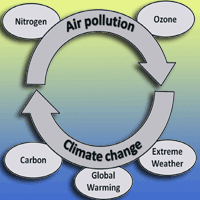
A meta-database comparison from various European Research and Monitoring Networks dedicated to forest sites
iForest - Biogeosciences and Forestry, Volume 6, Issue 1, Pages 1-9 (2013)
doi: https://doi.org/10.3832/ifor0751-006
Published: Jan 14, 2013 - Copyright © 2013 SISEF
Technical Advances
Collection/Special Issue: IUFRO 7.01.00 - COST Action FP0903, Kaunas (Lithuania - 2012)
Biological Reactions of Forest to Climate Change and Air Pollution
Guest Editors: Elena Paoletti, Andrzej Bytnerowicz, Algirdas Augustaitis
Abstract
Of a wide variety of international forest research and monitoring networks, several networks are dedicated to the effects of climate change on forests, while the effects of anthropogenic pollutants on forests have been a major area for both monitoring and research for decades. The large amounts of data already obtained within existing monitoring programmes and large-scale international projects can be used to increase understanding of the state and potential of forest mitigation and adaptation to climate change in a polluted environment, and a major challenge now is to evaluate and integrate the presently available databases. We present a meta-database with the main goal to highlight available data and integrate the information about research and monitoring of selected European Research and Monitoring Networks (ERMNs). Depending on the selected ERMNs, the list of variables and the measurement units differ widely in the databases. As a result, activities related to the identification, evaluation and integration of the presently available databases are important for the scientific community. Furthermore, and equally important, the recognition of current knowledge gaps and future needed research is made easier. This analysis suggests that: ground-level ozone is under-investigated, although it is one of the pollutants of greatest concern to forests; in addition to CO2, long-term other greenhouse gasses (GHG) flux measurements should be carried out; there is still a need of improving links between monitoring of atmospheric changes and impacts on forests; research-oriented manipulative experiments in the forests are missing.
Keywords
Research and Monitoring Network, Meta-database, Forest, Monitoring
Introduction
Forest ecosystems play a major role in the water cycle, contain a large biodiversity, and are significant carbon (C) sinks and thus highly relevant in climate change mitigation. Forests play also a special function in the greenhouse gas (GHG) balance compared to various other natural ecosystems, because of their ability to absorb and store a significant amount of carbon dioxide (CO2) from the atmosphere ([58]). Forests depend on the surrounding site conditions and climate. In the future, they are expected to face significant pressures from climate change and air pollution as well as from intensive use through larger biomass outtake for bioenergy. The effect of climate change on forests (for example through increased frequency of extreme weather events or pathogen outbreaks) is a major area of research. An equally important task is to continuously monitor significant pollutants such as ozone (O3), nitrogen (N), acid precipitation and heavy metals. The ICP Forests, ICP Integrated Monitoring (ICPIM) and Cooperative Programme for Monitoring and Evaluation of the Long-range Transmission of Air Pollutants in Europe (EMEP) networks constitute examples of European monitoring networks which were established mainly to monitor changes in acid deposition and to correlate these with research findings. Additionally, ozone, which next to anthropogenic CO2 is a powerful GHG, is one of the main subjects of the research of several ERMNs (e.g., EMEP, ICP Forests, ICPIM, Greenhouse gas management in European land use systems (GHG-Europe), Infrastructure for Measurements of the European Carbon Cycle (IMECC) and Monitoring atmospheric composition & climate (MACC)). A majority of the current projects in Europe are funded by the European Commission (EC) or in the framework of the United Nations Economic Commission for Europe (UNECE). Apart from the European organizations, the national environmental funding agencies (ministries of environmental protection, agriculture etc.) are fundamental.
A better understanding of the integrated effects of different anthropogenic and natural stress factors on forest ecosystem functioning is essential in order to maintain, enhance and restore multiple forest ecosystem goods and services ([24]). In this context, the large amounts of data already obtained within existing monitoring programmes and large-scale international projects will be useful. A major challenge is to identify, evaluate and compare the available databases from the past research networks (like CarboEurope, NitroEurope), ongoing monitoring networks (ICP Forests, ICPIM, EMEP, LTER Europe, LIFE+ EnvEurope) and ongoing research networks (e.g., GHG-Europe, ICOS) and to harmonize these databases ([4], [8], [24]). The description and integration of the databases on the European level will help to describe the global GHG balance on the continental scale ([17]) and increase the feasibility of describing the carbon balance in Europe ([36], [6], [39], [53]).
The European Cooperation for Science and Technology (COST) programme has made a serious contribution to accomplishing the above goal. As an intergovernmental research platform established in 1971, COST is structured into particular actions, each of which has its individually defined objectives, goals and deliverables ([11]). In the domains of “Forests, their Products and Services” and “Earth System Science and Environmental Management”, two actions which focus on monitoring of air pollution and trace gas fluxes should be mentioned: FP0903 (Climate Change and Forest Mitigation and Adaptation in a Polluted Environment) and ES0804 (Advancing the Integrated Monitoring of Trace Gas Exchange between Biosphere and Atmosphere). The main objective of FP0903 is to increase the level of understanding of the present and future response of forest ecosystems to climate change in a polluted environment. Furthermore, an objective is to identify, evaluate and integrate the presently available databases, which are elaborated by the various ERMNs (Fig. 1) in order to recognize the current knowledge gaps and future emerging research needs ([12]).
Fig. 1 - The interaction between European Research and Monitoring Networks at forests and remote sites in relation to the particular pollutant and global-scale processes ([12]).
The main aim of this paper is to present a meta-database, which is the result of the collaboration and knowledge transfer between the COST Action FP0903 countries. The elaborated tables, in the form of MS Excel spreadsheets, contain information which is freely available on the various research networks’ websites, related to the effects of different stress factors such as air pollution, GHG emissions and climate change on ecosystems (in particular forest, grassland, wetland, cropland and oceans). The idea behind the meta-database is to assist in the integration of metadata from the various European networks already dealing with the effects of different stress factors on forest. While the presented meta-database includes information related to different ecosystem types (forest, grassland, wetland, cropland and oceans), this particular paper focuses especially on forest ecosystems. Despite the fact that the methodology applied in the research networks has already been described in several publications ([3], [31]), the databases have not been compared and harmonized yet.
Meta-database
Objective
The main objective of the presented meta-database is to highlight available data, compare databases and harmonize (as far as it is possible) the information from the various existing datasets. All of the selected projects are strongly concentrating on climate change and air pollution and their influence on natural ecosystems. The meta-database is freely available on the COST Action FP0903 web page (⇒ http://cost-fp0903.ipp.cnr.it/working-⇒ http://cost-fp0903.ipp.cnr.it/working-groups/wg1.html). The meta-database description includes the characteristics of the variables comprised in the Excel spreadsheets. The presented meta-database cannot replace existing databases, but exists merely to improve access to, and coordination between, the different datasets.
Selection of research and monitoring networks
The presented meta-database includes 15 ERMNs (Tab. 1). Project selection was based on the following criteria: pollutant type, ecosystem type, database accessibility and project status (past, ongoing, continual). For C (including CO2), the CarboEurope, GHG-Europe, IMECC, COCOS and ICOS have been selected. For N, the NitroEurope and ECLAIRE projects have been chosen. The ERMNs mentioned above provide open access to their databases, even after the termination of the projects. Furthermore, ongoing monitoring networks like ICP Forests, ICPIM, EMEP and LTER Europe have been included because of the different air pollutants and climatic parameters that are monitored and the long-term measurement time scale. What is equally important, the first two monitoring networks mentioned above are dedicated especially to forest ecosystems.
Tab. 1 - Basic information about the selected European Research and Monitoring Networks.
Project name | Abbreviation | Web page address | Data base web page address |
---|---|---|---|
Cooperative Programme for Monitoring and Evaluation of the Long-range Transmission of Air Pollutants in Europe | EMEP | ⇒ http://tarantula.nilu.no/projects/ccc/emepdata.html and online: ⇒ http://ebas.nilu.no/ | ⇒ http://tarantula.nilu.no/projects/ccc/emepdata.html |
Coordination Action Carbon Observation System | COCOS | ⇒ http://www.cocos-carbon.org/ | ⇒ http://dataportal.cocos-project.org/ |
Effects of Climate Change on Air Pollution Impacts and Response Strategies for European Ecosystems | ECLAIRE | ⇒ http://www.eclaire-fp7.eu/ | Not defined yet. |
European Long-Term Ecosystem Research Network |
LTER | ⇒ http://www.lter-europe.net/ | ⇒ https://secure.umweltbundesamt.at/eMORIS/jsp/common/login.jsf |
Global Earth Observation and Monitoring of the Atmosphere | GEOMON | ⇒ http://www.geomon.eu/ | ⇒ http://geomon.nilu.no/, ⇒ ftp://ftp.nilu.no/pub/GEOmon/ |
Global Terrestrial Observing System | GTOS | ⇒ http://www.fao.org/GTOS/index.html | ⇒ http://www.gosic.org/ios/GTOS_observing_system.asp |
Greenhouse gas management in European land use systems | GHG-Europe | ⇒ http://www.ghg-europe.eu/index.php | ⇒ http://www.europe-fluxdata.eu/newtcdc2/ghg-europe_home.aspx |
Infrastructure for Measurements of the European Carbon Cycle | IMECC | ⇒ http://imecc.ipsl.jussieu.fr/ | ⇒ http://www.europe-fluxdata.eu/newtcdc2/IMECC-TCDC_home.aspx |
Integrated Carbon Observation System | ICOS | ⇒ http://www.icos-infrastructure.eu/ | Not defined yet. |
Integrated non-CO2 greenhouse gas Observing Systems |
InGOS | ⇒ http://www.ingos-infrastructure.eu/ | Not defined yet. |
Integrated Project CarboEurope-IP Assessment of the European Terrestrial Carbon Balance |
CarboEurope | ⇒ http://www.carboeurope.org/ | ⇒ http://www.europe-fluxdata.eu/imecc |
International Cooperative Programme on Integrated Monitoring of Air Pollution Effects on Ecosystems | ICPIM | ⇒ http://www.environment.fi/default.asp?contentid=17110&lan=en | - |
International Cooperative Programme on Assessment and Monitoring of Air Pollution Effects on Forests |
ICP Forests | ⇒ http://icp-forests.net/ | ⇒ http://icp-forests.net/page/plots-data |
Monitoring atmospheric composition & climate | MACC | ⇒ http://www.gmes-atmosphere.eu/ | ⇒ http://www.gmes-atmosphere.eu/data/ |
The nitrogen cycle and its influence on the European greenhouse gas balance | NitroEurope | ⇒ http://www.nitroeurope.eu/ | - |
Spreadsheet descriptions
The presented meta-database is divided into 13 Excel spreadsheets. The first spreadsheet presents introductory information, including an explanation of the abbreviations used in the research. The introductory spreadsheet contains basic information about the main objective of the meta-database, the authors’ affiliations and contact information. Additionally, the source of funding is acknowledged. The subsequent spreadsheets contain general site information and then information related to the particular area of interest (meteorological parameters, soil characteristics, atmospheric chemistry, fluxes of water, C and N compounds) and particular ecosystems (forest, grassland, wetland, cropland).
The first “Projects” spreadsheet describes the selected ERMNs, related to GHG monitoring, carbon and nitrogen cycle observations, air pollution and climate change research (Tab. 1). Moreover, the URLs to the project websites and databases (also the data access details) are provided. For each project the number of measurement sites is listed. Detailed information about the coordinating institution and person in charge is also given, together with the source of funding.
The “General Information” spreadsheet contains the basic characteristics of the measurement sites in each of the selected ERMNs. First, experiment allocation details (e.g., longitude, latitude, region, country) are specified. Secondly, information about the land use (site type, area, topography, form of protection) and existing infrastructure (available roads, power supply) is presented. Finally, the basic meteorological characteristics of the measurement sites (air temperature, precipitation, wind direction and wind speed) are included.
Depending on the particular ERMN’s main objectives, the variables included in their databases are divided into several areas of interest including meteorological parameters, soil characteristics, atmospheric chemistry, fluxes of water, C and N compounds and additional information on e.g., the chemistry of runoff water, groundwater and surface water. The distribution of variables in the selected ERMNs is presented in Fig. 2. In the first two networks (CarboEurope and NitroEurope) the highest number of variables is dedicated to meteorology and soil characteristics. A specific characteristic of these two ERMNs is also the inclusion of flux measurements of C and N compounds. The GHG-Europe and IMECC networks, as a continuation of the above projects, are concentrated on the GHG (CO2, N2O and CH4) balance estimation of terrestrial ecosystems in Europe with respect to natural and anthropogenic drivers like climate and land use changes ([26], [34]). In the area of atmospheric chemistry, the EMEP network includes the highest number of variables (22 in the meta-database). Furthermore, the meta-database includes 13, 17 and 14 atmospheric chemistry variables for ICP Forests, ICPIM and MACC, respectively.
Fig. 2 - The number of variables available in the meta-database according to ERMN and area of interest.
The available variables in the selected ERMNs’ databases are presented differently on the networks’ home web sites. Variables based on direct measurements can be downloaded as site-specific values in tabular form (like in the CarboEurope network). In cases where satellites and atmospheric models are important sources of data, variables are available as regional maps and plots, differing in spatial and temporal scales ([40]).
The detailed description of the meteorological variables is divided into thematic groups. The standard variables, including precipitation, air temperature, air pressure, relative humidity, wind speed and wind direction, are present in most of the selected ERMNs. Furthermore, the radiation is measured, but the particular detailed radiation components (incoming/outgoing, shortwave/ longwave radiation, global/diffuse/net radiation, photosynthetically active radiation, albedo) are dependent on the specific programme. Additionally, in ICPIM the variables related to the beginning and length of the vegetation period (VP), precipitation during VP or beginning and length of the snow cover period are described ([33]). For some of the selected ERMNs containing micrometeorological flux data (CarboEurope, NitroEurope, GHG-Europe, IMECC, ICPIM, InGOS), the meta-database provides information on the flux measurement system and a list of micrometeorological variables related to atmospheric turbulence (like momentum flux and stability).
The most common standard for basic soil description is the Food and Agriculture Organization of the United Nations (FAO) soil classification which is applied in 6 of the selected ERMNs. The soil texture is described by the % content of sand, sandy loam, and clay loam according to the USDA Textural Triangle. In the majority of the selected ERMNs soil characteristics such as soil depth, root depth, and pH are given. Soil chemical variables included are total contents of Corg, N, S, P, Ca, carbonate content, exchangeable contents of Ca2+, Mg2+, K+, Na+ and dissolved concentrations of Na, K, Ca, Mg, Cl, SO4-S, SiO2 and heavy metals. The soil physical properties are characterized by soil water content, conductivity, field capacity, wilting point, and moisture. The measurement units are dependent on the ERMN methodology.
The “Atmospheric chemistry” spreadsheet is divided into two parts: characteristics of air and of precipitation. Gas concentrations, such as O3, CO2, H2O, NH3, NH4, HNO3, NO, NO2, and SO2, form the most important group of variables in part one. Additionally, atmospheric aerosols, radioactive elements (MACC project - Barium 40, Caesium 137, Iodine 131, Krypton 85, Ruthenium 103 etc. in Bq·m-3 unit) and tracers are measured. Heavy metals such as Cd, Pb, Cu, Zn, Cr, and Ni, As, and Persistent Organic Pollutants (POPs) are measured in both atmospheric air and precipitation. In part two of the “Atmospheric chemistry” spreadsheet throughfall/stemflow and wet/dry deposition (for NH4+, SO42-, NO3-, Ca, Mg, Na, K, Cl, and Hg) are included.
The data on the atmosphere-ecosystem exchange measurements are listed in the “Flux” spreadsheet. The fluxes that can be distinguished are terrestrial ecosystem fluxes, soil fluxes, and other fluxes. In the first group the CO2, CO, H2O, CH4, NH3, NO, N2O, NO2, and O3 fluxes are measured, mostly by using the eddy covariance technique as specified in the “Meteorology Measurement system” spreadsheet. The CO2 flux and autotrophic/heterotrophic respiration measurements, mostly using chamber techniques, are the most common soil variables. At the ecosystem scale, many ERMNs report Gross Primary Production (GPP), Net Primary Production (NPP), and Net Ecosystem Exchange (NEE), based on the CO2 flux measurements.
In four ERMNs (ICPIM, MACC, COCOS and GTOS), certain additional environmental information can be found related to the lake/runoff/groundwater chemistry, bird inventory, hydrobiology and glaciers. The given variables are characterized partly by a text description, partly by physical units ([33], [40], [9], [27]).
The meta-database presents three separate spreadsheets taking different natural ecosystem types (forest, grassland/wetland, and cropland) into consideration. Although each of the mentioned types has numerous specific variables related to it, for instance, tree parameters, grassland cutting or grazing, or cropland fertilizations, there are also variables which are common for all types. In the spreadsheets general information like site history, plant species composition, vegetation height or vegetation indexes are given. Also, particular information is given such as the single leaf/needle description (stomatal conductance, interception, wetness, LAI), through the particular tree characteristics (diameter at breast height, height, volume, age, social class), ending with the tree stand information (number of trees per unit area, phenological observations). Especially the ICP Forests database contains information about periodic events such as cuttings, fertilization, fruiting, flowering, leaf/needle damage with e.g., symptoms, causal factor, and age of damage.
ICP Forests is the project with the highest number of variables related to forest tree stand characteristics in the meta-database (Fig. 3). CarboEurope has the database with most terrestrial ecosystem-specific variables. Also, variables such as proportion of dead/green leaves and root depth are measured. Furthermore, the CarboEurope database includes factors related to management such as vegetation height after cutting as well as LAI after cutting, mineral and organic fertilization (form, amount and time period of applied fertilizers) and continuous or rotational grazing and number of animal. In CarboEurope (28 variables) and NitroEurope (19 variables) most cropland ecosystems were included. NitroEurope additionally provides information about soil profile characteristics, e.g., number of layers and their depth and biomass C and N concentrations in residues, grain and straw ([5], [45]).
Discussion
According to FAO ([21]), forests cover about 30% of the Earth’s land surface, which is 3953 million ha. In conversion to area per capita this represents 0.62 ha. As forests constitute such a substantial part of the Earth’s surface, it seems reasonable that an overweight of research studies are conducted in forests. According to the climatic scenarios elaborated by the Intergovernmental Panel on Climate Change, the emission of anthropogenic pollutants such as CO2, CH4, NO, N2O, O3 etc. will rise significantly until the year 2100 ([35]). Therefore, the reaction of forest ecosystems to these atmospheric changes is a crucial issue.
Scientific communities are interested in the mutual integration of existing international research knowledge and databases, with respect to measurement methodology, database availability and QA/QC procedures. Although each of the experimental sites has its own technical and scientific requirements, the basis for research should be as far as possible equal for all sites continentally/globally, which will enable up-scaling and comparison of results. The ICOS project constitutes an example of such a network focusing on “...building a network of standardized, long-term, high precision integrated monitoring of atmospheric greenhouse gas concentrations of CO2, CH4, CO and radiocarbon-CO2 to quantify the fossil fuel component, ecosystem fluxes of CO2, H2O, and heat together with ecosystem variables...” ([30]). Furthermore, the ICOS project, which at present is in the preparatory phase, assumes that measurements will be conducted for at least 30 years. This long-term perspective is especially important to environmental research, as the changes in natural ecosystems, perhaps especially in forests, can be best described in decade-long timespans. Examples of long-term ERMNs which have been operating since the 1970s and 80s include ICP Forests, ICPIM and EMEP. The ongoing monitoring networks mentioned above are at present an important source of information about the natural ecosystem state, health condition and response reaction to observed climate change. By application of state-of-the-art measurement techniques such as eddy covariance, relaxed eddy accumulation, and automated chambers in various research projects and networks, the estimation of the main GHG fluxes on various spatial and temporal scales is possible. Although flux variables are reported in the meta-database, their presence is related mainly to the short-term projects (CarboEurope, NitroEurope, GHG-Europe and IMECC). Taking into consideration the above statement, it is important to enhance the ongoing monitoring networks by introducing GHG flux measurements as a standard.
The networks operate on different spatial scales. All of the selected ERMNs are significantly diversified in reference to which pollutants they treat (Fig. 4). Carbon dioxide and N are most researched and are therefore represented by the highest number of variables. In contrast, ozone is less investigated in the selected ERMNs.
Ozone
Ozone is an air pollutant with harmful effects on both sensitive plants and animals’ respiratory systems. Additionally, this compound is a powerful GHG. Due to increased emissions of precursor compounds, the present near-surface O3 concentrations are substantially higher than the pre-industrial levels. Modern day annual average background ozone concentrations over the mid-latitudes of the northern hemisphere range within approximately 20-45 ppb ([59]), which is a 100-350% increase relative to the pre-industrial age (~10 ppb). According to the climatic scenarios this trend of enhanced ozone will increase in the years to come. It has been estimated that the current O3 levels decrease the biomass growth of northern hemisphere forests by 7% ([61]) and that half of the world’s forests may be exposed to damaging concentrations of > 60 ppb by 2100 ([25]). The negative potential impact of ozone on trees, especially their growth, leaf/needle visible injury, productivity or biodiversity, is well documented ([42], [37], [49]). Translating this potential into impacts on world forests is still a major challenge ([54]). In addition, the present standards for protection are based on the amount of ozone in the air (exposure), while the impacts arise from the amount of ozone that enters through the stomata (flux). This is why exposure and impacts appear to be decoupled in regions where other stressors limit stomatal aperture, like water stress in the Mediterranean region ([48]).
Ozone concentration in ambient air is monitored in EMEP, ICP Forests, ICPIM, GHG-Europe, IMECC and MACC. In EMEP and ICP Forests, measurements of ozone are related to its harmful influence on the forest stand, by calculating the AOT40 index ([20], [32]). AOT40 is the sum of the positive differences between the hourly mean ozone concentration and the threshold of 40 ppb, during daylight hours in a fixed growing season ([57]). The database of EMEP, which represents the most extensive O3 monitoring station network of these ERMNs, contains hourly O3 concentration data from 130 sites in 27 countries (for 2009 - [56]). Although measurement of O3 flux is useful, the direct measurement at canopy level is more difficult than that of O3 concentrations ([7]), and for this reason these data are only available from GHG-Europe and IMECC.
Carbon dioxide
The atmospheric concentration of CO2 grew from 280 ppm in the industrial revolution period to 385 ppm in 2010 (+38%), and rose about 1.5 ppm per year in the last decade ([52], [14]). This increase is related to CO2 emissions induced by fossil fuel combustion, utilization of natural gas/oil products, increasing energy consumption and land-use changes. Of all emitted CO2, only about half is returned to the atmosphere, due to sequestration of C in oceans and terrestrial ecosystems. Of the terrestrial ecosystems, forest ecosystems are most important with respect to CO2 assimilation from the atmosphere. Forests, including forest soils, are thus dominating the global C pools of terrestrial systems. Tropical forests are the most significant C pools, with boreal and middle latitude forests following. The global C balance in forest ecosystems has been widely described in the literature ([16], [50], [14], [39]).
In general, forest C, as an element, is present in the meta-database on several levels. The total organic C concentration in forest soils, content in roots and foliage, and the global C concentration in the underground biomass per unit area are variables included. The percentage C content in stem wood, foliage, particular shoots and leaves is measured on a single tree level (CarboEurope, NitroEurope, GHG-Europe, ICP Forests and IMECC). Upscaling the research to the stand level, the interest shifts from C as an element to CO2. The main area of interest is focused on measurements of forest productivity (Gross Ecosystem Production) and the net CO2 exchange rate between forest and atmosphere (Net Ecosystem Exchange), divided into tree biomass and the soil.
Nitrogen
At present, the main sources of anthropogenic nitrogen (N) are related to N fertilization and N fixed during fossil fuel combustion ([41]). Modern day annual estimates of nitrogen deposition from the atmosphere over central Europe range within approximately 10-20 kg N ha-1 year-1 ([41], [29]). Nitrogen as an element is equally valuable to plants and microorganisms as it influences their growth and stimulates their productivity. Furthermore, the level of N affects the ecosystem species composition and biodiversity ([60]). Additionally, in the situation where nitrogen is abundant, N saturation can lead to NO3- leaching and eutrophication ([1], [28], [22]). The N cycle is inseparably related to the C cycle and it also stimulates plants’ photosynthesis and respiration processes, which can affect the forests’ ability to sequester CO2 in tree biomass ([44], [13], [53]).
Nitrogen variables in the ERMNs have such points of reference as the soil level, below/aboveground biomass and single tree/ leaves/needles. In NitroEurope net N mineralization, nitrification, denitrification, NH4+ and NO3- concentrations, microbial biomass and NO3- leaching are measured. Nitrogen is included in measurements in air (NH3, NH4, HNO3, NO, NO2), in precipitation (total N, NH4+, NO3-) and in soil solutions and groundwater (NH4+ and NO3- concentrations).
Acidity
Sulphur dioxide (SO2) and nitrogen dioxide (NO2) are emitted to the atmosphere largely from anthropogenic sources such as fossil fuel combustion, transport and industry intensive development. Additionally, the variations in the concentrations of the NO2 pollutant in soil, ground water, lakes or rivers are related to the intensive agricultural production. The total SO2 emission shows a decreasing trend since 1980; in Europe and North America -74% from 1990 to 2008 ([18], [55]). For 1990, the global SO2 emission was estimated at about 120 Tg. As a result of the strict controls that have been implemented in Organization for Economic Co-operation and Development (OECD) countries and the economic restructuring in Central and Eastern Europe after 1990, the SO2 emissions declined by about 20% to the year 2000 ([10]). With regard to the global NO2 emissions, a decreasing trend can be observed in Europe (because of the strict emission controls in western Europe) in contrast to Asia where the emissions have increased rapidly since the 1970s ([2]).
The removal of SO2 and NO2 compounds from the atmosphere to the natural ecosystems stems from both wet and dry deposition processes. The mechanism of acid precipitation formation is based on the oxidation of SOx and NOx in the atmosphere to acids like H2SO4 and HNO3, which are then deposited in precipitation ([38]). Deposition of acidic pollutants has negative effects on forests, causing damage (discoloration, defoliation, mortality increase etc.) and decreasing forest biodiversity and productivity ([19]). Apart from the effects mentioned above, sulphate and inorganic N deposition significantly influences soil properties such as pH, base saturation or cation exchange capacity ([15]).
The variables related to acidification are included in the meta-database in several spreadsheets, depending on the area of interest (atmosphere, soil etc.). In relation to the measurements of the atmospheric air dry deposition, the following variables can be distinguished: NH4+, SO42-, NO3-, Ca, Mg, Na, K, Cl, and Hg (EMEP, ICP Forests, ICPIM, MACC, GEOMON). Measurements also include stemflow and throughfall deposition (CarboEurope, GHG-Europe, IMECC, ICP Forests and ICPIM). The research into soil acidity includes the measurement of NH4+ and NO3- concentrations in soil solution and groundwater, dissolved SO42- and heavy metals, pH, base cations and alkalinity ([32], [33], [45]).
Heavy metals
Heavy metals such as cadmium (Cd), lead (Pb), copper (Cu), zinc (Zn), chromium (Cr), nickel (Ni) and iron (Fe) are emitted to the atmosphere from the anthropogenic sources such as: coal combustion in power plants, industry (iron, steel cement production) and gasoline combustion (especially for Pb). According to Pacyna et al. ([47]), there has been a continuous reduction of heavy metal emissions in Europe during the last 40 years. Furthermore, there is a potential for further reduction of these emissions until the year 2010 by up to about 40% for Cd, Cr, and Ni and about 57% for Pb ([47], [20]).
The heavy metals mentioned above have negative effects on forest ecosystems, leading to different types of plant damage, like leaf discoloration, increasing tree mortality or significant decreases in tree growth and biodiversity ([43], [23]). Additionally, mercury (Hg) which is also an important trace element emitted to the atmosphere, can influence not only the environment but also human health ([46]).
Depending on the forest type (coniferous, broadleaves, mixed forests), species, plant architecture (such as canopy form and visibility), and stand density, the amount of heavy metal deposition can vary. The heavy metals are mostly found in forest soils. The pH constitutes the key factor which determines heavy metal availability and toxicity to plants in this particular environment. Any increase in soil acidity may result in heavy metal leaching from soil ([43]). Moreover, the trees’ reactions to high concentrations of heavy metals can leave them vulnerable to more and more damage caused by different species of fungi and insects ([51]). The following heavy metal elements are measured in the ERMNs: cadmium (Cd), lead (Pb), copper (Cu), zinc (Zn), chromium (Cr), nickel (Ni) and iron (Fe). Heavy metal concentrations are measured in EMEP, LTER, ICPIM and ICP Forests.
Conclusions
In the last years, the investigation and understanding of the state and potential of forest mitigation and adaptation to climate change in a polluted environment has increased significantly. Much of such research is based on a wide variety of environmental measurements carried out in the framework of various global and continental research networks. In these networks the effects of various stress factors, such as air pollution, GHG emissions and climate change on natural ecosystems have been quantified in different research projects. This paper describes a meta-database which has been created based on the major research and monitoring networks in Europe.
The main anthropogenic pollutants like CO2, N pollutants, and heavy metals are covered fairly well by the high number of variables in the presented meta-database. However, in the case of ozone, it is ambient ozone concentrations that are often investigated, whereas fluxes of ozone which are more relevant to negative impacts on vegetation need more attention. However, the present status of ozone in the different monitoring and research networks will be improved by forthcoming projects such as “Effects of Climate Change on Air Pollution Impacts and Response Strategies for European Ecosystems” (ECLAIRE). In general, there is still a need of improving links between monitoring of atmospheric changes and impacts on forests because these two fundamental activities are carried out separately in independent ERMNs. Research- oriented manipulative experiments in the forests are limited, although they would significantly contribute to the understanding of real-world forest adaptation to the ongoing atmospheric changes.
As the climatic scenarios presented by the IPCC are not optimistic, understanding of the interactions between natural ecosystems and the atmosphere is crucial. This goal could be better attained if information about different international projects and research programmes for various ecosystems (forest, grassland, wetland, etc.), subjects (meteorology, chemistry, etc.), and ecosystem compartments (atmosphere, soil, etc.) was integrated. It is equally important to standardize the measurements, apply appropriate QA/QC procedures and improve access to and use of the different databases. By unification of the applied methodology, upscaling and comparison of the results will be possible. The presented meta-database is a first step in this direction.
Of major importance to the scientific community is the identification of current knowledge gaps and emerging research areas which are present in research projects and networks. On the basis of information included in the presented meta-database, the differences in the selected ERMNs are visible, especially in the presence of particular variables in the databases and their measurement units. There is a crucial need for harmonization and integration of research and monitoring projects and networks. One of the future possibilities for harmonization is the establishment of comprehensive and well equipped research sites, also known as Supersites ([24]), which will be a source of detailed information about the examined ecosystem. The database established on the basis of a Supersite network will optimize the sites’ geographical distribution and assure coverage of the most relevant ecosystems in Europe.
Acknowledgments
We acknowledge the COST Action FP0903 “Climate Change and Forest Mitigation and Adaptation in a Polluted Environment”. The meta-database was elaborated in the frame of the Short Term Scientific Mission (STSM) fellowship with the reference number COST-STSM-ECOST-STSM- FP0903-011011-010860 financed by the COST Action FP0903. The STSM title is: “Establishment of a European forest monitoring meta-database”. We wish to thank all scientists and experts contributing to the discussions.
References
CrossRef | Gscholar
CrossRef | Gscholar
CrossRef | Gscholar
CrossRef | Gscholar
Gscholar
Gscholar
Gscholar
CrossRef | Gscholar
Gscholar
CrossRef | Gscholar
CrossRef | Gscholar
Gscholar
Gscholar
Gscholar
CrossRef | Gscholar
CrossRef | Gscholar
Gscholar
Authors’ Info
Authors’ Affiliation
J Olejnik
M Urbaniak
Meteorology Department, Poznan University of Life Sciences, Piatkowska 94, 60-649 Poznan (Poland)
Norwegian Forest and Landscape Institute, P.O. Box 115, N-1431 s (Norway)
IVL Swedish Environmental Research Institute, Box 210 60, SE-100 31 Stockholm (Sweden)
Alterra, Wageningen University and Research Centre, P.O. Box 47, 6700 AA Wageningen (The Netherlands)
Department of Aquatic Sciences and Assessment, Swedish University of Agricultural Sciences, P.O. Box 7050, SE-750 07 Uppsala (Sweden)
Finnish Meteorological Institute, Climate Change Research, P.O. Box 503, FI-00101 Helsinki (Finland)
PCC of ICP Forests, Institute for World Forestry, Johann Heinrich von Thünen-Institute (vTI), Leuschnerstrasse 91, D-21031 Hamburg (Germany)
Istituto per la Protezione delle Piante - CNR, v. Madonna del Piano 10, I-50019 Sesto Fiorentino, FI (Italy)
Corresponding author
Paper Info
Citation
Danielewska A, Clarke N, Olejnik J, Hansen K, Vries W, Lundin L, Tuovinen J, Fischer R, Urbaniak M, Paoletti E (2013). A meta-database comparison from various European Research and Monitoring Networks dedicated to forest sites. iForest 6: 1-9. - doi: 10.3832/ifor0751-006
Academic Editor
Alberto Santini
Paper history
Received: Aug 27, 2012
Accepted: Nov 22, 2012
First online: Jan 14, 2013
Publication Date: Feb 05, 2013
Publication Time: 1.77 months
Copyright Information
© SISEF - The Italian Society of Silviculture and Forest Ecology 2013
Open Access
This article is distributed under the terms of the Creative Commons Attribution-Non Commercial 4.0 International (https://creativecommons.org/licenses/by-nc/4.0/), which permits unrestricted use, distribution, and reproduction in any medium, provided you give appropriate credit to the original author(s) and the source, provide a link to the Creative Commons license, and indicate if changes were made.
Web Metrics
Breakdown by View Type
Article Usage
Total Article Views: 66869
(from publication date up to now)
Breakdown by View Type
HTML Page Views: 56986
Abstract Page Views: 3628
PDF Downloads: 4336
Citation/Reference Downloads: 94
XML Downloads: 1825
Web Metrics
Days since publication: 4212
Overall contacts: 66869
Avg. contacts per week: 111.13
Article Citations
Article citations are based on data periodically collected from the Clarivate Web of Science web site
(last update: Feb 2023)
Total number of cites (since 2013): 11
Average cites per year: 1.00
Publication Metrics
by Dimensions ©
Articles citing this article
List of the papers citing this article based on CrossRef Cited-by.
Related Contents
iForest Similar Articles
Review Papers
Monitoring the effects of air pollution on forest condition in Europe: is crown defoliation an adequate indicator?
vol. 3, pp. 86-88 (online: 15 July 2010)
Short Communications
QA/QC activities and ecological monitoring in the Acid Deposition Monitoring Network in East Asia (EANET)
vol. 2, pp. 26-29 (online: 21 January 2009)
Editorials
COST Action FP0903: “Research, monitoring and modelling in the study of climate change and air pollution impacts on forest ecosystems”
vol. 4, pp. 160-161 (online: 11 August 2011)
Research Articles
Long-term monitoring of air pollution effects on selected forest ecosystems in the Bucegi-Piatra Craiului and Retezat Mountains, southern Carpathians (Romania)
vol. 4, pp. 49-60 (online: 05 April 2011)
Commentaries & Perspectives
Availability, accessibility, quality and comparability of monitoring data for European forests for use in air pollution and climate change science
vol. 4, pp. 162-166 (online: 11 August 2011)
Editorials
Adaptation of forest ecosystems to air pollution and climate change: a global assessment on research priorities
vol. 4, pp. 44-48 (online: 05 April 2011)
Commentaries & Perspectives
Clean air policy under the UNECE Convention on long-range transboundary air pollution: how are monitoring results “translated” to policy action
vol. 2, pp. 49-50 (online: 21 January 2009)
Research Articles
Genetic monitoring in forests - early warning and controlling system for ecosystemic changes
vol. 4, pp. 77-81 (online: 05 April 2011)
Research Articles
First results of a nation-wide systematic forest condition survey in Turkey
vol. 4, pp. 145-149 (online: 01 June 2011)
Short Communications
Ozone flux modelling for risk assessment: status and research needs
vol. 2, pp. 34-37 (online: 21 January 2009)
iForest Database Search
Search By Author
- A Danielewska
- N Clarke
- J Olejnik
- K Hansen
- W Vries
- L Lundin
- J Tuovinen
- R Fischer
- M Urbaniak
- E Paoletti
Search By Keyword
Google Scholar Search
Citing Articles
Search By Author
- A Danielewska
- N Clarke
- J Olejnik
- K Hansen
- W Vries
- L Lundin
- J Tuovinen
- R Fischer
- M Urbaniak
- E Paoletti
Search By Keywords
PubMed Search
Search By Author
- A Danielewska
- N Clarke
- J Olejnik
- K Hansen
- W Vries
- L Lundin
- J Tuovinen
- R Fischer
- M Urbaniak
- E Paoletti
Search By Keyword