exact mechanism is still unclear, PAs may act as free radical scavengers, stabilize membranes and retard senescence ([74]). Up-regulation of PA metabolism has been reported in poplars exposed to high Zn or Cu concentrations under in vitro ([31]) or greenhouse/pot ([55]) conditions and has been shown to correlate with the extent of metal tolerance.
The present review summarizes the results of our studies carried out in the last ten years, aimed at: (i) field screening for metal tolerance and accumulation of poplar clone collections established on a Cu- and Zn-polluted site; (ii) analyzing the microbial populations present in the poplar plantation on the polluted site; (iii) investigating the effect of AMF on a tolerant poplar clone selected on the polluted site; (iv) monitoring the changes in some molecular/biochemical parameters involved in stress responses; and (v) analyzing the transcriptome and proteome changes elicited in leaves by HM and/or AMF.
Field screening of tolerant poplar clones
After assessing their genetic dissimilarity through Amplified Fragment Length Polymorphism (AFLP) analysis, more than 2000 cuttings of 168 different poplar clones (40 clones of P. alba and 128 clones of P. nigra) collected along the banks of the Ticino river (near Pavia, Italy) were planted in March 2003 on a highly Cu- and Zn-polluted soil (ca. 900 and 1200 mg kg-1 dry weight soil, respectively in the top 60-cm layer) close to an industrial plant. The clones were screened for survival and growth at the end of two consecutive growing seasons; metal accumulation in plant organs and leaf PA concentrations were determined in the best performing ones ([14]). After the first growth season, survival ranged from 0 to 80% (Tab. 1); clones with more than 45% survival varied from 20 to 28%, the latter in the P. alba collection named AL. This confirmed the large clonal variability of poplar, according to previous reports on Salicaceae ([6]) and their mainly sexual reproduction ([78]). Survival after the second growth season positively correlated (R2=0.75-0.95) with plant size at the end of the first season, thus confirming the evidence that individuals with a larger biomass had a higher probability of survival ([89]).
Tab. 1 - Survival percentage and metal concentrations in selected poplar clones (AL22, AL35, NG12, NG19, SN26 and SN56) growing on a contaminated site at the end of the first growth season. Data are the mean ± SE.
Poplar clone | Survival percentage (%) |
Metals (mg kg-1 dry weight) | |||||
---|---|---|---|---|---|---|---|
Cu | Zn | ||||||
Leaves | Stems | Roots | Leaves | Stems | Roots | ||
AL22 | 66.7 | 404 ± 22 | 37 ± 8 | 78 ± 53 | 845 ± 77 | 125 ± 27 | 97 ± 30 |
AL35 | 75 | 236 ± 50 | 109 ± 13 | 568 ± 174 | 2533 ± 234 | 710 ± 23 | 1159 ± 219 |
NG12 | 75 | 162 ± 7 | 34 ± 1 | 71 ± 6 | 2012 ± 246 | 672 ± 26 | 263 ± 9 |
NG19 | 50 | 190 ± 10 | 25 ± 10 | 50 ± 10 | 1076 ± 156 | 238 ± 10 | 137 ± 10 |
SN26 | 66.7 | 171 ± 10 | 57 ± 18 | 178 ± 18 | 1889 ± 223 | 552 ± 22 | 182 ± 4 |
SN56 | 75 | 132 ± 13 | 20 ± 23 | 81 ± 23 | 879 ± 189 | 151 ± 6 | 202 ± 62 |
Six of the best-performing poplar clones were selected for further analyses. After the first growth season the highest amount of Zn was found in the leaves, as compared with stem and roots, in agreement with previous results in Salix and Populus ([26], [50]) indicating that Zn is efficiently translocated from the roots and accumulated in the leaves (Tab. 1). The clone named AL35 exhibited the highest concentration of Zn in all three organs as compared with the other clones. AL35 also displayed a high capacity for accumulating Cu, whose concentration exceeded several fold that found in other clones, particularly in the roots; the latter is in accordance with the low translocability of this metal ([12], [46], [82]).
The measure of foliar free and conjugated PA levels in the same plants confirmed that AL35 has outstanding features (Tab. 2). Although clonal variability was less accentuated than variability in metal accumulation capacity, AL35 stood out for its very high PA levels, with a prevalence of conjugated forms, which reached, in the case of Put
, a concentration 18-fold higher than that of other clones. The prevalence of conjugated PAs relative to the free forms is consistent with the abundance of phenolic compounds in poplar tissues ([84]). The strong positive correlation across clones between foliar levels of PAs and root Cu concentration (R2 = 0.79 for total PAs) suggested that Cu rather than Zn drove the long-term PA response. In fact, Cu is considered more toxic than Zn, and has been demonstrated to induce differential responses in terms of growth and PA accumulation also in micropropagated white poplar (cv. “Villafranca”) shoots cultured in vitro ([31]). Thus, in clone AL35 grown on the polluted site, the higher free and conjugated Put
levels were associated to the very high concentration of both HMs accumulated in all three organs. Given that AL35 also exhibited a very high survival rate (75%), its capacity to synthesize and accumulate large amounts of Put
likely contributed to its tolerance to HMs. It has been suggested that PAs may protect the plant through reduction of oxidative damage/lipid peroxidation by free radical scavenging and even metal chelation ([36], [57], [74]).
Tab. 2 - Free and conjugated polyamine levels in selected poplar clones (AL22, AL35, NG12, NG19, SN26 and SN56) growing on a contaminated site at the end of the second growth season. Data are the mean ± SE. (Put
): putrescine; (Spd
): spermidine; (Spm
): spermine.
Poplar clone |
Polyamines (nmol g-1 fresh weight) | |||||
---|---|---|---|---|---|---|
Free | Conjugated | |||||
Put
|
Spd
|
Spm
|
Put
|
Spd
|
Spm
|
|
AL22 | 20.4 ± 4.6 | 32.4 ± 3.4 | 20.2 ± 0.6 | 284.0 ± 41.0 | 102.0 ± 20.0 | 8.0 ± 1.0 |
AL35 | 360.8 ± 17.8 | 54.1 ± 3.4 | 0.0 | 1853.8 ± 151.5 | 287.7 ± 11.8 | 0.0 |
NG12 | 35.9 ± 5.3 | 22.7 ± 2.4 | 3.8 ± 0.1 | 205.4 ± 54.1 | 74.1 ± 6.0 | 0.0 |
NG19 | 33.3 ± 2.2 | 25.3 ± 5.9 | 6.5 ± 1.8 | 162.3 ± 0.8 | 221.4 ± 13.0 | 0.0 |
SN26 | 54.3 ± 0.9 | 67.0 ± 2.6 | 25.6 ± 2.8 | 115.0 ± 45.8 | 129.5 ± 55.0 | 31.0 ± 3.0 |
SN56 | 22.4 ± 1.2 | 57.1 ± 2.9 | 10.4 ± 0.3 | 109.0 ± 15.0 | 105.0 ± 15.0 | 0.0 |
Survey of soil microbial populations associated to poplar in the polluted site
The importance of rhizosphere bacteria in growth and development of their host plants, and the use of microorganisms or their genes for engineering plants in enhancing phytoremediation is still underestimated ([28], [32]), even though there is clear evidence that metal phytoextraction and accumulation in plants can be affected by soil microorganisms ([1], [75], [79]).
A second experimental plot was established next to the previous one on the same Cu- and Zn-polluted site (where the first poplar clone screening was performed) using the best performing white and black poplar clones from the previous trial and the commercial hybrid poplar clone I-214 (Populus × canadensis Moench) as a spacer between different clones ([33]). Although the overall survival of the clones confirmed previous results, cuttings from the same clone showed a marked variability in terms of survival and growth in different zones of the field (“position effect”). After checking that there were no significantly different Cu, Zn and phosphorus concentrations in the soil in the different zones, we turned our attention to the soil microbial populations. After two and a half years from planting, microbiological and molecular analyses were focused on microbial populations collected in proximity of the roots of large and small I-214 trees, as well as in the soil with no plants (bulk soil - [33]). I-214 trees were chosen because they were more abundant than any other tested clone (being used as spacer, their number equaled the sum of all the individuals of the other clones under study); furthermore, they were very evenly distributed on the area chosen for the study. In agreement with the “rhizosphere effect” ([40]), results showed that the density, activity and metabolic versatility of the culturable fraction of the bacteria tended to increase from the bulk soil to the soil collected beneath the large I-214 trees. Overall, about 100 culturable bacterial strains were isolated and identified from the three different soil samples (bulk soil, small I-214, and large I-214). The Denaturing Gradient Gel Electrophoresis (DGGE) profiles of the culturable fraction revealed differences in bacterial populations depending upon the soil sample. In bulk soil, all the isolated strains were Gram positive, including sporulating (Bacillus sp.) and non sporulating (Arthrobacter and Streptomyces spp.) species. Two Gram negative species (Chryseobacterium soldanellicola and Variovorax paradoxus) were preferentially associated with the poplar-planted soils. C. soldanellicola has never been reported to be tolerant to HMs, and it was previously isolated only from roots of sand-dune plants ([62]). On the other hand, V. paradoxus has been found to promote plant growth under stress conditions probaly due to its ability to synthesize 1-aminocyclopropane-1-carboxylate (ACC) deaminase, an enzyme which modulates the level of the stress hormone ethylene in plants ([10]). In the soil surrounding the large I-214 poplar plants, Flavobacterium was the prevalent genus. Flavobacteria are found in the soil and rhizosphere where they tolerate high levels of major pollutants, including HMs ([47], [64]), and promote plant growth under natural and stressful conditions ([42], [47]). Both culture-independent methods for the whole bacterial community and DGGE analysis of the culturable fraction revealed a larger biodiversity in the poplar-associated soil samples as compared to the bulk soil, strongly suggesting that poplar trees select and increment the rhizosphere-associated microflora, possibly through the release of root exudates.
Arbuscular mycorrhizal fungi improve biomass production on polluted soil
Given the interesting features of AL35, the next step was to investigate the role of AMF in poplar tolerance to Cu and Zn using this clone. To this aim, poplar cuttings were pre-inoculated as described in Lingua et al. ([55]), or not inoculated (controls), with either Glomus mosseae (Gm) or G. intraradices (Gi), now Funneliformis mosseae and Rhizophagus intraradices, respectively, according to the reviewed Glomeromycota classification by Schüßler & Walker ([72]). After one month cuttings were transferred to pots containing the same polluted (P) soil collected from the experimental site, or non polluted (NP) agricultural soil, and grown for two vegetative seasons (2006-2007) in a greenhouse. Leaf sampling was performed four (S1, July 2006), six (S2, September 2006) and 16 months (S3, July 2007) after cutting transplanting. At the end of the experiment (S3), the extent of mycorrhization (5-23%), though fairly low, was in line with previous reports ([67]). At this time, plant biomass was severely affected by HMs in the absence of AMF (up to 85% growth inhibition - Tab. 3). Interestingly, both fungal species restored plant biomass to control levels (Tab. 3), despite the generally higher HM accumulation in plant organs of mycorrhizal plants compared with those of non mycorrhizal ones (Tab. 4). In particular, Cu concentration was enhanced by both AMF in leaves and roots, while that of Zn was only enhanced by Gm in the roots. The total amount of metal accumulated by poplar organs, especially the roots, also increased dramatically in mycorrhizal plants (i.e., 10 and 37 fold in the case of Cu, and 12.5 and 5 fold in the case of Zn for Gm and Gi, respectively - [18]). In some non-woody plants, both monocot and dicot, increased uptake of metals (As, Cu, Zn) was reported in mycorrhizal plants as compared with non-mycorrhizal ones ([44], [85]).
Tab. 3 - Leaf, stem and root biomass (mean g dry weight plant-1) of P. alba clone AL35 after two seasons of growth (S3) in the greenhouse on unpolluted (NP) or polluted (P) soil in the absence or in the presence of either G. mosseae (Gm) or G. intraradices (Gi). Data are the mean ± SE (n=3).
Treatment | Biomass | ||
---|---|---|---|
Leaves | Stems | Roots | |
NP | 3.88 ± 0.82 | 10.31 ± 4.71 | 5.32 ± 2.94 |
NP-Gm | 0.76 ± 0.01 | 12.69 ± 3.14 | 3.13 ± 1.15 |
NP-Gi | 2.17 ± 0.23 | 7.20 ± 0.91 | 3.29 ± 0.16 |
P | 0.51 ± 0.07 | 1.31 ± 0.08 | 0.79 ± 0.07 |
P-Gm | 2.82 ± 0.16 | 8.24 ± 2.93 | 4.88 ± 1.80 |
P-Gi | 0.44 ± 0.04 | 8.31 ± 0.56 | 3.40 ± 0.84 |
Tab. 4 - Cu and Zn concentrations in leaves, stems and roots of P. alba clone AL35 after two seasons of growth (S3) in the greenhouse on unpolluted (NP) or polluted (P) soil in the absence or in the presence of either G. mosseae (Gm) or G. intraradices (Gi). Data are the mean ± SE.
Treatment | Metals (mg kg-1 dry weight) | |||||
---|---|---|---|---|---|---|
Cu | Zn | |||||
Leaves | Stems | Roots | Leaves | Stems | Roots | |
NP | 13.76 ± 1.3 | 8.45 ± 0.6 | 37.13 ± 3.2 | 385.22 ± 34.6 | 82.09 ± 7.2 | 92.24 ± 8.2 |
NP-Gm | 12.08 ± 0.9 | 5.71 ± 0.5 | 14.21 ± 1.2 | 269.22 ± 24.2 | 82.99 ± 7.5 | 43.79 ± 3.8 |
NP-Gi | 13.01 ± 1.2 | 5.73 ± 0.5 | 15.72 ± 1.4 | 284.97 ± 26.0 | 76.19 ± 6.9 | 37.87 ± 3.3 |
P | 20.16 ± 1.7 | 19.07 ± 1.7 | 97.56 ± 8.6 | 387.12 ± 34.9 | 126.96 ± 11.2 | 98.50 ± 8.8 |
P-Gm | 31.86 ± 2.7 | 7.72 ± 0.6 | 605.47 ± 54.3 | 532.63 ± 47.8 | 63.76 ± 5.7 | 212.11 ± 19.1 |
P-Gi | 26.90 ± 2.3 | 5.66 ± 0.5 | 244.69 ± 21.8 | 461.18 ± 41.7 | 116.40 ± 10.5 | 115.76 ± 10.3 |
Growth inhibition is a frequent symptom of HM phytotoxicity. Although the two fungal species can exert differential effects ([55]) in AL35 plants grown on P soil the restoration of biomass production by pre-inoculation with AMF indicates that mycorrhization exerted a strong protective effect against HM toxicity. The higher phosphorus concentration in mycorrhizal roots suggests that growth recovery was due at least in part to the improved nutritional status, and certainly not to the reduced HM uptake. The mechanism by which the fungal symbionts exerted their protective role has been the focus of our further investigations.
Metallothioneins are up-regulated in mycorrhizal plants
Mycorrhizal plants grew better than their non-mycorrhizal counterparts though accumulating more Cu and Zn, suggesting the presence of detoxifying mechanisms arising from cellular molecular and biochemical processes. Quantitative RT-PCR was performed to investigate the steady-state transcript levels of the poplar MT multi-gene family in mycorrhizal plants compared with non-mycorrhizal ones grown on either P or NP soil ([18]). In P soil, both AMF strongly up-regulated the expression of both a and b isogenes of PaMT1, PaMT2 and PaMT3 both at the first (S1) and third (S3) leaf sampling (Tab. 5). The largest increase was observed for PaMT1a in leaves of Gm plants. Overall, these results suggest that MTs may indeed afford protection against HM-induced stress, as previously reported in Pisum ([69]). MTs probably exert an antioxidant function as reported in transgenic P. alba cv. Villafranca plantlets over-expressing a pea MT2 gene ([9]). Although no correlation was found between MT expression and leaf metal concentration at S3 (but not S1), roots of mycorrhizal plants had accumulated one or both metals at higher concentration than controls. This would suggest that a signal coming from roots induced an up-regulation of all leaf MT mRNAs even before the plants had translocated and accumulated the metals at the leaf level. Moreover, since the fungus alone did not induce up-regulation of any of the MT isogenes on NP soil ([18]), it appears that both high metal concentration and AMF are needed to trigger such induction. This has been confirmed by more recent results from a genome-wide transcriptomic analysis ([19]). By contrast, in Brassica and in tomato, a decrease in MT expression was reported in mycorrhizal as compared with non-mycorrhizal plants. However, in tomato this was associated with lowered metal concentration in mycorrhizal plants ([23], [60]), in contrast to the results obtained from the clone AL35.
Tab. 5 - Modulation of poplar MT (MT1 to 3, isoforms a and b), ADC and SPDS (1 and 2) gene transcript levels in leaves of P. alba clone AL35 after 4 months (S1) and 16 months (S3) of growth in the greenhouse on polluted (P) soil in the absence or in the presence of either G. mosseae (Gm) or G. intraradices. Leaf mRNA levels were quantified by real-time RT-PCR and normalized with respect to actin. Arrows indicate alterations in gene expression relative to non mycorrhizal plants (on P soil).
Genes | S1 | S3 | ||
---|---|---|---|---|
P-Gm | P-Gi | P-Gm | P-Gi | |
PAMT1a | ↑ | ↑ | ↑ | ↑ |
PAMT1b | ↑ | ↑ | ↑ | ↑ |
PAMT2a | ↑ | ↑ | ↑ | ↑ |
PAMT2b | ↑ | ↑ | ↑ | ↑ |
PAMT3a | ↓ | ↑ | ↑ | ↑ |
PAMT3b | ↑ | ↑ | ↑ | ↑ |
PaADC | ↑ | ↑ | ↔ | ↔ |
PaSPDS1 | ↓ | ↑ | ↑ | ↑ |
PaSPDS2 | ↓ | ↑ | ↓ | ↑ |
Plant PA metabolism positively responds to mycorrhization
In the field trial on the polluted site, the high-performing AL35 clone exhibited an endogenous concentration of Put
that was 18-fold higher than in the other clones. This corroborates the idea that PAs have a major role in conferring metal tolerance to plants. In subsequent greenhouse experiments performed with this clone, a different response to AMF inoculation was observed for genes encoding for the PA biosynthetic enzymes arginine decarboxylase (ADC), responsible for Put
biosynthesis, and spermidine synthase (SPDS), which forms Spd
from Put
. Transcriptional changes associated with colonization by G. mosseae or G. intraradices in roots of Medicago truncatula Gaertn. also revealed several hundred genes that were either up- or down-regulated by only one of the two fungal species ([43]). At first sampling (S1), an up-regulation of PaADC occurred in leaves of mycorrhizal AL35 plants grown on P soil relative to non-mycorrhizal controls, while at third sampling (S3) PaADC was down-regulated in the same plants (Tab. 5). PaADC up-regulation is in line with the purported role of this enzyme in plant responses to different stresses including metal stress ([3], [36], [65]). No Put
was accumulated possibly because it was transformed into Spd
and/or Spm
. At third sampling, PaSPDS1 and PaSPDS2 transcripts were both induced by AMF (Tab. 5). As a result of PaSPDS up-regulation, free Spd
titres were higher in the presence than in the absence of AMF and correlated with improved plant growth; conjugated Spd
and Spm
levels were also dramatically enhanced in plants inoculated with G. intraradices relative to uninoculated controls. Although enhanced Put
titres seem to be the common physiological response to HM stress ([14], [31], [36], [52]), the accumulation of the higher PAs Spd
and Spm
seems to be typical of mycorrhizal plants grown under abiotic stress ([71]). The positive role of PAs, especially Spd
and Spm
, possibly relies on their polycationic nature allowing a high biological activity ([39]), such as binding with charged macromolecules. PAs may also afford protection from HM-induced stress by exerting an antioxidant activity, and/or by chelating metals ([37], [49]). Moreover, the singlet oxygen quenching capacity of phenylamides and their free radical scavenging properties have been established ([29]). These molecules are regarded as end-products of cellular metabolism (secondary metabolites), and long-distance signalling from mycorrhizal roots has been previously reported ([22], [38]), resulting in the enhancement of secondary metabolite production in leaves.
The leaf transcriptome and proteome are modulated in mycorrhizal plants
Transcriptome analysis
Given the beneficial effects of AMF inoculation on poplar trees grown on HM-contaminated soil and the modified expression of major stress-related genes by fungal symbiosis, the hypothesis that a broader range of genes is involved in the improved growth performance of mycorrhizal AL35 plants on P soil relative to non-mycorrhizal ones was pursued. A genome-wide transcriptomic analysis was conducted by cDNA-AFLP on lea- ves of AL35 plants grown on NP or P soil, the latter in the presence or in the absence of G. mosseae or G. intraradices ([19]). A comparison of the cDNA-AFLP patterns of all four experimental conditions at S1 revealed that a large number of transcription-derived fragments (TDFs) were differentially modulated, and that AMF inoculation strongly modified the leaf transcriptome, partially restoring it to the control profile ([19]). Most of the sequenced TDFs had similarities with database entries with known function and thus the identified TDFs were assigned to functional categories. The largest group of cDNA sequences (18% of the total) corresponded to secondary plant metabolism, while defense, photosynthesis/energy, and intracellular traffic categories were also well represented (11%). Thirteen genes whose expression was altered in mycorrhizal plants plus MT, ADC, and SPDS genes were selected as representing important functional categories, including secondary metabolism and defense, and analyzed by qRT-PCR.
Results showed that the vast majority of the selected genes was not affected or down-regulated on P soil, but up-regulated by one or both AMF, especially G. mosseae (Tab. 6). This group includes defense genes such as thaumatin-like protein (TLP), glutathione synthase (GSH) and several MTs, but also genes involved in primary metabolism and transcription. Another group was down-regulated by HMs and restored to control levels or up-regulated in mycorrhizal plants (Tab. 6). They mainly belong to defense (MTs, remorin, peroxidase) and secondary metabolism (prephenate dehydratase) categories; the latter category included also ADC gene. Thus, most genes whose expression was strongly altered belong to stress-related functional categories. Most of these genes are reported for the first time in response to HM stress, and in particular to AMF colonization. It was also shown based on their expression pattern that the fungal species behave differently. In particular, G. mosseae appears to have a better capacity than G. intraradices to activate the plant’s defense system as previously hypothesized in the case of cv. Villafranca, which was able to recover growth when cultivated on artificially Cu or Zn polluted soil ([55]). These results were further corroborated by means of a MSAP (Methylation Sensitive Amplified Polymorphism) analysis aimed at highlighting epigenetic modifications of the DNA in the presence of HMs and/or AMF ([17]).
Tab. 6 - Modulation of transcript levels of genes selected, following a cDNA-AFLP analysis, in leaves of P. alba clone AL35 after 4 months (S1) of growth in the greenhouse on polluted (P) soil in the absence or in the presence of either G. mosseae (Gm) or G. intraradices (Gi). Leaf mRNA levels were quantified by real-time RT-PCR and normalized with respect to actin. Arrows indicate alterations in gene expression relative to non mycorrhizal plants grown on NP soil.
Genes | S1 | ||
---|---|---|---|
P | P-Gm | P-Gi | |
Clathrin protein | ↑ | ↓ | ↓ |
Phytochelatin synthase | ↑ | ↔ | ↑ |
Chlorophyll binding protein | ↔ | ↑ | ↑ |
Pyridoxine-5’-phosphate oxidase | ↔ | ↑ | ↑ |
Nuclear transport factor 2 | ↔ | ↑ | ↑ |
Teosinte-branched-like protein | ↔ | ↑ | ↔ |
S-adenosylmethionine-dependent methyltransferase | ↔ | ↑ | ↔ |
Glutathione synthase | ↔ | ↑ | ↑ |
Thaumatin like protein | ↔ | ↔ | ↑ |
Remorin protein | ↓ | ↔ | ↑ |
Arginine decarboxylase | ↓ | ↔ | ↔ |
Prephenate dehydratase | ↓ | ↑ | ↔ |
Polygalacturonase | ↓ | ↔ | ↔ |
Peroxidase | ↓ | ↑ | ↑ |
Glutamine synthase | ↓ | ↑ | ↔ |
Auxin responsive protein | ↓ | ↑ | ↔ |
A cDNA microarray approach was pursued in order to investigate the expression of some genes involved in antioxidant metabolism and metal homeostasis in leaves and roots of AL35 poplar plants inoculated with G. mosseae ([61]). A total of twenty-six genes were considered, including eight superoxide dismutases (4 CuZnSod, 2 FeSod, 2 MnSod), three catalases (Cat), three ascorbate peroxidases (Apx), one dehydroascorbate reductase (Dhar), one γ-glutamylcysteine synthase (Ecs), two glutathione reductases (Grc), six metallothioneins (MT, only in roots), one metal transporter (Mtp), and one phytochelatin synthase (Pcs). Microarray data were validated by RT-qPCR on five of the above-mentioned genes. Plants grown on P soil generally exhibited higher transcript levels. However, some of the responses were clearly tissue specific; for instance, Mtp and Pcs were down-regulated in roots, but up-regulated in leaves. Similarly, MT1a and MT1b were down-regulated in roots. In general, ANOVA indicated that the metal treatment affected the transcription of a larger number of genes involved in antioxidant metabolism in leaves than in roots, suggesting that metals in roots might be more abundant in the apoplast than in the symplast. Mycorrhizal plants grown on P soil grew better and showed a down-regulation of most antioxidant genes, in spite of a higher concentration of metals in their tissues, suggesting a higher degree of protection in this plant and a lower need of activating antioxidant pathways implicated in ROS scavenging. Similar results were previously reported in the case of mycorrhizal Pisum sativum ([69]) and Medicago truncatula ([5]) under Cd stress.
Proteomic analysis
A proteomic analysis was performed on the leaves of clone AL35 inoculated or not with G. intraradices and grown in the greenhouse on P or NP soil ([54]). Protein expression was evaluated four (S1), six (S2) and 16 (S3) months after transplanting of the cuttings. Though the description of all proteins modulated in the four different treatments and at three time points is beyond the scope of this paper, some general observations can be summarized here. Most of the identified proteins showing variations concerned the functional groups of “photosynthesis and carbon fixation” and “sugar metabolism”. This is not surprising considering the analyzed organ. Secondly, the expression profiles varied significantly with time: at S1, 22 spots showed a differential expression between the four treatments, 52 at S2, and 66 at S3. At the first sampling time, a relevant number of proteins were involved in the functional group “protein folding”, while at the second and third sampling times this group was less represented and the previously missing groups of “oxidative damage” and “glutathione metabolism” were represented. Furthermore, at S1 all the modulated spots were affected by fungal inoculation (as shown by two-way ANOVA), while only 25% of them were affected by the metal treatment. At S2 the situation was reversed with most of the spots (94%) affected by the metal treatment and only 42% of them influenced by the fungal inoculation. At the last sampling, both factors were equally important.
Conclusions
Our results demontrate the feasibility of establishing a multi-clonal poplar stool bed on a highly Cu- and Zn-contaminated soil for phytoremediation purposes. The broad genetic variability of natural poplar collections allow high performing clones to be selected and used for soil recovery. One of the selected clones (AL35) showed outstanding features for both Cu and Zn phytoextraction and/or phytostabilization purposes. In addition, results show that plant association with bacterial and fungal microorganisms is able to greatly improve plant growth performance on a metal-polluted soil. Evidence is provided that stress recovery may arise from the protective role of MTs and PAs, whose genes are specifically up-regulated by the plant-AMF interaction. Large-scale molecular analyses confirm that the symbiosis determines several changes at the transcriptional/translational levels, which presumably underlie the improved performance of plants under stressful conditions. Data resulting from the proteomic analysis shall be integrated and compared with those from transcriptomic and biochemical analyses, according to a system biology approach, in order to exploit the different sensitivity of the various techniques, and provide a general framework for the plant’s response to HMs. In the future, the acquired knowledge on candidate genes/proteins involved in AMF-enhanced poplar tolerance could represent the basis for molecular engineering efforts to obtain tolerant plants to be used in phytoremediation activities.
Acknowledgments
This research was supported by funds from the Italian Ministry for Education, University and Scientific Research (PRIN 2003077418 and PRIN 2005055337) and from the Italian Ministry of the Environment, Land and Sea Protection (“Research and development in biotechnology applied to the protection of the environment”) in collaboration with The People’s Republic of China to S.C.; it is also part of the doctorate program carried out by A.C. at the Federico II University of Naples (IT).
References
CrossRef | Gscholar
CrossRef | Gscholar
CrossRef | Gscholar
CrossRef | Gscholar
CrossRef | Gscholar
CrossRef | Gscholar
Gscholar
Gscholar
CrossRef | Gscholar
CrossRef | Gscholar
CrossRef | Gscholar
Gscholar
Gscholar
CrossRef | Gscholar
CrossRef | Gscholar
CrossRef | Gscholar
Authors’ Info
Authors’ Affiliation
Stefano Castiglione
Dipartimento di Chimica e Biologia, Università di Salerno, Fisciano (SA - Italy)
Dipartimento di Scienze Agrarie, Università di Bologna, Bologna (Italy)
Guido Lingua
Dipartimento di Scienze e Innovazione Tecnologica, Università del Piemonte Orientale, Alessandria (Italy)
Dipartimento di Scienze Biologiche, Geologiche e Ambientali, Università di Bologna, Bologna (Italy)
Corresponding author
Paper Info
Citation
Cicatelli A, Torrigiani P, Todeschini V, Biondi S, Castiglione S, Lingua G (2014). Arbuscular mycorrhizal fungi as a tool to ameliorate the phytoremediation potential of poplar: biochemical and molecular aspects. iForest 7: 333-341. - doi: 10.3832/ifor1045-007
Academic Editor
Alberto Santini
Paper history
Received: May 28, 2013
Accepted: Dec 31, 2013
First online: Apr 17, 2014
Publication Date: Oct 01, 2014
Publication Time: 3.57 months
Copyright Information
© SISEF - The Italian Society of Silviculture and Forest Ecology 2014
Open Access
This article is distributed under the terms of the Creative Commons Attribution-Non Commercial 4.0 International (https://creativecommons.org/licenses/by-nc/4.0/), which permits unrestricted use, distribution, and reproduction in any medium, provided you give appropriate credit to the original author(s) and the source, provide a link to the Creative Commons license, and indicate if changes were made.
Web Metrics
Breakdown by View Type
Article Usage
Total Article Views: 48838
(from publication date up to now)
Breakdown by View Type
HTML Page Views: 40664
Abstract Page Views: 2543
PDF Downloads: 4415
Citation/Reference Downloads: 24
XML Downloads: 1192
Web Metrics
Days since publication: 3754
Overall contacts: 48838
Avg. contacts per week: 91.07
Article Citations
Article citations are based on data periodically collected from the Clarivate Web of Science web site
(last update: Feb 2023)
Total number of cites (since 2014): 23
Average cites per year: 2.30
Publication Metrics
by Dimensions ©
Articles citing this article
List of the papers citing this article based on CrossRef Cited-by.
Related Contents
iForest Similar Articles
Research Articles
Arbuscular mycorrhizal fungal symbiosis with Sorbus torminalis does not vary with soil nutrients and enzyme activities across different sites
vol. 8, pp. 308-313 (online: 03 September 2014)
Research Articles
Arbuscular mycorrhizal colonization in black poplar roots after defoliation by a non-native and a native insect
vol. 9, pp. 868-874 (online: 29 August 2016)
Research Articles
Heavy metal accumulation characteristics of Nepalese alder (Alnus nepalensis) growing in a lead-zinc spoil heap, Yunnan, south-western China
vol. 7, pp. 204-208 (online: 27 February 2014)
Research Articles
Effects of arbuscular mycorrhizal fungi on microbial activity and nutrient release are sensitive to acid deposition during litter decomposition in a subtropical Cinnamomum camphora forest
vol. 16, pp. 314-324 (online: 13 November 2023)
Research Articles
Shifts in the arbuscular mycorrhizal fungal community composition of Betula alnoides along young, middle-aged plantation and adjacent natural forest
vol. 13, pp. 447-455 (online: 07 October 2020)
Research Articles
Effect of plant species on P cycle-related microorganisms associated with litter decomposition and P soil availability: implications for agroforestry management
vol. 9, pp. 294-302 (online: 05 October 2015)
Short Communications
A quick screening to assess the phytoextraction potential of cadmium and copper in Quercus pubescens plantlets
vol. 10, pp. 93-98 (online: 13 October 2016)
Research Articles
Soil nutrient status, nutrient return and retranslocation in poplar species and clones in northern Iran
vol. 6, pp. 336-341 (online: 29 August 2013)
Review Papers
Shaping the multifunctional tree: the use of Salicaceae in environmental restoration
vol. 6, pp. 37-47 (online: 21 January 2013)
Research Articles
Dielectric properties of paraffin wax emulsion/copper azole compound system treated wood
vol. 12, pp. 199-206 (online: 10 April 2019)
iForest Database Search
Search By Author
Search By Keyword
Google Scholar Search
Citing Articles
Search By Author
Search By Keywords
PubMed Search
Search By Author
Search By Keyword