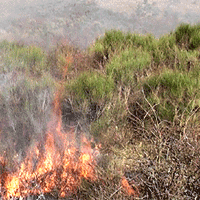
Post-fire effects and short-term regeneration dynamics following high-severity crown fires in a Mediterranean forest
iForest - Biogeosciences and Forestry, Volume 5, Issue 3, Pages 93-100 (2012)
doi: https://doi.org/10.3832/ifor0612-005
Published: May 30, 2012 - Copyright © 2012 SISEF
Research Articles
Abstract
Resilience against fire disturbance of Mediterranean vegetation has been frequently described. However, due to climatic change and abandonment of local land use practices, the fire regime is changing, probably leading to higher intensities and frequencies of disturbance events. The forthcoming scenario calls for a full understanding of post-disturbance tree recruitment processes, structural resilience and possible consequences on the overall forest biodiversity. In particular, knowledge on severe crown fires’ effects on forest stand structural attributes needs to be further explored. In this work, we describe and quantify fire impact and short-term response of a Mediterranean forest affected by high severity crown fires, focusing on the compositional and structural diversity of living and dead trees, spatial pattern of fire-induced mortality, recovery dynamics of tree species. The analysis, based on a synchronic approach, was carried out within four burned and two not burned fully stem-mapped research plots located in NW Italy, belonging to two forest categories differing for their main tree restoration strategies. Distance-dependent and distance-independent indices were applied to assess structural diversity dynamics over time since fire occurrence. Within the analyzed forests fire was found to affect mostly forest structure rather than its composition. Number of snags largely increases immediately after the fire, but it levels off due to their fall dynamics. Regeneration strategies and fire severity influenced species abundance and consequently diversity patterns. Stem diameter and height diversity were modified as well, with a strong increase in the first post-fire year and a sharp reduction six years after the disturbance. Fire determined also a higher heterogeneity in crown cover and vertical structure. Spatial patterns of surviving trees and snags were greatly affected by fire, producing an increase in aggregation and segregation mechanisms. Autosuccessional processes are supposed to preserve current forest structure and composition, but the ecosystem self-restoring capability should be analyzed in the light of the possible changes in fire regime.
Keywords
Structural diversity, Snag dynamics, Pinus pinaster, Regeneration strategies, Fire severity
Introduction
Fire is recognized as the most important natural disturbance in Mediterranean ecosystems ([71], [78]). In the Mediterranean basin, given the long historical human impact on ecosystems, the incidence of fire has always been particularly high ([38], [68]). In addition to natural causes of ignition, burning was one of the management tools commonly used by man ([54], [40]). Fire thus acted as a fundamental factor on ecosystem functioning and as a major ecological driver of vegetation changes ([3], [51], [36]), by modeling landscapes ([20]) and contributing to maintain habitat heterogeneity and biological diversity ([50], [5]).
Even though fires have been a major factor in Mediterranean ecosystems for millennia, the general trend in number of fires and area burned in European Mediterranean areas has dramatically increased during the last decades, principally due to land-use and climatic changes ([61], [40], [52], [21], [58]). According to the predicted climate change scenarios for the Mediterranean region, further increases in wildfire frequency and burned areas are expected ([10], [22]).
Although Mediterranean vegetation is able to cope with fire ([68], [56]), alterations in the fire regime (in terms for instance of higher fire recurrence or incidence of large events) can generate important consequences for Mediterranean ecosystems ([81], [74], [41]) and their plant diversity. Given the very strong effect of fire on vegetation, plant distribution and ecosystem function could likely be more heavily affected by the ongoing modifications in fire regime consequent to global changes than by the direct effect of climate changes ([58]). Moreover, the combined impact of these fire regime changes and enlarged environmental limitations for post-fire tree recruitment could deeply affect the species composition of Mediterranean forests ([59], [60], [42], [43]).
It is well documented that Mediterranean ecosystems recover readily after fire through an autosuccessional process ([26], [71]) based on efficient regeneration strategies. Post-fire recovery is usually realized by direct regeneration, i.e., the fast recovery of a plant community made up by the same species pool that existed before the disturbance ([65]). Two main plant regeneration strategies conferring resilience to Mediterranean ecosystems are the capacity to resprout after fire (resprouter species), and the stimulation of the recruitment by fire (seeder species - [6], [77]). In several fire-prone communities varying proportions of woody sprouters and seeders are included ([27], [44]). Fire regime may influence the relative abundance of sprouters and seeders ([39]). Since significant changes in disturbance regimes are predicted by global change scenarios, more attention must be paid to the ecosystems’ ability to recover ([14]). Among the main research needs for Mediterranean forests, key topics are resilience to disturbance in general ([37], [67]) and post-fire recruitment processes in particular ([75]).
The main aim of this work was to describe and quantify short-term vegetation response after high-severity crown fires in a Mediterranean forest with stands featuring a different composition of seeder and resprouter woody species in the tree layer. Recovery processes were investigated to disclose differences in resilience mechanisms among species with different restoration strategies. Specific research goals were the assessment of: (a) compositional and structural diversity of living and dead trees; (b) spatial pattern of fire-induced mortality; (c) recovery dynamics of tree species.
Materials and methods
Study site
The study was carried out in the province of Savona, Central Liguria, NW Italy (lat 44° 12’ - 44° 19’ N, long 8° 22’ - 8° 28’ E). The geologic bedrock is quite heterogeneous and is mainly represented by Cenozoic and Mesozoic igneous and metamorphic rocks. The climate is typical Mediterranean, with mild and moderately moist winters and warm and dry summers, characterized by a significant summer drought. Along with a few remaining monospecific Pinus pinaster Aiton stands, the majority of forests are mixed stands, with both conifer (mainly P. pinaster) and broadleaved species (including mainly oaks - Quercus pubescens Willd., Quercus ilex L. -, as well as Castanea sativa Miller, Fraxinus ornus L., and other woody species like Erica arborea L., Arbutus unedo L.) or with a predominance of broadleaves. The fire regime, as throughout Liguria Region, is characterized by frequent and severe wildfires, spread all over the year, but mostly concentrated during summer ([47]).
Two high severity crown fire events, located in close proximity (< 10 km), were selected for a case study. The first wildfire occurred in August 1998 and burned 146 ha, while the second occurred in August 2003 and burned 235 ha. Burned areas within the fire perimeters were differentiated according to the percentage of seeder and sprouter species in the overstory, identifying two main forest categories: the mixed-broadleaved forest category (henceforth: MB) characterized by the predominance of sprouters, and the broadleaved-coniferous forest category (BC) made up by both sprouters and obligate seeders.
Sampling
We established one intensive sampling plot for each forest category inside the fire perimeters (BC_03; BC_98; MB_03; MB_98) and another one for each forest category in the surrounding unburned area as control plot (BC_nb; MB_nb). The latter were not burned by high intensity fires since 20 years at least.
Each plot (50 x 50 m) has one side parallel to the maximum slope. Within each plot all living trees and shrubs (stems with dbh ≥ 3 cm), snags (standing dead trees with dbh ≥ 3 cm and height > 1 m), logs (downed dead trees or part of, with the biggest size diameter ≥ 10 cm), and stumps (surface diameter ≥ 10 cm and height ≤ 1 m) were identified, labeled with numbered plastic tags and mapped with a total survey station (Geotronics - Geodimeter 400). Sampling activities were carried out in summer 2004.
For every living stem, we recorded species, diameter at breast height (dbh), total height, height of lowest living branches (upslope and downslope), four radii of the crown projection on the ground. For each snag, log or stump inside the plot, the species based on distinguishing traits not modified by fire, the diameter (dbh for snags, diameter on both ends for logs, diameter at the root collar for stumps) and the total height (snags, stumps) or length (logs) were recorded. The number of post-fire resprouts of tree and shrub species was also recorded. Tree seedlings and saplings were recorded within sixteen square subplots (6.25 m2) randomly established inside each 50 x 50 m plot.
Structural diversity
Structural diversity within plots was assessed applying both distance-independent and distance-dependent measures. For more details on the diversity indices used, see Appendix 1.
The Brillouin index (HB) and the Evenness (E) for the Brillouin index ([7], [46]) were computed to describe the variability in woody species abundance.
Following Kuuluvainen et al. ([34]), we used the distribution of tree heights to represent the vertical structure of the forest. The tree height diversity (THD) index was computed for living stems and snags ([35]), adopting 2.5-m-deep horizontal layers. We also applied the tree diameter diversity (TDD) index ([66]) to 5 cm dbh classes of living and dead stems.
The vertical distribution of canopy cover within the plots was assessed through the Vertical Evenness (VE) index by Neumann & Starlinger ([55]).
To describe the tree spatial pattern within the plots, Point Pattern Analysis (PPA) techniques were applied by means of Ripley’s K ([64]) using the software SPPA 2.0 ([25]). Since the cumulative K-function confounds effects at larger distances with effects at shorter distances ([19]), the O-ring functions ([80]) were adopted as complementary analyses using the PROGRAMITA software ([79]).
To determine the distributions of trees or tree-classes as random, regular or clumped, univariate Ripley’s K(t) and O(r) functions were applied. To assess the relationships between classes (i.e., living vs. dead), we examined bivariate spatial interactions using Ripley’s K12(t) ([45]) and O12(r) functions ([80]).
We considered as living the individual stools showing at least one shoot (dbh ≥ 3 cm) alive. All the analyses were carried out only for classes having more than 20 elements, and starting from 1 m up to 25 m applying a 1 m lag distance, thus not exceeding half of the study area in order to limit the influence of the margin effects ([24]).
Null models were chosen for the different analyses to avoid misinterpretation of the results ([23]). Complete spatial randomness (CSR) was adopted as null model for the univariate analyses. For the living vs. dead stems analysis, we chose the null hypothesis of random mortality applying the random labeling ([33]), since the mortality agents (i.e., wildfires) can only work within the limits set by the distribution of living trees prior to the disturbance events themselves ([1]).
Results
A total of 6482 living stems, snags, logs and stumps were recorded, mapped and measured in the tree layer of the 6 investigated plots. Living stem density (Tab. 1) ranged from 182 stems ha-1 (BC_03) to 3787 stems ha-1 (MB_nb). Snag density ranged from 345 stems ha-1 (BC_nb) to 2716 stems ha-1 (BC_03). Basal area of living stems (Tab. 1) ranged from 2.9 m2 ha-1 (BC_98) to 16.8 m2 ha-1 (MB_nb). Concerning snags, the lowest basal area (1.1 m2 ha-1) was also recorded in MB_98, while the maximum value (23.8 m2 ha-1) was found in BC_03.
Tab. 1 - Stand characteristics of the six investigated plots. (BC_nb): not burned broadleaved-coniferous forest stand; (BC_03): broadleaved-coniferous forest stand burned in 2003; (BC_98): broadleaved-coniferous forest stand burned in 1998; (MB_nb): not burned mixed-broadleaved forest stand; (MB_03): mixed-broadleaved forest stand burned in 2003; (MB_98): mixed-broadleaved forest stand burned in 1998.
Plots | BC_nb | BC_03 | BC_98 | MB_nb | MB_03 | MB_98 |
---|---|---|---|---|---|---|
Mean elevation (m a.s.l.) | 277 | 231 | 277 | 236 | 225 | 170 |
Aspect | N-E | N-E | N-E | S-E | S-W | N-W |
Slope (degrees) | 22 | 28 | 22 | 29 | 19 | 17 |
Living stem density (stems ha-1) | 2310 | 182 | 929 | 3787 | 394 | 994 |
Living stem basal area (m2 ha-1) | 15.8 | 5.8 | 2.9 | 16.8 | 7.9 | 4.9 |
Snag density (stems ha-1) | 345 | 2716 | 1421 | 425 | 2643 | 348 |
Snag basal area (m2 ha-1) | 4.3 | 23.8 | 10.1 | 2.1 | 19.9 | 1.1 |
Regeneration density (n ha-1) | 9800 | 11100 | 21900 | 7300 | 11900 | 1600 |
Regeneration richness (n taxa) | 5 | 6 | 10 | 7 | 10 | 7 |
Comparable values in density of seed germinated individuals were found for unburned plots and those burned in 2003 (Mann-Whitney test; p>0.05), whereas a highly significant difference occurred in 1998-burned plots (Mann-Whitney test; p<0.001)
The size-class distribution of living stems and snags was reverse J-shaped for all the six plots (Fig. 1). Larger stems were mostly maritime pine trees, secondarily oaks and chestnut. The major difference between plots is the different contribution of snags and living stems to size classes. Along the post-fire chronosequence, the most represented size class was the 5-cm one. In the unburned plots almost 90% of stems belonging to this size class was made up by living stems, while immediately following the fire events, it included almost exclusively dead stems.
Fig. 1 - Diameter distribution of living stems and snags. Living stems are represented in black, snags in grey. Solid lines correspond to the death ratio, i.e., the proportion between dead and total stems in a given diameter class.
Among the most abundant species in the overstory, Arbutus unedo and Fraxinus ornus showed a high resprouting percentage in both 2003 plots (Tab. 2), while as expected in the two 1998-burned plots the percentage is reduced, with no significant differences in BC and MB (χ2 test; p>0.05). Erica arborea had a different behavior in the two plots burned in 2003, with a higher resprouting percentage in BC_03 (χ2 test; p<0.001). In the two 1998-burned plots the resprouting percentages are still high, with no significant differences. Similarly, Castanea sativa showed a higher resprouting percentage in BC_03 (χ2 test; p<0.05).
Tab. 2 - Number of top-killed stools and resprouting percentage [Re. (%)] in the four burned plots, for the main resprouting species. (BC_03): broadleaved-coniferous forest stand burned in 2003; (BC_98): broadleaved-coniferous forest stand burned in 1998; (MB_03): mixed-broadleaved forest stand burned in 2003; (MB_98): mixed-broadleaved forest stand burned in 1998.
Species | BC_03 | BC_98 | MB_03 | MB_98 | ||||
---|---|---|---|---|---|---|---|---|
# stools | Re. (%) | # stools | Re. (%) | # stools | Re. (%) | # stools | Re. (%) | |
Arbutus unedo | 41 | 92.7 | 18 | 44.4 | 31 | 77.5 | 35 | 25.7 |
Castanea sativa | 100 | 49 | 72 | 9.7 | 13 | 27.7 | - | - |
Erica arborea | 82 | 72 | 114 | 77.2 | 13 | 34.2 | 12 | 58.3 |
Fraxinus ornus | 74 | 68.9 | 1 | 0 | 87 | 55.4 | 7 | 0 |
Quercus ilex | - | - | 3 | 100 | 33 | 75 | 6 | 0 |
Quercus pubescens | - | - | 5 | 60 | 17 | 65.4 | 14 | 7.1 |
Higher species diversity values (HB) in the tree layer were found in both unburned plots (Tab. 3). The lowest diversity value was recorded in BC_03, while the index showed a higher value in BC_98. Within the MB plots the Brillouin index shows a decrease along the chronosequence. Lower evenness values (E) were obtained within both plots burned in 1998. BC_03 showed instead an E value higher than BC_nb. The opposite situation was found between MB_03 and MB_nb.
Tab. 3 - Structural diversity in the tree layer through distance-independent variables. Measures were computed for living trees with Brillouin and Vertical Eveness (VE) indices, while tree height diversity (THD) index and tree diameter diversity (TDD) index were calculated for both living stems and snags. (BC_nb): not burned broadleaved-coniferous forest stand; (BC_03): broadleaved-coniferous forest stand burned in 2003; (BC_98): broadleaved-coniferous forest stand burned in 1998; (MB_nb): not burned mixed-broadleaved forest stand; (MB_03): mixed-broadleaved forest stand burned in 2003; (MB_98): mixed-broadleaved forest stand burned in 1998.
Structural diversity | BC_nb | BC_03 | BC_98 | MB_nb | MB_03 | MB_98 |
---|---|---|---|---|---|---|
Richness (n taxa) | 10 | 5 | 9 | 7 | 8 | 8 |
Brillouin diversity (HB) | 1.617 | 1.077 | 1.273 | 1.55 | 1.489 | 1.402 |
Brillouin evenness (E) | 0.717 | 0.749 | 0.616 | 0.81 | 0.781 | 0.707 |
THD living stems | 1.469 | 1.838 | 0.795 | 1.28 | 1.887 | 1.026 |
THD snags | 1.516 | 1.487 | 1.122 | 0.966 | 1.716 | 0.668 |
TDD living stems | 1.02 | 1.736 | 0.296 | 0.731 | 1.713 | 0.628 |
TDD snags | 1.314 | 1.063 | 1.009 | 0.8 | 1.119 | 0.367 |
VE | 0.86 | 0.82 | 0.97 | 0.81 | 0.8 | 0.88 |
Living stems of both categories had similar THD and TDD patterns: early after fire occurrence higher values of the indices were encountered (Tab. 3). A sharp decrease was evident in the plots burned in 1998, which on the contrary showed the highest VE values. Snag THD and TDD values highlight a different behavior in BC and MB plots.
Ripley’s K(t) for living trees revealed a clumped distribution in all the plots (Tab. 4). The tendency toward aggregation was confirmed at short distances by the O-ring analysis. Only BC_03 showed a slightly reduced aggregation. Standing dead trees were clumped in BC plots, while in MB plots there was a gradient from a random distribution in MB_nb to aggregation in MB_03, with MB_98 showing an intermediate situation. The living vs. dead trees analysis revealed similar tendencies among plots burned in the same wildfire, and differences within the same forest category (Fig. 2). Repulsion was found from 3 m on in MB_98 and BC_98, while in the more recently burned plots the negative interaction was evident up to 4 m (BC_03) and 11 m (MB_03). No significant spatial interactions were found in unburned plots. All the results are consistent with the O-ring analyses (Fig. 2).
Tab. 4 - Ripley’s K(t) for living stems, snags and total stems in the six plots. Clumped distribution is indicated by “◊”, while “-” indicates no statistically significant difference from the CSR (Complete Spatial Randomness) hypothesis (p<0.01). Dark symbols (“•”) stands for the K(t) statistics being consistent with the O(r). (BC_nb): not burned broadleaved-coniferous forest stand; (BC_03): broadleaved-coniferous forest stand burned in 2003; BC_98: broadleaved-coniferous forest stand burned in 1998; (MB_nb): not burned mixed-broadleaved forest stand; (MB_03): mixed-broadleaved forest stand burned in 2003; MB_98: mixed-broadleaved forest stand burned in 1998.
Plot | Classes | t(m) | ||||||||||||||||||||||||
---|---|---|---|---|---|---|---|---|---|---|---|---|---|---|---|---|---|---|---|---|---|---|---|---|---|---|
1 | 2 | 3 | 4 | 5 | 6 | 7 | 8 | 9 | 10 | 11 | 12 | 13 | 14 | 15 | 16 | 17 | 18 | 19 | 20 | 21 | 22 | 23 | 24 | 25 | ||
BC_nb | Living stems | • | • | • | • | • | • | • | • | • | • | • | • | ◊ | ◊ | ◊ | ◊ | ◊ | ◊ | ◊ | ◊ | ◊ | ◊ | ◊ | ◊ | ◊ |
Snags | • | • | • | • | • | • | • | • | • | • | • | • | • | • | • | • | • | • | ◊ | ◊ | • | • | • | ◊ | • | |
Total Stems | • | • | • | • | • | • | • | • | • | • | • | • | • | • | • | • | ◊ | ◊ | ◊ | ◊ | ◊ | • | ◊ | ◊ | ◊ | |
BC_03 | Living stems | - | - | - | - | • | ◊ | ◊ | ◊ | ◊ | ◊ | ◊ | - | - | - | - | ◊ | - | - | - | - | • | ◊ | ◊ | • | ◊ |
Snags | - | - | • | • | • | • | • | • | • | • | ◊ | • | • | ◊ | ◊ | ◊ | ◊ | ◊ | ◊ | ◊ | ◊ | ◊ | ◊ | ◊ | ◊ | |
Total Stems | - | - | • | • | • | • | • | • | • | • | ◊ | • | • | • | • | • | ◊ | • | ◊ | ◊ | • | ◊ | • | • | • | |
BC_98 | Living stems | - | • | ◊ | • | • | • | • | ◊ | • | ◊ | • | • | ◊ | ◊ | ◊ | ◊ | ◊ | • | ◊ | ◊ | ◊ | ◊ | ◊ | ◊ | ◊ |
Snags | - | • | • | • | • | • | • | • | • | • | • | • | • | • | • | • | • | • | • | • | • | • | ◊ | ◊ | ◊ | |
Total Stems | - | • | • | ◊ | • | • | • | • | • | • | • | • | • | • | • | • | • | ◊ | • | • | ◊ | • | • | • | ◊ | |
MB_nb | Living stems | - | • | • | • | • | • | • | • | ◊ | ◊ | • | ◊ | • | • | ◊ | ◊ | ◊ | ◊ | ◊ | ◊ | ◊ | ◊ | - | - | - |
Snags | - | - | - | - | - | - | - | - | - | - | - | - | - | - | - | - | - | - | - | - | - | - | - | - | - | |
Total Stems | - | • | • | • | • | • | • | • | • | • | • | ◊ | ◊ | ◊ | ◊ | ◊ | ◊ | ◊ | ◊ | ◊ | ◊ | ◊ | ◊ | ◊ | - | |
MB_03 | Living stems | • | • | • | • | • | • | • | • | • | • | • | • | • | • | ◊ | ◊ | ◊ | ◊ | ◊ | ◊ | ◊ | ◊ | ◊ | ◊ | ◊ |
Snags | - | • | • | • | • | • | • | ◊ | • | ◊ | • | ◊ | ◊ | • | ◊ | • | • | ◊ | ◊ | ◊ | ◊ | ◊ | ◊ | ◊ | - | |
Total Stems | - | • | • | • | • | • | • | • | • | • | • | ◊ | ◊ | ◊ | ◊ | ◊ | ◊ | ◊ | ◊ | ◊ | ◊ | ◊ | - | - | - | |
MB_98 | Living stems | • | • | • | • | • | • | • | • | • | • | • | • | ◊ | ◊ | ◊ | ◊ | ◊ | ◊ | ◊ | ◊ | ◊ | • | ◊ | ◊ | • |
Snags | - | - | - | - | - | - | - | - | - | ◊ | ◊ | ◊ | - | - | - | - | - | - | - | - | - | - | - | - | - | |
Total Stems | • | • | • | • | • | • | • | • | • | ◊ | • | ◊ | • | • | ◊ | ◊ | ◊ | ◊ | ◊ | ◊ | ◊ | ◊ | ◊ | ◊ | ◊ |
Fig. 2 - Second order bivariate Ripley’s L12(t) and O-ring O12(r) analyses for living vs. dead stems inside the plots. Solid line is respectively L12(t) and O12(r), while dashed lines represent the 99% confidence envelopes under the random labelling null model. Points over the confidence envelope show positive spatial interactions (attraction), points below the confidence envelope show negative spatial interaction (repulsion), while points located inside the envelope show no significant spatial interaction.
Discussion
Fire caused a change in the population structure of both forest categories. The two communities were similar in terms of number and size of fire-killed and surviving tree stems. Fire affected mostly the smaller diameters, greatly reducing their number. At the same time, the majority of largest individuals, mainly maritime pines, were killed.
Following disturbance, the density of living stems and snags showed an opposite behaviour as a consequence of self-restoration strategies and fall dynamics. The processes of tree mortality and snag recruitment are balanced by snag decay and fall ([15]). Snag fall rates vary according to snag size, tree species, the microenvironment, cause and season of mortality. Snag longevity is a site-specific process that needs to be determined for each area of interest ([32], [15]). Smaller trees are more vulnerable to fire-induced death and usually tend to fall faster than larger diameter snags ([15], [11]). In the 1998 plots all snags belonged to the greatest size-classes.
The passage of a high intensity fire front induced a modification in the proportion of sprouters and seeders, due to the concurrent presence of species with varying degrees of resistance and resilience to fire. Regeneration strategies can directly influence species abundance and consequently both dominance and evenness values. Consistently with Lloret et al. ([39]), the higher presence of resprouters in the mixed-broadleaved forest category determined stability in species richness, even in early successional stages. Highly resilient resprouters can assure species persistence thanks to their ability to produce new shoots immediately after the disturbance event ([31], [69]).
Both forest categories showed a generalized increase in structural complexity of living stems immediately following the fire, while in the early post-fire years a decline was registered, reaching values lower that those found for unburned plots. By affecting mostly smaller size stems, fire reduced their pre-disturbance structural dominance. As a consequence, there were more even diameter and height distributions, leading to an increase in structural diversity. This was however a short-lived modification: six years after the perturbation, the high proportional abundance of sprout-origin individuals determined a renewed simplification in structural diversity
Dissimilar patterns of mortality before the disturbance generated a different behavior in the structural diversity of dead stems. In the BC category, characterized by a high presence of chestnut and maritime pine affected respectively by Chryphonectria parasitica (Murr.) Barr. and Matsucoccus feytaudi Ducasse, stem mortality was rather high and distributed among the different diameter classes also in the unburned plot. On the contrary, in the MB category, no particular factor seems to have a role in determining tree mortality, except the usual competition dynamics.
Fire determined a larger heterogeneity of the vertical structure in the earlier burned plots, enhancing the evenness of cover distribution between the different layers throught the recovery dynamics. The fire event induced an increase in aggregation within the stands. Disturbance by fire has actually been found to increase the degree of clumping ([63], [12]). Fire frequncis or intensities may differently affect patterns of aggregation ([12]). Tree mortality was clumped in all the burned sites, but mortality was also spatially aggregated in BC_nb, confirming that some allogenic mortality agents were acting in the stand.
Segregation mechanisms were generated by the fire, inducing a spatial repulsion between surviving and killed stems. Six years after the fire event, natural restoration dynamics and snag fall processes altered living and dead stems spatial relationships. Close to snags, positive afterlife effects ([30]) such as regeneration establishment and broadleaves resprouting mitigate repulsion.
Fire frequently creates a high degree of spatial variability in plant survival, which partially depends on the size of individuals ([29], [28]), fire-resistance mechanisms and flammability of species ([48]). Maritime pines were severely damaged by fire, influencing the spatial distribution of both dead and living stems. P. pinaster is actually a highly pyrophytic species ([76]), with a high resin content, possibly favoring ignition ([73]) and increasing the amount of energy released when the foliage burns.
Fire severity influences the number and the type of plants dying in a fire and their consequent spatial patterns. Fires of low or medium severity produce a highly selective mortality, depending on the species and size of each individual, while fires of high severity usually kill all individuals, regardless of species or size ([72]). In the latter situation, plant survival is often confined in scattered safe sites (cool spots), resulting in clumps of surviving stems.
Fire severity also influences post-fire regeneration in the burned area ([2]), which is also strongly dependent on the regenerative strategies of species ([57]).
Seedling recruitment was similar in richness and abundance within both forest categories immediately after fire. In early successional stages after fire, an abundant establishment of saplings and sprouts has been documented by several authors (e.g., [17]), mostly as a consequence of the reduced competition for resources. However, in sprouter-dominated communities, seeders are often unfavoured ([54], [68]). The dense shrub layer originated by the strong resprouting six years after the fire event in MB_98 probably hampered seedling survival, as confirmed by the very scarce seedling number. BC_98 has instead a very high seedling density, the majority of them being P. pinaster individuals. As found in other studies (e.g., [49]), five years after fire there is usually a high rate of pine establishment. Mortality of maritime pine seedlings is essentially affected by interspecific competition ([16]), particularly with shrub species, whose detrimental effects on genus Pinus have been often described ([13], [62]). Moreover, seeder species persistence could be endangered by the alteration of fire regimes, leading to differnces according to theit range of tolerance ([77]). Severe crown fires can negatively affect post-disturbance regeneration, causing higher damages, and reducing seed dispersal, viability and emergence rate ([16]).
Fire effects were found to affect more tree dimensions and spatial patterns rather than composition. Snag dynamics, in particular, were a key element of the observed changes in forest structure, mainly as a consequence of their short-term evolution.
The sprouter-dominated forest stands reveal a major difficulty in restoring the pre-fire conditions. Since the majority of adult conifers in the whole area were killed by the fire, the low number of pine seedlings can cause a partial failure of direct regeneration ([65]) and affect future forest composition.
The scarce number of tree seedlings six years after fire occurrence, along with the high resprouting rates and ground cover of shrub species, may suggest a shift towards a community dominated by a low structured, shrub vegetation. A similar scenario has been proposed for the Mediterranean basin with higher disturbance frequency ([53], [70]). An increase in the recurrence of high-intensity fires may alter recovery dynamics of usually resilient pine species as a consequence of shrub invasion, delaying succession processes and inducing a regression in the forest structure ([9]).
Forthcoming changes in fire regimes, yet largely unknown ([18]), and the need to study the response of fire-prone Mediterranean vegetation over several decades ([8]), make it necessary to further investigate post-disturbance structural dynamics, also through a long-term monitoring approach ([4]).
References
Gscholar
Gscholar
Gscholar
Gscholar
Gscholar
Gscholar
Gscholar
Gscholar
Gscholar
Gscholar
Gscholar
Gscholar
Gscholar
Gscholar
Gscholar
Gscholar
Gscholar
Gscholar
Supplementary Material
Authors’ Info
Authors’ Affiliation
M Garbarino
Department AGROSELVITER, University of Torino, v. L. Da Vinci 44, I-10095 Grugliasco (TO - Italy)
Department TESAF, University of Padova, v.le dell’Università 16, I-35020 Legnaro (PD - Italy)
Corresponding author
Paper Info
Citation
Marzano R, Lingua E, Garbarino M (2012). Post-fire effects and short-term regeneration dynamics following high-severity crown fires in a Mediterranean forest. iForest 5: 93-100. - doi: 10.3832/ifor0612-005
Academic Editor
Roberto Tognetti
Paper history
Received: Oct 23, 2011
Accepted: Apr 05, 2012
First online: May 30, 2012
Publication Date: Jun 29, 2012
Publication Time: 1.83 months
Copyright Information
© SISEF - The Italian Society of Silviculture and Forest Ecology 2012
Open Access
This article is distributed under the terms of the Creative Commons Attribution-Non Commercial 4.0 International (https://creativecommons.org/licenses/by-nc/4.0/), which permits unrestricted use, distribution, and reproduction in any medium, provided you give appropriate credit to the original author(s) and the source, provide a link to the Creative Commons license, and indicate if changes were made.
Web Metrics
Breakdown by View Type
Article Usage
Total Article Views: 62219
(from publication date up to now)
Breakdown by View Type
HTML Page Views: 49497
Abstract Page Views: 4074
PDF Downloads: 6943
Citation/Reference Downloads: 48
XML Downloads: 1657
Web Metrics
Days since publication: 4733
Overall contacts: 62219
Avg. contacts per week: 92.02
Article Citations
Article citations are based on data periodically collected from the Clarivate Web of Science web site
(last update: Mar 2025)
Total number of cites (since 2012): 31
Average cites per year: 2.21
Publication Metrics
by Dimensions ©
Articles citing this article
List of the papers citing this article based on CrossRef Cited-by.
Related Contents
iForest Similar Articles
Research Articles
Role of serotiny on Pinus pinaster Aiton germination and its relation to mother plant age and fire severity
vol. 12, pp. 491-497 (online: 02 November 2019)
Short Communications
Upscaling the estimation of surface-fire rate of spread in maritime pine (Pinus pinaster Ait.) forest
vol. 7, pp. 123-125 (online: 13 January 2014)
Research Articles
Post-fire recovery of Abies cephalonica forest communities: the case of Mt Parnitha National Park, Attica, Greece
vol. 11, pp. 757-764 (online: 15 November 2018)
Research Articles
Influence of salvage logging on forest recovery following intermediate severity canopy disturbances in mixed beech dominated forests of Slovenia
vol. 9, pp. 430-436 (online: 07 January 2016)
Research Articles
Post-fire recovery of the plant community in Pinus brutia forests: active vs. indirect restoration techniques after salvage logging
vol. 11, pp. 635-642 (online: 04 October 2018)
Research Articles
Historical fire ecology and its effect on vegetation dynamics of the Lagunas de Montebello National Park, Chiapas, México
vol. 14, pp. 548-559 (online: 01 December 2021)
Research Articles
Impact of wildfire on the edaphic microarthropod community in a Pinus pinaster forest in central Italy
vol. 8, pp. 874-883 (online: 05 May 2015)
Research Articles
Assessing the performance of fire danger indexes in a Mediterranean area
vol. 11, pp. 563-571 (online: 01 September 2018)
Research Articles
Stand dynamics and natural regeneration in silver fir (Abies alba Mill.) plantations after traditional rotation age
vol. 7, pp. 313-323 (online: 08 April 2014)
Research Articles
Short- and long-term natural regeneration after windthrow disturbances in Norway spruce forests in Bulgaria
vol. 11, pp. 675-684 (online: 23 October 2018)
iForest Database Search
Search By Author
Search By Keyword
Google Scholar Search
Citing Articles
Search By Author
Search By Keywords
PubMed Search
Search By Author
Search By Keyword