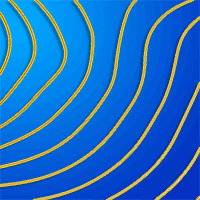
Effects of functional traits on the spatial distribution and hyperdominance of tree species in the Cerrado biome
iForest - Biogeosciences and Forestry, Volume 15, Issue 5, Pages 339-348 (2022)
doi: https://doi.org/10.3832/ifor3920-015
Published: Sep 01, 2022 - Copyright © 2022 SISEF
Research Articles
Abstract
The ecological influence of functional traits on species persistence as well as on their role over the organization of forest communities in the Brazilian Cerrado biome have not been fully understood yet. In this study, we assessed the effects of six functional groups, characterized by three seed dispersal syndromes (i.e., anemochory, autochory, and zoochory) and three wood density classes (i.e., hardwood, lightwood, and softwood), on tree spatial distribution patterns, habitat occupancy, and ecosystem services (biomass hyper dominance and abundance) provided by a forest community located in the “Parque do Lajeado”, state of Tocantins, Brazil. The similarity among study sites was characterized by applying the tree dominant height approach and the environmental and soil variables as input. The floristic similarity was assessed by applying the Bray-Curtis index. The zoochoric species showed more aggregated spatial pattern at local scale, which indicates that it is more sensitive to environmental gradients than other dispersal syndromes. Meanwhile, hardwood density species were more established in the community, being more persistent to environmental filters. We observed that a small number of species contributed with about 50% of the abundance and biomass of the community, whose functional traits (wood density and dispersal syndrome) indirectly affect the relationship among the community species richness and their ecosystem functions. We observed that the functional traits related to seed dispersal and wood density functional groups resulted in different spatial distribution patterns of those tree species. Therefore, functional traits and environmental factors combined have substantially affected the structure and composition of forest communities at local scale.
Keywords
Seed Dispersal, Wood Density, Species Abundance Distribution, Spatial Patterns, Brazilian Savanna
Introduction
In tropical regions of seasonal climates (e.g., Neotropical savanna), the available soil water content is the main limiting factor for seedling establishment and growth ([15], [45]). Climatic seasonality may affect the timing of seed production and germination patterns as well as the seedling development and establishment ([15]). The external environmental factors affect species performances and the organizational patterns of communities in time and space. However, success of plant species depends on their life history strategies ([3]). The ecological strategies can be understood as a trade-off between the functional traits of an individual plant and the environmental factors, generated by physical, physiological, or ontological constraints ([1]). The Darwinian’ natural selection has an important role on species ecological strategies, favoring morphological, physiological, and phenological characteristics involving key aspects of life history, reflecting the interaction between organism and environment ([43], [1]). There is a lack-of-knowledge of how plants use ecological strategies to occupy habitats located within the Brazilian Neotropical savanna. Therefore, a comprehensive analysis of ecological strategies related to dispersion and site occupancy of savanna tree species will provide supporting information for ecological restoration, management, and conservation of tropical ecosystems and for predicting their responses to potential future climate changes.
In this study, we aimed to understand those ecological strategies and effects of environmental factors on the plant distributions in the Cerrado biome by investigating their functional traits, as previously applied and recommended by De Missio et al. ([11]). Several ecological strategies can be understood based on functional traits ([10]), because they enable a mechanistic link between fundamental biological processes and community dynamics, as they capture essential aspects of ecophysiology, morphology and species’ life history strategy ([29]). Some of those traits have been commonly used in studies carried out in different vegetation types around the world: maximum plant height, wood density, leaf area, specific leaf area, fruit size, and seed size ([1]). Furthermore, the plant ecological strategies are generally related to some factors: competitive ability and dominance, resource acquisition, reproduction and dispersion, responsiveness to disturbances, and defense ([1], [21]).
The morphology of dispersion units reflects different adaptations of plants for seed dispersal by different ways (dispersal syndromes), for example, it is a response to a set of ecological and evolutionary factors ([46]). The dispersal is the most important process for promoting genetic diversity through the maintenance of gene flow between populations, which also affect the distribution of species at local or large scale ([25], [23]). On the other hand, wood density performs several essential functions in plants, including mechanically supporting the aboveground tissue, storing water and other resources, and transporting sap, indicating their life history and mechanical and physiological strategies ([36]). Furthermore, woody tissues are likely to face physiological, structural, and defensive trade-offs, and how a plant optimizes these competing functions can have major ecological implications, which may be measured by its growth and survival rates ([8]).
“The wood economics spectrum” refers to a manifold of co-varying wood traits around which species cluster ([8]). Two categories related to strategies of use of resources have been suggested ([14]): (I) conservative, in which plants preserve and immobilize resources acquired through protecting tissues (e.g., high density of wood); or (ii) acquisitive, with traits that provide rapid resource acquisition (e.g., low wood density). In relation to dispersal syndromes, the adaptation to the conditions of the habitats involves profound differences in the energy costs for the plants, resulting in two main models of seeds dispersal ([23], [7]). The low energetic investment model is characterized by of production numerous small seeds with less time required for maturation, usually with adaptations to dispersal by autonomous mechanisms or by non-selective vectors and can reach great distances of dispersal ([23]). On the other hand, the high energetic investment model is characterized by producing a large seed in smaller numbers, often provided with tissues or structures rich in nutrients and energy, requiring longer maturation time ([23]).
Recent studies highlight that dominant tree species are more important in maintaining vital ecosystem processes than others ([43]) such as, plant density, biomass, carbon, and fruit production ([16], [43], [39]). This unusual effect of a small number of tree species on the vital processes of the forest ecosystem is called hyper dominance ([43], [39]) and it can describe important ecosystem services at local scale ([39]). Based on it, the woody communities differ not only in species richness, but also in the dominance of their species ([30], [39]).
In our study, we focused on the functional traits and the effects of environmental factors on the tree distribution and, consequently, differentiation of ecosystem services using data collected for 82 tree species in our study site characterized by Savanna Forest (Cerradão), within the Brazilian Cerrado biome. We measured traits related to dispersal capacity, growth, and establishment in restricted environments of each tree species. We addressed the following research questions: (i) what is the relationship between the dispersal syndromes and the spatial patterns and dominance of woody species? (ii) How environmental factors may affect spatial distribution of high-density woody species? (iii) How abundant and biomass dominant are those hyper dominant species? For the first question, we hypothesized that the different dispersal syndromes lead to different spatial patterns, where autochoric species disperse their seeds close to the mother plant, giving them a more spatially aggregated pattern. Also, the zoochoric species disperse seeds over greater distances, providing a higher survival rate, and promoting greater dominance for those species. To address the second question, we hypothesized that species with high wood density occur regardless of environmental factors. Also, species showing low and medium wood densities are favored by environments with greater availability of resource and are more sensitive to environmental variables. For the third question, we hypothesized that the same hyperdominant species in relative abundance are not necessarily biomass hyperdominant, therefore, we assumed that dispersal and wood diversity traits mediate that differentiation.
Material and methods
Study area
The Cerradão (forest savanna) is a forest formation showing sclerophilic characteristics, characterized by the preferential presence of species that occur in the Cerrado sensu strictu (savanna woodland) and by forest species, forming continuous canopy and tree cover that can vary from 50% to 90%, being higher in the rainy season. The average height of the tree stratum varies from 8 to 15 m, with a predominance of semi-deciduous species on deep, well-drained and medium to low fertility soils ([38]). Our study was carried out in a Cerradão patch surrounded by savanna woodland and other vegetation types, over a total area of 12.2 ha, spatially located in the border of the Lajeado State Park, Palmas municipality, state of Tocantins, altitude of 500 m a.s.l. The Lajeado State Park is located near to Palmas city in the central-west region of the State of Tocantins, encompassing a total area of 9000 ha of countryside covered by savanna and forest formations. The climate in the study area is characterized as C2wA’a’ ([2]) with a dry season ranging from May to September and a rainy season ranging from October to April, tipically humid and sub-humid with a moderate water deficit during the winter. The average annual rainfall is 1700 mm and the predominant soil is Dystrophic Alicol Oxisol ([12]).
Forest inventory and data collection
We applied a systematic sampling for field measurements. Eight transects of 20 meters width and variable lengths were set in the study site, at 60-meters away from each other, perpendicular to the largest slope of the fragment. For floristics study, each transect was subdivided into quadrats of 20 × 20 m, totalling 54 plots and 2.16 ha sampled (Fig. 1). All tree species showing DBH ≥ 5 cm at 1.30 m height above the ground level (i.e., the diameter at breast height, DBH) were measured within each plot. Additionally, the total heights of all trees were measured, and their geographic coordinates acquired within each transect. All tree species were identified by a botanic expert in the field and double checked by herbarium specialists. Soil samples were collected for moisture and density determination. Samples were collected in trenches (down to 60 cm deep) using a Kopecky volumetric ring to measure soil density without structural deformity. Soil moisture content was determined by applying the gravimetric method at three different depths (0-20 cm; 20-40 cm; 40-60 cm): Uv = (a - b / c), in which Uv is the volumetric moisture (m³); a is the mass of the wet sample (kg); b is the mass of the dried sample (kg); c is the sample volume (dm³) ([13]).
Fig. 1 - Study area within the Lajeado State Park, Tocantins State, Brazil. The 54 sampling plots spatially located in a transition zone between Cerradão, savannah, countryside and other forest are displayed.
Aboveground biomass estimation
We used an adjusted equation to estimate the dry aboveground biomass (AGB, kg) for the study area ([32]) as defined by eqn. 1:
where DBH is the diameter at breast height (cm) and H is the total tree height (m). Total biomass (Mg ha-1) per plot was obtained by adding the AGB of all individual trees with a diameter at 1.30 m from the soil ≥ 5 cm.
Functional traits: dispersal syndromes and wood density
We determined the morphological fruit characteristics based on field observations and herbaria consultation. All plant genera identified in the study site were listed by Flora Do Brazil ([17]) and classified according to the dispersion syndromes in zoochoric, anemochoric or autochoric. We checked different secondary sources to properly classify the dispersal syndrome ([17]) by considering only their primary dispersal, according to the criteria presented by Martins et al. ([31]) and Howe ([23]):
- Autochory - Dry fruits with explosive opening or elastic dehiscence, shooting seed certain distances from the mother plant; or diaspores without any apparent adaptation to specific dispersing agents, being passively released from the parent plant when mature. Those plants showing no characteristic odors usually present pale colors with shades between brown and gray;
- Anemochory - Fruits or seeds with aerodynamic structures (wings, plumes) and / or with capacity to float in the air; or tiny and / or light seeds that are carried by the wind, showing no characteristic odors.
- Zoochory - Fleshy fruits, with nutritious tissues for fauna that commonly present flashy colors; dry fruits with arylated or carunculated seeds, or colored and mimetic seeds of aril or with floury mesocarp with nutritional rewards; dry fruits with the formation of fleshy pseudo-fruit or production of aromatic oils and resins; or diaspores without color, odor, or nutritional reserve but with structures capable of adhering to the animals’ bodies; odor present in some cases.
The wood density of each species was acquired from the Global Wood Density Database ([8]). We adopted the wood density of genus averages when it was not available at species level. We categorized the wood density in three functional groups according to the methodology adopted from Borchert ([6]) and Engert et al. ([14]): (i) lightwood, with low wood density (d <0.5 g cm-3); (ii) softwood, with intermediate wood density (0.5 ≤ d ≤ 0.8 g cm-3); and (iii) hardwood, with high wood density (d > 0.8 g cm-3).
Environmental variables
We classified the forest based on dominant height, soil density, and soil moisture content. The dominant height (Hd) was calculated as (eqn. 2):
where H is the total height, SD is the standard deviation for DBH and n the total number of obervations.
We removed the effects of the “species” to reduce autocorrelation issues in the dataset ([10]) by applying a mixed model approach and using the dominant height corrected as ê + Hd, where ê is the residual of adjusted eqn. 3 and Hd is the average height of the dominant trees ([37]).
where b is the fixed effect, s is the random effect (species), X and Z are the incidence matrices for the groups of fixed and random effects, respectively, and ê is the random error.
Finally, we estimate the environmental similarity (Euclidean distance) among the field plots by associating the dominant height (Hd) regardless of the “species” effects, based on the soil data of each plot and on an environmental data matrix. The Euclidean distances were normalized between 0 and 1 to estimate the environmental similarity, as recommended by Vega et al. ([47]).
Data analysis
Although previous studies have suggested seed-fall data and repeated censuses to assess the dispersal consequences for seedling distributions, we observed that such methodological approach is not suitable to be applied in time-scales analysis required by studies of more mature tree populations ([41]). In our study, we evaluated the floristic similarity per dispersal syndrome (Bray-Curtis Index) as a function of the distance matrix (in meters) among the 54 field-measured plots, applying the distance decay models (DDM - [47], [18]) to answer our first scientific question (“What is the relationship between the dispersal syndromes and the spatial patterns and dominance of woody species?”). Additionally, we used the function “decay.model” of the “betapart” package in software R ([5]) for adjusting the GLM using the floristic similarity as a response variable and, as predictors, the spatial distance and environmental similarity. The log link function and gaussian error distribution was used in the exponential decay model by keeping untransformed the spatial distances.
The Loess regression was used to improve the visualization of the central trend of tree dispersion patterns ([18]). The quality adjustment of those decay models was computed as pseudo r² and Akaike Information Criterion (AIC). We evaluated the effects of distance on species dispersion by including spatial dispersal patterns of each syndrome. We calculated floristic similarity separately for each functional group to minimize the potential effects of community richness on the Bray-Curtis similarity index scale.
We assessed the influence of dispersal syndromes on dominance by applying the horizontal structure analysis of the plant community, which considers the spatial distribution of all species. According to Mueller-Dombois & Ellenberg ([34]), we calculated the importance value (IV, % - eqn. 7) of each species as the sum of its relative dominance (RDo, % - eqn. 4), relative frequency (RF, % - eqn. 5) and relative density (RD, % - eqn. 6):
where G is the basal area (m² ha-1); ni and N are the number of individuals of the i-th species and the total number of individuals sampled, respectively; AF is the absolute frequency of the i-th species and TF is the total frequency.
The spatial patterns (aggregated, random, or regular) of those species contributing with 50% of the importance value were computed by applying the Nearest Neighbor analysis using the package “spatstat” in R ([4]) with α = 0.05 (eqn. 8):
where hat{d} is the average distance observed between the nearest neighbors; A is the area (m²) and n the number of points considered. For aggregate patterns, r < 1; for Poisson patterns (random), r ~ 1; for regular distribution of points, r > 1.
To answer the question of “How environmental factors may affect spatial distribution of high-density woody species”, we applied the distance decay model (DDM) using floristic similarity as a function of the environmental similarity. In this case, we assumed that environmental dissimilarity increases as floristic dissimilarity also increases ([47]).
For our third question (“How abundant and biomass dominant are those hyper dominant species?”), we constructed dominance curves to identify hyperdominant species, in which species were ordered from the most common to the rarest on the abscissa with the relative abundance and biomass on the ordinate ([35]). We considered hyperdominant species those that contributed 50% of total biomass and total relative abundance ([16], [39]). In addition, we regressed the percentage contribution of each species to biomass as a function of its percentage contribution to relative abundance. This regression was performed on the complete data set for biomass and species abundance. Prior to the analysis, the data were normalized by applying a logarithmic transformation ([16]).
Results
Horizontal structure analysis and spatial pattern of species
We observed that 5.8% of the species represent about 50% of the total importance value (IV) in the studied forest community, mostly constituted by tree species with zoochoric dispersion syndrome. Some 50% of those species showing highest relative dominance was classified as zoochoric species (15.86% and 13.54% - Tab. 1). Meanwhile, the anemochoric and autochoric species represent 8% and 7% of relative dominances, respectively. From the total sampled species, we observed that only 16.4% of species represent 80% of the importance value. The diameters of the tree species varied from 5 to 65 cm (CV = 60.9%) and the heights varied from 3 to 21 m (CV = 87.5%).
Tab. 1 - The 20 most abundant tree species in fragment of Cerradão, TO-Brazil. The data were obtained through a horizontal structure analysis. (n); number of individuals; (DS): dispersal syndromes (Z, zoochory; A, anemochory; AU, autochory); (RD): relative density (%); (RF): relative frequency (%); (RDo): relative dominance (%); (IV): importance value (%).
Species | n | DS | RD | RF | RDo | IV | IV (%) |
Cumulative IV (%) |
---|---|---|---|---|---|---|---|---|
Myrcia splendens (Sw.) DC. | 416 | Z | 16.91 | 4.51 | 15.86 | 37.281 | 12.43 | 12.43 |
Emmotum nitens (Benth.) Miers | 278 | Z | 11.14 | 5.67 | 13.54 | 30.35 | 10.12 | 22.55 |
Qualea parviflora Mart. | 211 | A | 8.58 | 5.54 | 8.13 | 22.248 | 7.42 | 29.97 |
Xylopia aromatica (Lam.) Mart. | 205 | Z | 8.33 | 5.67 | 5.02 | 19.028 | 6.34 | 36.31 |
Tapirira guianensis Aubl. | 169 | Z | 6.83 | 3.48 | 8.43 | 18.734 | 6.24 | 42.55 |
Miconia albicans (Sw.) Steud. | 229 | Z | 9.07 | 5.67 | 2.35 | 17.082 | 5.69 | 48.24 |
Parkia platycephala Benth. | 54 | AU | 2.2 | 3.87 | 6.79 | 12.85 | 4.28 | 52.52 |
Caryocar coriaceum Wittm. | 51 | Z | 2.03 | 3.48 | 6.03 | 11.544 | 3.85 | 56.37 |
Tachigali vulgaris L.G.Silva & H.C.Lima | 72 | A | 2.93 | 3.87 | 4.36 | 11.152 | 3.72 | 60.09 |
Mezilaurus itauba (Meisn.) Taub. ex Mez | 73 | A | 2.97 | 2.32 | 4.08 | 9.371 | 3.12 | 63.21 |
Maprounea guianensis Aubl. | 50 | Z | 2.03 | 3.22 | 1.25 | 6.501 | 2.17 | 65.38 |
Pouteria ramiflora (Mart.) Radlk. | 29 | Z | 1.18 | 2.32 | 2.72 | 6.223 | 2.07 | 67.45 |
Byrsonima sericea DC. | 33 | Z | 1.34 | 2.58 | 1.48 | 5.402 | 1.8 | 69.25 |
Miconia cuspidata Mart. ex. Naudin | 42 | Z | 1.71 | 2.32 | 1.36 | 5.39 | 1.8 | 71.05 |
Aspidosperma subincanum Mart. | 30 | A | 1.22 | 2.58 | 1.06 | 4.859 | 1.62 | 72.67 |
Tapirira obtusa (Benth.) J.D.Mitch. | 32 | Z | 1.22 | 2.06 | 1.2 | 4.48 | 1.49 | 74.16 |
Qualea grandiflora Mart. | 27 | A | 1.1 | 2.58 | 0.75 | 4.429 | 1.48 | 75.64 |
Erythroxylum daphnites Mart. | 36 | Z | 1.46 | 2.32 | 0.31 | 4.091 | 1.36 | 77 |
Ferdinandusa elliptica (Pohl) Pohl | 14 | A | 0.57 | 0.9 | 2.35 | 3.82 | 1.27 | 78.27 |
Byrsonima pachyphylla A. Juss. | 24 | Z | 0.98 | 2.06 | 0.75 | 3.787 | 1.26 | 79.53 |
Among the five zoochoric species showing the highest importance value, we observed that only the Emmotum nitens (Benth.) Miers showed random spatial distribution (r ~ 1; p > 0.05 - Tab. 2); whereas the four other species showed an aggregated spatial pattern (r < 1; p < 0.05). Regarding autochoric and anemochoric species, both showed random spatial patterns (r ~ 1; p > 0.05 - Tab. 2). The functional group of medium density wood (softwood) was majority in the species composition and represented about 50% of the importance value; most softwood species showed an aggregate spatial distribution, and only one representative species of the high-density wood functional group (hardwood) was randomly distributed in the study area. On the other hand, only one species representative of the functional group of low wood density (lightwood) showed a strong pattern of spatial aggregation (Tab. 2).
Tab. 2 - Spatial patterns for species that represent about 50% of the importance value in fragment of Cerradão, TO, Brazil. (r): Nearest neighbour statistic; (Z): zoochory; (A): anemochory; (AU): autochory. Methods of adjusting the edge effect through Donnelly correction.
Species | Dispersal syndromes |
Wood density |
r | p-value |
---|---|---|---|---|
Myrcia splendens (Sw.) DC. | Z | Softwood | 0.93 | 0.02 |
Emmotum nitens (Benth.) Miers | Z | Hardwood | 1.04 | 0.22 |
Qualea parviflora Mart. | A | Softwood | 0.96 | 0.36 |
Xylopia aromatica (Lam.) Mart. | Z | Softwood | 0.90 | 0.01 |
Tapirira guianensis Aubl. | Z | Lightwood | 0.76 | < 0.001 |
Miconia albicans (Sw.) Steud. | Z | Softwood | 0.92 | 0.03 |
Parkia platycephala Benth. | AU | Softwood | 0.89 | 0.19 |
Floristic similarity as a function of distance and environmental similarity
We observed that floristic similarity decreased as the distance between sample units increased (Fig. 2a), which can be explained by alternative mechanisms, such as limitations to dispersal. This pattern was observed for the community and each dispersion syndrome, except for the autochoric species, which showed less sensitivity to floristic similarity as a function of distance (Fig. 3c). The autochoric species is the functional group exclusively composed by species of the Fabaceae family. Regarding environmental similarity, we found an opposite trend, which showed an increase of environmental and floristic similarities. However, the autochoric, hardwood and lightwood species, showed less sensitivity to environmental gradients (Fig. 3f, Fig. 4d and Fig. 4f). We found that the distance between plots was an important dispersive factor (pseudo r² = 0.38, p < 0.05); however, in different environments, the species were not established (pseudo r² = 0.16, p < 0.05), resulting in low floristic similarity, even at close distances (Fig. 2a, Fig. 2b and Fig. 2c).
Fig. 2 - The Bray-Curtis Similarity Index of tree species measured for each sampling plot compared to the plot distances and environmental similarity. (a) Paired linear distances between plots (m). (b) Environmental similarity among sites based on dominant tree heights (Hd) and soil properties. (c) The interaction between the factors depicted at the figure parts “a” and “b”, placed into a surface plot. Curve fits were done using the LOESS regression procedure.
Fig. 3 - Bray-Curtis Similarity Index of the tree species observed for the sampling plots and seed dispersal syndrome: 3a, 3b and 3c) Bray-Curtis Similarity Index × geographic distances between plots for the zoochoric, anemochoric and autochoric species, respectively; 3d, 3e and 3f) Bray-Curtis Similarity Index × environmental similarity among sites based on dominant tree heights (Hd) and soil properties for the zoochoric, anemochoric and autochoric species, respectively. Curve fits were done using the LOESS regression (dashed lines) and GLM (solid lines) procedure.
Fig. 4 - Bray-Curtis Similarity Index of the tree species observed for the sampling plots and wood density functional groups: 3a, 3b and 3c) Bray-Curtis Similarity Index × geographic distances between plots for the hardwood, softwood and lightwood species, respectively; 3d, 3e and 3f) Bray-Curtis Similarity Index × environmental similarity among sites based on dominant tree heights (Hd) and soil properties for the hardwood, softwood and lightwood species, respectively. Curve fits were done using the LOESS regression (dashed lines) and GLM (solid lines) procedure.
The hardwood functional group (high wood density species), representing 24.4% of the total species in the community, had greater floristic similarity as a function of the dispersion distance and environmental similarity. Differently, the functional group of medium density (softwood species), resulted in lower values of floristic similarity, showing most aggregation and greater sensitivity to environmental filters.
Hyperdominant species: biomass and relative abundance
We found that about 6% of the species contributed with 50% of the relative abundance, while 7% contributed with 50% of the biomass stock; only Emmotum nitens (Benth.) Miers, Myrcia splendens (Sw.) DC. and Qualea parviflora Mart. contributed to both abundance and biomass, which together represented about 30% of the importance value of the forest community.
Although Myrcia splendens (Sw.) DC. mostly contributed to the relative abundance (16.8%), it ranked second in terms of contribution to biomass stock (10.12%), followed by Emmotum nitens (Benth.) Miers (11.2% for abundance and 16.2% for biomass), which belongs to the hardwood functional group of species showing zoochoric dispersion syndrome. The relative abundance of tree species showed a good predictor for estimating the biomass stock (F-test = 239, df = 80, p <0.001; coefficient of determination r² = 0.75 - Fig. 5a). The importance ranking of species relative abundance (Fig. 5b) demonstrated that tree species contributing with 50% of relative abundance partly differed from those that contributed with 50% of the biomass stock.
Fig. 5 - a) Relationship between species contributions to stem abundance and contributions to biomass. Regression models are plotted with blue line; the regression equation for the contribution rate of biomass is: log (% biomass) = -0.3483 + 1.21503 x log (% stem). (b) Species abundance distribution by relative abundance and biomass of hyperdominant tree species in the 54 Cerradao sampling plots. The dashed lines indicate the limits of tree species that accumulated up to 50% of the total abundance; or (c) 50% of total community aboveground biomass.
Discussion
Dispersal syndromes: spatial patterns and dominance
The predominance of zoochoric dispersal syndrome within forest formations was expected, since most species (about 80%) are dispersed by animals and frugivores which usually inhabit forest and other formations within the Cerrado biome ([27]). In our study, the zoochoric tree species dominated the woody community (Tab. 1). Fourteen out of twenty most abundant species are zoochoric, of which two species (Myrcia splendens [Sw.] DC. and Emmotum nitens [Benth.] Miers) are responsible for 23% of the importance value (Tab. 1). Our results indicate that local fauna has a crucial role for seed dispersion within the Cerradão vegetation type.
Spatial patterns of adult plants are a consequence of several ecological processes related to seed dispersal and recruitment. Dispersal limitation, mediated by dispersal syndrome, is considered a key factor in the formation of adult plant spatial patterns ([25]). The functional group dispersed by animals proved to be more aggregated at distances < 300 m (Fig. 3a). This likely occurs because animals do not move randomly in the space ([9]). Therefore, the intrinsic characteristics of the dispersion vectors (e.g., physiology and body size) combined with extrinsic environmental factors, can determine how animals forage, move, and deposit seeds in space and time ([9]). In our study site, we observed that, as the distance increases, there was a drastic reduction in the floristic similarity of species with zoochoric dispersion syndrome (Fig. 3a), which is potentially related to the dispersal limitation.
We observed that the reduction in floristic similarity was also the result of environmental factors (pseudo r² = 0.17, p < 0.05 - Fig. 3d). This result indicates that in heterogeneous sites, plants are spatially distributed in patchy microhabitats based on interspecific tradeoffs of resources and environmental requirements ([20]). The patterns observed in this analysis suggest that there is a predominance of the high energetic investment model adopted by the zoochoric species. It can provide seed dispersion to those habitats more likely to meet the ecological demands of new individuals ([40]).
The autochorous species showed floristic similarity independent of the distance and environmental factors (pseudo r² < 0.001, p > 0.05), opposite to the expected patterns of spreading over shorter distances, as seed germination occurs close to tree parents ([15]). This pattern can be explained by the matrix plant height, which can affect the seed dispersal distance ([46]). We assume that it is an important strategy to increase the dispersion efficiency of those species showing more limited syndromes. Taller trees were able to disperse seeds from higher heights and larger crowns ([26]), thus spreading seeds over greater distances than shorter individuals, increasing dispersion efficiency and reducing predation in the surroundings of the parent tree ([24]).
The low sensitivity of autochoric species to the environment variables (pseudo r² < 0.05, p > 0.05) is likely due to seed dormancy ([15]). Indeed, seed viability is maintained even in unfavorable environmental conditions, increasing the probability of seedling establishment when the environment becomes favorable to germination, and decreasing the competition between sibling plants ([15]). Accordingly, species with limited seed dispersal, tend to produce dormant seeds, while anemochorous and zoochorous species show dormant seeds only when dispersal occurs during unfavorable seasons, such as the rainy-to-dry season transition ([15]).
Anemochoric species has shown more floristic similarity than the zoochoric species over distances greater than 300 m (Fig. 3c), which confirms their ability to disperse seeds over longer distances ([46], [25]). Although zoochoric species can reach large dispersion distances, their seeds are often transported to random places that may be ecologically inappropriate for seedling growth. The performance of the low investment model, usually attributed to plants better adapted to dispersion by autonomous mechanisms or by non-selective vectors (e.g., anemochoric) depend on the quantitative aspects of dispersion ([23]).
We observed a close relationship between anemochory syndrome and the plant height. The anemochoric species tends to occupy the upper forest layers, where there is a stronger wind and fewer obstacles to the displacement of the diaspores ([46]). The maximum tree height of 19 m was observed for species with that syndrome. Despite of it, we observed a significant decay in floristic similarity as a function of distance and environment. We assume that this pattern occurs mainly because of the great anatomical variety of the diaspores ([31]), tree height from where the seeds are released, in addition to the presence of obstacles ([23]), which affects aerodynamics and, consequently, the distance dispersion ([44]).
Functional group of wood density: species spatial patterns according to environmental factors and distance
Our results demonstrate that species of high wood density (hardwood) were established more independently of environmental factors in habitats with high floristic similarity (pseudo r² = 0.084, p < 0.05). This can be explained as high density woods provide more tree environmental stability, greater physical defense against pathogens, and resistance to environmental disorders ([8]). In seasonal climatic environments, such as the Cerrado, functional traits of high wood density species are crucial to improve their persistence in habitats ([1]). Indeed, vessel embolisms are less likely to occur when investing in more resistant tissues with a lower concentration of conducting vessels in the xylem active area, which provides greater hydraulic reactivation during the rainy season, thus resulting in greater resistance to drought ([6], [8]).
On the other hand, the softwood functional group was more sensitive to environmental stresses (pseudo r² = 0.114. p < 0.05), leading us to hypothesize that this could be related to less efficient physiological strategies to prevent drought. Based on the above considerations, we can argue that these species compensate it by investing more in growth and reproduction. Although lightwood species are less efficient in harsh environments, they maintained a constant floristic pattern as a function of environmental factors (pseudo r² = 0.002, p > 0.05 - [6], [11]). The spatial consistency of the floristic pattern is likely due to a water storage strategy aimed to maintain photosynthesis, as well as alternative ways to avoid strong decreases of water potential during the dry season ([10]).
Our results indicates that wood density is an important functional attribute in the demographic responses of tree species to environmental gradients. Previous studies have explored the relationships between different wood density and dispersion syndromes ([22]), observing a high positive correlation between wood density and seed size in zoochoric species and a negative relationship for species not dispersed by animals. This association increases chances of any process that limits dispersion to negatively affect the forest dynamics for species with high wood density ([22]), which may have long-term effects in carbon storage and in sensitivity to forest droughts ([8], [45], [28]). In this study, the high floristic similarity of the hardwood functional group as a function of distance (pseudo r²=0.22, p<0.05), reinforce the hypothesis that differences in dispersion patterns between functional groups of wood density are strongly related to their dispersion syndromes, since in hardwood functional group dispersal is predominantly due to animals.
Hyperdominance in abundance and biomass
Previous studies on tropical forest ([16] at large scales, [39] at small scales) have shown that a small number of species contribute disproportionately to the abundance and biomass of communities. Dominance is typical in tropical ecosystems ([30]), and is common in the Cerrado sensu lato ([42]). This indirectly impacts the relationship between species richness and their role in the ecosystem ([36]). The dominance of a restricted group of species reflects their competitive advantage in the community ([42]). In this study, we found that few species contributed more than 50% to biomass (7.3%) or 50% to abundance (6.1%).
Our results indicated that Parkia platycephala Benth. (autochorous, softwood), Tapirira guianensis Aubl. (zoochorous, lightwood) and Tachigali vulgaris L.G.Silva & H.C.Lima (anemochorous, softwood) contributed with 22% to all forest biomass, but only 50% to the total abundance of the community (Fig. 5b). Based on the metabolic theory of ecology, the metabolic rate of an organism scales as a power function of its body mass. This means that larger trees accumulate organic matter much faster than smaller ones, thus leading to unproportionally higher biomass of these species ([19]). These considerations presumably explain why those species are great contributors to biomass, although they are not very abundant, as already observed in recent studies ([39]).
According to the findings of previous studies on tropical forests ([16]), we observed that more abundant species tend to contribute more to biomass stocks, with 75% of the variation in forest biomass being explained by the relative abundance of species (Fig. 5a). However, it is evident that certain species contribute more than others (Fig. 5b, Fig. 5c). We assume that functional traits, which varied between the groups of sampled species, substantially affected this differentiation. The observed predominance and greater abundance of typical savanna species such as Miconia albicans (Sw.) Steud., Qualea parviflora Mart. and Xylopia aromatica (Lam.) Mart., which are more adapted to xeric conditions, suggest that the different functional traits of these species could reflect different mechanisms of adaptation to water stress conditions, enabling a better resource sharing ([42]).
Although a predominance of typical savanna species was found for abundance hyperdominance (Fig. 4b), the forest species Emmotum nitens (Benth.) Miers and Myrcia splendens (Sw.) DC. stood out in terms of stem and biomass hyperdominance (Fig. 4b, Fig. 4c), reaching the top rank by applying both approaches. Despite Myrcia splendens has almost twice amount of individuals and ranks to the top of species abundance, Emmotum nitens (Benth.) Miers, which belongs to the hardwood and zoochoric dispersion functional groups, showed a higher contribution to the biomass stock (8.7 Mg ha-1). Some studies indicate that that difference in the contribution is influenced by functional traits, such as wood density, which may be related to more conservative characteristics in terms of use of resources, providing the conservation and immobilization of carbon via protected tissues ([16], [39]). We observed that the functional traits are key factors in differentiating the contribution of species to the ecosystem functioning; species with high wood density contribute more to the total biomass than low density wood species in tropical forests ([33]).
Conclusions
The contrasting patterns observed for hardwood and lightwood functional groups could suggest different strategies to overcome the water deficit. Indeed, hardwood species tend to be more drought-tolerant than other species, while lightwood species seem to be able to minimize drought effects by investing in lighter wood tissues. However, these groups of species have shown lower floristic similarity patterns between them at fine spatial scales.
Tree species dispersed by animals proved to be more aggregated, being more sensitive to the environmental gradients than other dispersion syndromes. Seed dispersion at this functional group tends to occur in habitats better matching the ecological demands of the recruitments. In addition, we found that species unevenly contribute to ecosystem functions, and we assumed that functional traits are key factors for explaining the observed patterns.
Defining the functional groups based on wood density and dispersion syndrome allowed to better understand the amplitude of species occurrence, define its potential to occupy different habitats, and differentiate the ecosystem services provided by those species. However, the success of dispersion mechanisms and growth strategies also depend on the habitat and are directly related to environmental factors, which interact with functional traits of each species to determine dispersion and establishment, and ultimately its spatial distribution.
Funding
This work was supported by the Research Support Foundation of the Distrito Federal (FAP-DF, Brazil), which financed the field data collection, and by the Higher Education Enhancement Coordination (CAPES, Brazil), which financed the master’s scholarship of the first author.
Acknowledgements
We would like to thank the Nature Institute of Tocantins (NATURATINS, Brazil) for managing the Lajeado State Park.
References
Gscholar
Gscholar
CrossRef | Gscholar
CrossRef | Gscholar
CrossRef | Gscholar
CrossRef | Gscholar
CrossRef | Gscholar
CrossRef | Gscholar
CrossRef | Gscholar
Gscholar
CrossRef | Gscholar
CrossRef | Gscholar
Gscholar
CrossRef | Gscholar
Authors’ Info
Authors’ Affiliation
Eder Pereira Miguel 0000-0001-6259-4594
Eraldo Aparecido Trondoli Matricardi 0000-0002-5323-6100
Alba Valéria Rezende 0000-0002-1167-9798
Fabrício Assis Leal 0000-0001-8522-0114
Mario Lima Dos Santos
University of Brasilia, Department of Forest Sciences, University Campus Darcy Ribeiro W/N, 70910-900 Brasilia, DF (Brazil)
Goias Federal University, Department of forest improvement, Avenida Esperança W/N, Samambaia Campus, 74690-900 Goinia, GO (Brazil)
Corresponding author
Paper Info
Citation
De Souza HJ, Miguel EP, Resende RT, Matricardi EAT, Rezende AV, Leal FA, Dos Santos ML (2022). Effects of functional traits on the spatial distribution and hyperdominance of tree species in the Cerrado biome. iForest 15: 339-348. - doi: 10.3832/ifor3920-015
Academic Editor
Michele Carbognani
Paper history
Received: Jul 05, 2021
Accepted: Jul 05, 2022
First online: Sep 01, 2022
Publication Date: Oct 31, 2022
Publication Time: 1.93 months
Copyright Information
© SISEF - The Italian Society of Silviculture and Forest Ecology 2022
Open Access
This article is distributed under the terms of the Creative Commons Attribution-Non Commercial 4.0 International (https://creativecommons.org/licenses/by-nc/4.0/), which permits unrestricted use, distribution, and reproduction in any medium, provided you give appropriate credit to the original author(s) and the source, provide a link to the Creative Commons license, and indicate if changes were made.
Web Metrics
Breakdown by View Type
Article Usage
Total Article Views: 19834
(from publication date up to now)
Breakdown by View Type
HTML Page Views: 17440
Abstract Page Views: 1164
PDF Downloads: 1060
Citation/Reference Downloads: 1
XML Downloads: 169
Web Metrics
Days since publication: 695
Overall contacts: 19834
Avg. contacts per week: 199.77
Article Citations
Article citations are based on data periodically collected from the Clarivate Web of Science web site
(last update: Feb 2023)
(No citations were found up to date. Please come back later)
Publication Metrics
by Dimensions ©
Articles citing this article
List of the papers citing this article based on CrossRef Cited-by.
Related Contents
iForest Similar Articles
Research Articles
Seed trait and rodent species determine seed dispersal and predation: evidences from semi-natural enclosures
vol. 8, pp. 207-213 (online: 28 August 2014)
Research Articles
The effect of seed size on seed fate in a subtropical forest, southwest of China
vol. 9, pp. 652-657 (online: 04 April 2016)
Short Communications
Effects of nitrogen, calcium and cation exchange capacity on gum yield in Acacia senegal under plantation and savanna woodland conditions in northern Guinea savanna, Nigeria
vol. 4, pp. 190-194 (online: 11 August 2011)
Short Communications
Evidence of Alectoris chukar (Aves, Galliformes) as seed dispersal and germinating agent for Pistacia khinjuk in Balochistan, Pakistan
vol. 14, pp. 378-382 (online: 22 August 2021)
Research Articles
Inter- and intra-annual patterns of seed rain in the black spruce stands of Quebec, Canada
vol. 10, pp. 189-195 (online: 13 December 2016)
Research Articles
Dispersal and hoarding of sympatric forest seeds by rodents in a temperate forest from northern China
vol. 7, pp. 70-74 (online: 18 November 2013)
Research Articles
Analysing species abundance distribution patterns across sampling scales in three natural forests in Northeastern China
vol. 13, pp. 482-489 (online: 01 November 2020)
Short Communications
Biodiversity and ecosystem functioning: exploring large-scale patterns in mainland China
vol. 5, pp. 230-234 (online: 02 October 2012)
Research Articles
Earlywood vessel features in Quercus faginea: relationship between ring width and wood density at two sites in Portugal
vol. 8, pp. 866-873 (online: 27 April 2015)
Short Communications
Towards an optimal sampling effort for paternity analysis in forest trees: what do the raw numbers tell us?
vol. 5, pp. 18-25 (online: 27 February 2012)
iForest Database Search
Search By Author
Search By Keyword
Google Scholar Search
Citing Articles
Search By Author
Search By Keywords
PubMed Search
Search By Author
Search By Keyword