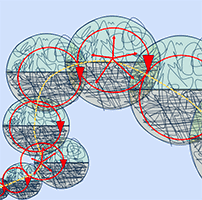
The soil-conscious forestry and the forbidden apple (§)
iForest - Biogeosciences and Forestry, Volume 17, Issue 4, Pages 252-268 (2024)
doi: https://doi.org/10.3832/ifor4584-017
Published: Aug 16, 2024 - Copyright © 2024 SISEF
Review Papers
(§): CORRIGENDUM: An errata corrige in Figure 9 of this paper has been published on October 13, 2024. (see 10.3832/ifor4584-017-bis).
Abstract
In this article, we propose a silvicultural approach that takes soil health into account. Using a biblical metaphor, the first chapter highlights the forest’s critical role in the planet’s future, emphasizing that forest biodiversity is essential for sustaining a planet inhabited by complex life forms like humans. The second chapter focuses to the soil, explaining how the biological dynamics of the soil are interconnected with the forest cycle, demonstrating that vegetation and soil form a continuous, co-evolving system. The third chapter explores the possibility of extending the forest cycle to restore soil functionality, illustrating how the recycling of the forest’s biological past facilitates the emergence of a new, evolving soil-vegetation system. Examples of forest management practices in France, Italy, and Switzerland are presented, including graphs and mathematical models depicting the evolution of organic carbon in the soil and the distribution of tree stems by diameter, age, and stature. The description of uneven-aged Swiss forests, featuring fir and beech, is sourced from the Helvetica Pro-Silva website.
Keywords
Anthropocene, Adam and Eve, Forest Soil, Humus, Soil-conscious Forestry, Envers Forest, Forest Dynamic, Old-growth Forest
Introduction
The forbidden apple
Well-known biblical recommendation: “You can do whatever you want, planet Earth is yours, plants, animals, mountains, sea, clouds, everything. That apple tree you see in the middle of the meadow, only that one, behold, don’t eat its apples.” (Fig. 1).
Fig. 1 - Adam and Eve in the Trauttmansdorff Castle Park, Merano, Italy. It is possible to conceive the primary forests that remain, like other biodiversity-rich environments on our planet, as “forbidden apples” for humans. In the sense of being able to understand that it is better “not to eat them”, as they are really essential for us, we humans as a dependent part of a living planet.
Some 6-7 million years ago, the lineage we share with chimpanzees bifurcated, generating a branch of hominids. They differed from their ancestors because they preferred to stand upright position, on two feet instead of four ([20], [21]). Until then, and for a few million years more, evolution depended on environmental conditions, first astronomical, chemical, physical, then increasingly biological. A list of incomplete, scientifically shared in the essential ecological synthetic lines, that explains evolution and supports the content and conclusions of this article, is available in Appendix 1 (Supplementary material).
Little by little, the hominids changed the cards on the table: for ten thousand years now, but above all and definitely in the last century, evolution depends directly on the needs of this new species of living beings that we are.
We know that Hominids were an integral part of the forest in the Paleolithic and also in a large part of the Neolithic. It is contravening a hypothetical divine warning that Homo sapiens entered the Anthropocene. Only in the last decades of human history, the consideration of non-log products, under the names of ecosystem services or biodiversity, indicates a forest exploitation with a more conscious view of the biological value of this ecosystem.
The causes of human impact on our planet are summarized in Appendix 2 (Supplementary material).
The forests of the world
State of the art
The surface of the planet (51 billion ha = 51 × 109 ha) consists of 71% oceans and 29% of emerged lands (14.9 × 109 ha). Of these emerged lands, 71% (10.6 × 109 ha) is habitable (it is not bare rock, desert or ice). Ten thousand years ago, forests covered 57% (6 × 109 ha) of habitable land; today 38% (~4.02 × 109 ha), half (2 × 109 ha) are almost intact and one third (1.33 × 109 ha) are in the state of primary forest, i.e., naturally regenerating with primary species ([38]).
In 1700, the area devoted to agriculture occupied 3% of the habitable land, and grazing 6%; in 2020, these values became 15% and 31%, respectively; the part occupied by ungrazed prairies and shrublands reaches 14%.
Gradually, cultivation, grazing and urbanization have occupied 1/3 of the forest area ([40]). As human populations living in forests are relatively disarmed and declining ([137]), and urban populations continue to grow and require resources and food, the replacement process is called for to continue to meet the needs of humans and domestic animals ([41]).
Can humans live far from Earth? Let’s try to argue in the affirmative. It would be enough to take with us greenhouses full of plants and animals that will produce food for us in space, when we set out in search of other worlds as in science fiction movies. Is this journey in the company of a small productive part of the planet really possible? This topic is covered in Appendix 3 (Supplementary material).
What if “forest ecosystem services” did not exist? This is also a topic that goes beyond the heart of our article. If it were true, however, we would have to review our mentality. The in-depth analysis is proposed in Appendix 4 (Supplementary material).
Indisputable facts
(i) For reasons essentially related to food and the contingent needs of humanity, humans have taken over half of the area that once belonged to the forest to make cultivation fields and cities ([40]). About 4 billion hectares of forest remain on the planet.
(ii) A quarter (about 1 billion ha) of the forest surface is still in the natural state of “old-growth forest”, i.e., a forest that has reached a very advanced age without having undergone major disturbances by human activities ([67], [50], [2], [124]), and 20% of this can be classified as “intact forest landscape”, a forest area of at least 500 km2 without remote human action ([96], [97]).
(iii) About half of the planet’s biodiversity is found in tropical forests. As their surface area has decreased, so has biodiversity. Overall estimated biodiversity losses outside forests in the period between 1970 and 2022 range from 18% to 94% depending on the region of the planet ([3], [63]).
(iv) Human needs for wood will increase by 37% between now and 2050 ([41]).
(v) In 2021 we lost another 3.75 million primary forests ([125]).
If we continue at this rate, we will lose all primary forests. Is that a big deal? If all forests were managed according to the rules of the latest close-to-nature ([5]), would this be a problem for the planet and for us humans? Why would soil-conscious forestry be more suitable for forest management than nature-friendly forestry? What does soil do that is important in practice that is not covered by close-to-nature silviculture?
The questions we are trying to answer
The article revolves around the concept of forest soil and is divided in three chapters: Soil, Soil Conscious Forestry and Conclusion. Following the model adopted in the introduction, the insights are placed in the Supplementary material to streamline the article. Below is the list of questions we addressed.
(i) Soil: what is the dying process? Does the soil have an ecosystem history? What is humipedon? Does the soil carbon storage and quality change as trees age?
(ii) How do forest manager identify the key elements of a forest soil ecosystem? In how much time does a forest renew itself? How can exotic species be managed? How to tackle climate change? To what extent are coppices, urban parks and tree plantations forests? How much biomass can we remove from forests? Are the last primary forests really important for humanity?
(iii) What is the point of view of two forest managers?
The soil and the forest
To imagine the underground world of a forest, we suggest that you turn the trees upside down in your mind to give them two tips, one outside and one inside the ground. The new double forest is closer to reality than you might think. Physically it is not symmetrical; underfoot the forest is more complex than above, because the soil matrix is denser, richer and different from that of the air. Considering that there are algae and cyanobacteria active even in the first 3-5 cm of soil, we can say that the luminous part of the photosynthetic process occurs above this depth and to a large extent outside the soil.
It is possible to see the soil complexity in Appendix 5 (Supplementary materials).
The future of a living forest is preceded by its death in the soil
Without soil water, photosynthesis in vascular plants would also stop; the first step in the process is the splitting of water molecules to recover the energy from the released electron. The products of photosynthesis are transported everywhere, including in the roots and the soil. To bring light to the solar panel-like leaves, the trees must grow above the other plants, and to do so they also need the mineral elements that are present in the soil. These mineral elements are not only those of the parent rock, but also those of the organic matter continuously recycled by the soil microorganisms. We are talking about wood, leaves, bark, flowers, bracts, cones, etc. or exudates inside and outside the soil, and also animal’s excrements, dead animals or parts of them that live out and in the soil. These are hundreds of grams per m2 per year that continuously “feed” the microorganisms and animals or fungi in the soil. In turn, these organisms die and are recycled, releasing the mineral elements that make up their tissues and molecules. In simple words, the soil is the belly of the forest, a belly fed by the living system of which it is a part.
Much of the forest is therefore under the forester’s feet. Water, energy, nutrients, biodiversity, forest production and renewal all depend on the soil. We know that it is useful to distinguish at least three layers (herbaceous, shrubby and arboreal) to be able to understand the dynamics of the vegetation. From a functional point of view, to better study its complexity, the soil can also be divided vertically into three sections ([134]):
(i) the Humipedon, with the organic horizons OL, OF, OH, and the surface organo-mineral A horizons. Much of the soil’s biodiversity lives these horizons. These organisms evolve with the soil and are the key to understanding the litter recycling processes and the relationships between plants and soil;
(ii) the Copedon, in more developed soils, composed of newly formed mineral horizons E and B. In this layer, the processes of formation of new clays take place which migrate biologically and not in the profile and which can be related to the water cycle in the soil;
(iii) the Lithopedon including the horizon of fragmented rock (C) and the superficial part of the rock more or less altered but still in place (R).
A good knowledge of the soil is essential for forest management. Particular attention should be paid to the humipedon, whose relationships with the evolution of the forest are illustrated below.
Either it is alive, in which case it is really “soil”, or it remains “rock”
There are no living organisms on the moon. For this reason, soil like that of our planet has not formed on this natural satellite. Constantly exposed to the disaggregating action of the physical and chemical agents of space, the lunar rock (identical in composition to that of the earth’s crust) has and continues to alter on the surface, remaining inert. On Earth, instead, rock has been transformed into soil by living beings. You only have to imagine the process to realize the importance of this phenomenon. Ecosystems emerge from spatial dust (it is possible that life originated from carbon molecules coming from space), first elementary and without walls, and then enclosed in even larger systems (single cell, groups of cells, etc.), composed of systems, and composing systems of systems. On Fig. 2the animals do not appear, because the most important and numerous of them are barely visible microarthropods ([56]) or the invisible ones such as microorganisms ([8]).
Fig. 2 - Soil has a history. On our planet the soil is undergoing an evolution that follows that of the rest of living beings. Crusto humipedons ([133]) are thin and cover a series of very young soils ranging from biofilms to desert crusts. They are formed by microorganisms or very small plants, fungi and animals. In the forest they are very common on rock outcrops or on tree trunks. Bryo are humipedons of greater thicknesses made of mosses and lichens, and pedofauna. Rhizo are humipedons that are formed by the strong action of the roots of herbaceous or shrubby plants, in cooperation with pedofauna. Along a gradient that runs from the high mountains to the plains, Mor and Moder or Tangel and Amphi humipedons form, respectively on acidic or basic parent rock respectively. In milder, lowland environments the dominant humipedon is the Mull. Moving through the figure from left to right we travel through the time of natural evolution and soil formation.
Regarding the functioning of the soil, there is a fundamental principle. Discovered in the agricultural field, it can be stated with this phrase: “if you want to feed the plants you have to feed the soil organisms”. For an illustrated study on this topic, please read Appendix 6 in the Supplementary material.
Bringing the discussion back to a practical and forestry field, just to take an example of this biological complexity, the role of bacteria in the digestive tract of earthworms, with the “priming effect” played by intestinal mucus, has been studied by several researchers ([11], [64], [44], [12], [61], [69]). There is also the role played by the mucus secreted outside ([10]). Recently, Guhra et al. ([47]) concluded that the specific adsorption of earthworm mucus constituents to soil minerals leads to the formation of mucus-mineral associations. The worms are not essential to the action of the bacteria, they just stimulate it, as do the root exudates, and the clays play a role through their developed surface. Without considering the biological aspects, Bramble et al. ([18]) highlight a contrast between 1:1 clays like kaolinite, with a low developed surface area, and 2:1 clays like hillite or montmorillonite (“swelling” clays) which offer a contact surface (and therefore a habitat) much more important for soil bacteria. Earthworms also serve to promote contact between bacteria and clays and play a role in their transport because most bacteria need a vector to move and find their food ([65], [66]), unless it arrives via percolation for example or diffusion.
The relationship between the living and dead parts of an ecosystem in the process of natural evolution is also very curious, and involves the soil activity ([135]). Much of this underground world still escapes us, but it exists and we need to know it, even if we are still at the first steps in using this biodiversity. Recently, there has been a large debate about the topsoil microbiome ([7]). For example, we know that climate change and human activities are causing a crisis in cork production, and that this problem is triggered by changes detected at the rhizospheric microbiome level ([73]). We must be able to prevent this trigger, to continue to grow cork oaks. We also know that soil carbon is found in aggregates and that microorganisms form these aggregates ([55]). The large stable aggregates are in the Mulls, and disappear in the Moders. In the latter, organic carbonium is found in unprocessed or poorly transformed plant remains. Here we need to be able to recognize these humus systems in the field. Everyone now agrees that we need to know the microbiome to manage the forest well, a microbiome that changes in and out of the soil with the number of tree species involved ([121]).
The humipedon and the forest soil functioning
The evolution of the soil is linked to the evolution of the living organisms that inhabit it. To understand this phenomenon, it is necessary to carefully analyze the humipedon. In it, living beings build two types of structures that are extremely important for the functioning of the soil: the organic OH horizon and the organo-mineral A horizon. They are functionally related structures, as when one increases, the other decreases, and vice versa. The OH thickness increases under difficult environmental conditions. Essentially these are low temperatures, drought, frost, or harmful/polluted/asphyxiated substrate for living organisms. The A horizon, on the other hand, increases in thickness (to the detriment of the OH horizon if present, which disappears) when conditions are favorable for the development of life in the soil, such as available water and suitable temperature, digestible and transformable organic matter. In order to understand the humipedon, it is therefore necessary to look at the profile and keep an eye on these two diagnostic horizons (Fig. S3 and Fig. S5 in Supplementary material). In general, they are produced by different pedofauna and microorganisms: the OH horizon is the result of arthropods, enchytraeids and epigeal earthworms; the A horizon is instead produced by endogeic and anecic earthworms. There are two easy-to-find exceptions: (i) when soil conditions are difficult for living organisms, the OH horizons are almost devoid of animals and the biodegradation of the organic substrate is carried out by fungi. The resulting horizon is a nozOF, i.e., a non-zoogenic OF horizon matted with fungal hyphae; the OF is not transformed into OH as usual because it is not eaten by animals but digested by fungi; (ii) earthworms periodically abandon dry environments, leaving the organic matter to be ingested and transformed by arthropods; these are ants or termites, or insect larvae that can produce an A horizon morpho-functionally similar to that of endogean and anecic earthworms (savannas, steppes, scrublands and Mediterranean forests).
Fig. 3illustrates the dynamics of these horizons in an Alpine spruce forest environment. The phenomenon was studied for the first time in France ([16], [15]) and then ecologically circumscribed and confirmed in Italy ([127]). The opening of the forest draws light and water at the soil surface; large earthworms arrive and feed in the OH horizon, expelling organo-mineral droppings. As the trees grow, they deplete the soil of nutrients and water, the earthworms retreat and the OH horizon reforms.
Fig. 3 - Amphi humipedon in a montane spruce forest in the Alps. Left: a black organic OH horizon overlies an ochre organo-mineral A horizon. Arthropods or enchytraeids develop in the OH horizon; target earthworms inhabit and produce the A horizon. On the right: graph from Bernier ([15]). There are many earthworms in the young or old stages of the forest and here the A horizon is very present and rather thick; in the central part of the graph corresponding to the stage of intermediate age of the forest, the A horizon tends to disappear in favor of a more organic OH horizon.
When trees are young, they feed on soil resources. When the trees are adults and vigorous, they can return nutrients to the soil. Finally, when the trees are old, they fall and feed the soil with all their mass ([4]). In general, the soil releases nutrients in the first stages of the life of a forest, then gradually rebuilt its capital immobilizing nutrients in shallow organic layers. And towards the end, it surpasses in nutrients to fuel the natural renewal of the forest and start the cycle again. The duration of the periods of depletion, restoration and preparation for renewal change with the tree species and the forest environment.
In addition to the stage of the sylvogenetic cycle, this alternation of soil horizons depends on the parent rock and the climatic conditions, which we know are particularly linked to altitude. This last aspect was carefully studied by Bernier ([14]) (Fig. 4).
Fig. 4 - Variations in the humipedon of a spruce forest as a function of tree age and elevation. From Bernier ([14]), modified to make it compatible with the modern classification of forest humus. With increasing altitude, the climate changes, becoming less conducive to the biodegradation of litter (the main factor is the lowering of the average air temperature). This favors the increase in thickness of the more superficial organic horizons (yellow and purple) to the detriment of the underlying organo-mineral horizons.
In a forest, the thickness of the superficial organic (OL, OF and OH) and organo-mineral (A) horizons change in space and time:
(i) when the climate becomes harsher for soil organisms, or when the soil becomes nutrient-poor during the growth of young trees, the biodegradation of the organic horizons slows down. The organic horizons are fed from above (loss by young trees of the leaves of low branches in the shade) and are also digested more slowly by the soil fauna. At the same time, the organo-mineral horizon A loses the entry of nutrients, which instead escape more rapidly because nourishing the roots of the rapidly growing trees; the A horizon becomes lighter in color and its glomerular structure breaks down and gives way to particular or massive structure;
(ii) at the end of the forest cycle when the canopy opens, sun, water, fauna arrive and the organic horizons can be eaten to become the organo-mineral horizon A; this horizon regains its glomerular structure and its storage capacity to store water and nutritive elements for the plants.
These two situations of the humipedon are called Moder and Mull humus systems. Named Amphi, a third system also develops between the two ([128]); called humus “gemellare” (twin humus) by Franz Hartman ([49]) and Amphimus in France ([131]), also known in Germany ([46]), this humus system helps to understand how forest soil works, with interdependent and alternating organic and organo-mineral horizons.
Forests in extremely cold and acidic environments have humipedons that are particularly rich in organic horizons and poor in pedofauna; the humus system is a Mor. Forests of extremely calcareous and dry environments have humipedons that are rich in organic horizons but in which a relatively active fauna is present in favorable periods; such a humus system is called Tangel. Even in these extreme environments the soil changes with the age of the trees but the renewal is not linked to the transformation of the organic horizons by earthworms but can involve a larger number of fungi and animals that feed on wood in humus systems called Ligno (Fig. 5 below, left and right corners).
Fig. 5 - Forest stages and corresponding change in humipedon. The 300-year cycle of an Alpine spruce forest can be subdivided into two important periods for the soil: the first 150 years of progressive impoverishment of the soil, with energy and mass migrating from it to the trees, followed by another 150 years of soil enrichment as energy and mass that returns from the trees to the soil. Three initial Para humus systems have been illustrated in Fig. 4: Crusto, Bryo and Rhizo. The Ligno system at the bottom of Fig. 5, which corresponds to the biodegradation of wood, is also classified as Para system. Generated by animals and fungi specific to this organic substrate, this biological process of wood recycling is particularly evident on the stumps and branches left to decompose-freely after the removal of the tree trunk. Decaying spruce stumps are often and vigorously colonized by the renewal of the same species. The Bryo system often precedes the spruce seedlings on the stumps.
Humipedon changes coinciding with the spruce forest stages studied in France were also observed in Trentino (Italy). A doctoral thesis has studied the physicochemical causes that led to soil modifications during the development stages of the alpine spruce forest ([127]), and has been recently published ([129]). Summarized in Fig. 6, the general characteristics reported on the plane of the two axes of the multivariate analysis are, from left to right, a decrease in temperature and an increase in soil moisture; from bottom to top we read an increase in pH and the thickness of the A horizons, a decrease in total organic carbon and the thickness of the organic horizons. Acid-North and Acid-South separate well for these characters and along the diagonal that divides the plane in two from the point on the upper left (South exposure) and on the lower right (North). Formations on basic substrate separate on the horizontal axis, along a soil moisture gradient. In the 4 series, we observe an evolution of the cyclic humipedon, consistent with what was illustrated in the previous figures (Fig. 3, Fig. 4 and Fig. 5), with an increase in organic layers in the stages of rapid growth of the forest and a return towards an environment inhabited by earthworms in the opening stages and the first period of forest renewal.
Fig. 6 - The development stages of the Alpine spruce forest, the corresponding humus systems, arranged by an ACP in the space defined by climatic and pedological variables. Figure constructed from the work of Zampedri ([127]), published in ([129]). Cutting the graph along the diagonal that starts from the upper left corner and reaches the lower right, the stations on carbonate rock (B, basic: limestone and dolomite; S and N, South and North respectively) are positioned in the upper half on the right, while those on acidic rock (A, acidic: granites and porphyries) end in the lower half on the left. High pH, CEC%, thickness of the maA and meA horizons, average temperature for the month of May, soil humidity and number of days with temperatures below zero push the stations into the upper left box. High organic carbon, thickness of organic horizons OF and OH and thickness of organo-mineral horizon miA, average soil temperature at 10 cm depth of months 2, 3, 4, 5, 6, absolute minimum in spring and autumn and average of the month of January, variables linked to the radiation budget instead push the points towards the lower left corner.
In the herbaceous opening (yellow background) we find a Mull, and exceptionally an Amphi in the most difficult condition (AN = Acidic North). In the two basic ones (BS and BN), the second stage of renewal (green background) is still characterized by a Mull, while in the acidic environment an Amphi to the south and a Moder to the north are found. The third stage is the critical one (orange background), in which the soil empties itself of its nutritive elements and water, giving them up to the rapidly growing trees, it is Amphi on basic and Moder or Mor on acid. The fourth (blue background), which in our forests rarely exceeds 200 years, is a Moder everywhere except in the best condition of BS (Basic Sud).
What can be seen in Fig. 6 regarding the inversely correlated determinants reported on opposite axes (two opposite horizontal and two vertical), could be interpreted as general trends in the functioning of the soil in high mountain Alpine spruce forest (for more details on soil horizon genesis, description and biology, see [132]): (i) the thicknesses of meA and maA horizons, both generated by large earthworms (meA = biomesostructured A horizon: the key fraction of the aggregate volume has a diameter > 1 mm and ≤ 4 mm; maA = biomacrostructured A horizon: the key fraction of the aggregate volume has a diameter greater than 4 mm), oppose the thicknesses of OF, OH and miA horizons (OF and OH = holorganic biotransformed litter; miA = biomicrostructured A horizon, from arthropods, the key fraction of the aggregate volume has a diameter ≤ 1mm); (ii) the temperature at 10 cm depth opposes the humidity of the soil; (iii) the total organic carbon of the soil increases under conditions of difficult bio-degradation of the litter.
From a general point of view, two phases can be identified in the forest soil of an Alpine spruce forest (Fig. 5 and Fig. 6): (a) a period of “extraction” of energy and matter to nourish the future of the forest (first 150 years), the autotrophic phase ([4]); (b) a period of storage of matter and energy necessary to replenish soil reserves and prepare new foundations for the future generation (second 150 years), the heterotrophic phase. In addition, the renewal of spruce appears to be favored by wood in the final stages of decomposition, at least in non-megaforbiae areas ([45]). The role of the mosses layer has also been discussed for some time, as it seems to favor regeneration in the long term but not in the short term ([85]). Generally, the circumscribed humus system that develops around rotting wood (limited to the stump, branch or root transforming in the soil) is called “Ligno”; the mosses system is instead called “Bryo” ([117], [133]). Bryo and Ligno can develop as a single system directly on the rock, or even on top of each other (Bryo on Ligno), or superimpose on Mull and Moder. It is important to note that the humus systems succeed each other in time in the forest stages; that the Mull system develops in clearings and under older trees, while the Moder develops in the intermediate stages. The Bryo and Ligno systems develop on Mull at the beginning and end of the cycle, and contribute favorably to the success of forest renewal. In contrast, Moder formation (intermediate stages of a non-overmature forest) slow down and prevent renewal (while still protecting the seeds?). The rotation shifts of forest production depend on the cultivated species. However, it rarely goes over 100-150 years in Europe. A part of the necessary return on investments in the soil is thus lost in this way ([4]). Let’s take dead wood as an example of energy entry into the soil. Ultimately, wood corresponds to stored solar energy whose use is possible by making it available to soil heterotrophs and generating new trophic chains. A new ecological pyramid takes place in the forest when wood reaches the ground. Once the energy source (in this case wood) is exhausted, these living organisms are reduced, becoming partly dead organic matter in the soil, with a consequent dilution of the release of nutrients into the soil as their bodies are mineralized. The same process of ecological complexation/restitution within the forest occurs every time dead organic matter reaches the ground.
This is what happened to agricultural land too, which lost its organic matter following intensive practices that did not give the necessary importance to soil biology ([71], [81]).
A soil-conscious forestry
Autopoiesis is the property that Maturana & Varela ([78]) attributed to living systems in 1980, regardless of scale. This is a very interesting concept for foresters because it explains well what a living system is. Further information in Appendix 7 (Supplementary material).
How to practice soil-conscious forestry
A forestry manager begins by recognizing the real phytocoenoses to which associate the potential equivalent ones. In them, it plans a complete forestry cycle, respecting the foreseen potential evolution of the system (composition of species, especially tree species, length of the life cycle of trees, all compatible with the practical and economic needs of forestry). Each forest ecosystem corresponding to a given phytocenoses is called “forest station” ([112]) or “forest type” ([36], [33], [130], [29]). We know that the forest type contains a relatively homogeneous environment in terms of biological, geopedological and climatic characteristics, and that it is divided into parcels by man for forest management convenience.
To prefigure the potential evolution of the system, forest planning is also based on the historical past of stands; in the parcels the manager proceed with an inventory of the structure, age, height and distribution of trees by species. Historical and recent examples in italy can be found in Mencuccini et al. ([80]), Dellagiacoma & Motta ([35]), Motta et al. ([86], [87], [84]), Berretti et al. ([17]), Del Favero ([29], [30], [31], [32]), Notarangelo et al. ([90]). There is also a model for Italy relating to the altitudinal increase over the decades of the phytoclimatic zones due to climate warming ([93]). For an article dedicated to the importance of the non-homogeneous structure of the forest as supported by Susmel ([112]), especially when aiming at the conservation of biodiversity, please refer to the recent work of Savilaakso et al. ([105]).
In general, the management plans include an important chapter on wildlife, linked to the natural renewal of the forest. Aspects of the soil are considered such as depth, rockiness, type of parent rock, slope, degree of coverage; often in the management plans there are chemical-physical analyzes (pH, bases, texture) carried out in points representative of the forest type. However, 50-60 years ago much more importance was given to humus, a practice that was then abandoned. The reason for this abandonment is due to the fact that the soil classification was purely descriptive ([109]). For the superficial part of the soil called “forest humus”, there was also a lot of confusion in the terms ([49], [42], [131], [54]). As a precaution, in Italy many forestry technicians often reported Mull-Moder type humus in their forest management plans, which corresponded to a wide range of humus between Mull and Moder (almost all existing humus), which is useless for practical purposes. Today there is a standardized international reference for an effective classification of what is called the Humipedon (the organic - OL, OF, OH; HF, HM, HS - and organo-mineral - A - surface horizons of the soil - [134]).
Forestry that pays attention to the soil should include an inventory of humipedons by structural type, and an inventory of the animal biodiversity of the soil. Studies on microorganisms would also be desirable, since the decomposition process depends on the relationship between known categories of fungi and bacteria ([126]) and on the soil type ([19]). Each stand development stage of the forest is important for maintaining the gamma diversity ([123]) and the whole community nestedness ([95]). The authors of the latter work argue for example that each stage of population development corresponds to specific sets of soil bacteria, fungi and arthropods; to maintain all of the biodiversity in the soil after a forest fire, it is necessary to reconstruct a mosaic that contains all the stages of forest development, the most important of these stages being that of old settlements.
Below are some points to consider for the formulation of a soil-conscious forestry, i.e., the application of silvicultural techniques taking into account the functional potential of the soil.
The renewal of the forest
It is known that the basic principle of silviculture is to maximize the product of the forest by ensuring that the value of the forest itself does not decrease over time, but remains or grows in the future ([68], [106]).
The second thing that a forest manager asks himself is this (after remembering that: “Damn, when are Romeo and Juliet, whom I left at the bar last night and who were supposed to help me early this morning, going to show up?” - We will ask them for their thoughts on the article in the conclusions): what is the natural evolution of the forest on which I am called to intervene? Where is “my” forest going, is it changing and how?
In fact, today foresters know that if they do not intervene, if they let it grow freely, the forest will do very well (unless humans act on it from distance, diverting water courses, causing the acidity of the rain, etc.). But the foresters must make the forest produce, for economic reasons, and this becomes difficult in a climate that changes faster than it should, because we have to know where the forest is going as it adapts to the new climate (and new humans that influence the climate). The forest has centuries-old cycles, many times longer than human ones. Foresters do not manage for purposes aimed at their own generation, but for that of their children’s children, and even for those further away.
In order to survive, the forest system (plants + animals + microorganisms of that environment) must renew itself. The signs of renewal should be sought in areas that support a humipedon, i.e., the sequence of organic (OL, OF, OH, when present) and organo-mineral (A) horizons at the surface (first 30 cm) of the soil. The humipedon is different in regeneration sites compared to that found in the areas covered by intermediate age and non-perishable trees ([16], [129]). It is necessary to study the humipedon in clearings, under old decaying trees with more or less open foliage, near stumps or decaying wood and in all uncovered areas with herbaceous/shrub vegetation which often contrasts with that built under the areas covered by intermediate age and non-perishable trees. The humipedon changes in the areas where the forest prepares its rebirth. Recent studies have partially clarified the role of light, temperature and also herbaceous cover on seed development ([126]). The size of the canopy opening appears to play a key role. There are even-aged forests that are renewed due to catastrophic events (wind, avalanches, fire). In these cases, the change of the humipedon occurs a posteriori throughout the area opened by the incident.
When there are no signs of renewal, it is necessary to find the causes of this lack by studying the ecology of the forest. The main characteristics of a healthy and balanced forest are the presence of all the stages of the forest (renewal, growth, stasis and decay), occupying the surface of the whole in due proportions and all with their own specific humipedon, and a biodiversity correlated with the stages of the forest. All this can be verified through phytosociological surveys (list of plant species by layers), census of wild fauna and pedological surveys (recognition of systems of humus and, if necessary, list of soil animals and microorganisms), in the different stages of the forest. If a forest has a balanced distribution of forest stages, then it will also show an adequate renewal stage; however, if this stage is absent, the reasons for this lack may be related to the imbalance of the system, in terms of specific composition, excessive homogeneity of age and structure of the forest, the absence or deficit of light on the ground and/or decaying woody material. In a forest that lacks natural renewal it is very likely that the older stage of decay does not exist, or is not advanced enough. It is then necessary to recreate the conditions for its progressive and protected aging, in order to lead it over time to the next stage of renewal. The mistake of the silvicultural past was not to recognize the importance of this stage of forest aging. It corresponds to the stage of reconstitution of the forest floor, from which the renewal of the forest ecosystem begins. At this stage, the soil is biologically, physically and chemically the most favorable for seed germination.
Remembering what was reported above, also expressed in Fig. 3 to Fig. 6, we can say that the forest shows a cyclical evolution, with phases of renewal, maturity and death programmed in harmony with the quality of the soil. When the forest is young it removes energy and matter from the soil to build the new population of trees; when it is mature, it slowly replenishes these withdrawals from the soil by injecting organic substance (litter and exudates) produced thanks to photosynthesis and collaboration with soil organisms; during the growth phase it gives up all its mass to soil organisms so that they can grow and nourish the new seedlings. These phases are seen at the humipedon level, with the growth in the forest of the OH horizon, which shifts the Mull system (without OH) towards systems with OH (Amphi on limestone, Moder on acid rock), to return towards a phase in Mull at the end of the cycle when the stand opens up due to loss of canopy.
Supporting the becoming of the forest
Each type of forest has its own stage of regeneration characterized by typical vegetation and humipedon. The study of the specific composition of the vegetation and the characteristics of the humipedon of the aging stage (deterioration of the topsoil and revitalization of the soil) of the forest provides valuable indications on the evolution of the system. By knowing the trend of climate change and the phytoclimatic bands of each region, the forester will be able to interpret the dynamics observed and glimpse the future of the forest. Aware of the difficulty of the long-term predictions required by forestry, the foresters will adopt a precautionary attitude: they will intervene cautiously (with light cuts) in order to accommodate the movement they has perceived in the forest and will periodically check the result of their action. If the result is an increase in the vigor of renewal and in the state of the forest in general, the foresters will continue this line of treatments (for example cuts in the oldest stage of the forest); if the evolution of the forest is not as expected, they will carry out new studies to understand the reaction of the forest, and on new bases they will try a different intervention capable of better accommodating the recent (even if unexpected) movement of the forest (e.g., widening the holes, starting from the margins, not cutting species that you thought were invasive, etc.).
Forest biodiversity
Respect for the evolution of the forest concerns above all its biodiversity, even the microbial one ([9], [138]). Biodiversity should be understood as the functional biological whole of the forest ([37], [60]). It is well known that old-growth forests contain old trees of maximum height for each species, and that the highest levels of biodiversity are found in these forests ([88], [34], [62], [2], [82], [105]). It is also true that the richest in terms of biodiversity are the humid tropical forests, where radiant energy and water are never lacking ([38]) and where the trees occupy multiple layers and reach impressive heights. It is therefore likely that the “stature” of the forest (height of the dominant trees) is related to the biodiversity of each formation ([37]), in accordance with the climatic region in which the trees grow. From this point of view, Lucio Susmel’s formulas ([112]) which relates height (S = stature, the average height of the few tallest trees in the forest, usually 3-5 trees per hectare) to other parameters of uneven-aged forests also have an ecological meaning that could be re-evaluated. We will show later how this can be taken into account (see chapter “Examples in Alpine environment”).
Exotic species
Out of respect for the original forest, the forester should not introduce new species. If species different from the local ones have arrived in the forest, or have been introduced in the past, the forester takes care of the endemic species so that they can resist the entry of the new species. If the new species manage to replace the local ones, the forester will support the formation of the new ecosystem. The removal of new species by complete eradication is only possible at the beginning of colonization. A few years after the new species ingression, it is useful to estimate whether the intervention is more harmful and costly in the long term than adapting to the presence of the new species.
This applies to all species, both plants or animals. When the bark beetle kills spruce forests, it means that this species that became food for the bark beetle was no longer in the natural balance of the system. However, these trees are lost for our economy but not for that of the forest which instead recovers them in the soil and will end up returning them to a new forest population, if left to evolve naturally. If the loss of this species represents a significant economic damage to the community, it will be necessary to intervene with the awareness that in the long term the replacement of the attacked species with a new species in equilibrium in the new environment must be envisaged. Similarly, when the pressure of ungulates prevents the renewal of the forest, it is important to promote the reintroduction of natural predators to restore the forest to its lost level of complexity. In some cases, following agreed, careful and respected conditions, the predator can also be a human hunter. Functional biodiversity is the best way to guarantee the homeostasis of the system and to combat the arrival of exotic species and diseases.
The growth of the forest and the soil organic carbon stock
The growth of the forest is continuous, as its trees never stop growing ([111]). For the future of the forest, what really matters is the balance of the entire forest for all the mineral elements that compose it and in terms of biodiversity. Those that remain in the forest as mineral elements useful for the development of life are contained in the living and dead organic matter ([37]). An ecosystem is in a state of growth when the dead organic substance is greater than the living one, as it is in the dead organic matter that all the living organisms in the ecosystem draw in order to grow. Since there are irremediably losses in the transition from dead to living organic matter (second principle of thermodynamics), the amount of dead matter is always greater than the amount of living matter. The growth of a living system depends on its biodiversity: if it continues to grow, it ensures the evolution and health of the whole ([51]). It is not possible to stop the growth of the forest as a whole, except by reducing its functional biodiversity and its necromass.
It is known that in old forests, 2/3 of organic carbon is found in the soil ([72], [103]). We have not addressed the issue of carbon storage in forest soil because there is still a lack of a real connection between the quality of the humipedon (humus system) and the quantity and quality of the stored carbon. An Eumull can contain as much carbon as a Dysmoder, but the molecules in which the carbon is fixed are very different: in organo-mineral excrements from earthworms in the first case, in slightly decomposed organic substance and very organic excrements of enchytraeids and arthropods in the second.
The world’s forest took 300 million years to form and reach this climate-dependent equilibrium; after to the last glaciation, current European forests took a few thousand years to recolonize the space freed by the retreat of the glaciers. Once the equilibrium is reached, storage should slow down and be equivalent to respiration loss. If it were natural, a thousand-year-old forest should have an accumulation rate close to zero.
Forest soils in France are sequestering substantial amounts of carbon ([57]). The total stock (down to 1 m) was higher under coniferous than under broadleaved stands (109.5 vs. 88.5 MgC ha-1, p = 0.006). Throughout France, between 1993 and 2012, considering all phases (age classes) of the production cycle, forest soils substantially accumulated +0.35 MgC ha-1 yr-1. Soil carbon sequestration (i.e., above- and below-ground litter production and decomposition, first 40 cm of soil, between 1993 and 2012) declined with tree age and was affected by stand structure. Jonard et al. ([57]) proposed two linear models of carbon sequestration, for even-aged (y = 0.71 - 0.0056 x) and uneven-aged (y = 1.38 - 0.0056 x) forests, where y is the SOC sequestration in MgC ha-1 yr-1 and x is the stand age. Basically, these are two descending lines with a loss in storage rate close to 1.1 Mg ha-1 in 200 years. For the uneven-aged forest the start is at 1 (for a 75 year-old stand) and the end at 0.26 MgC ha-1 yr-1; for the even-aged forest the start is at 0.71 and the end of the cycle at -0.41 MgC ha-1 yr-1. Roughly (average between coniferous and broadleaved), the total stock is around 100 Mg ha-1; with the measured growth rate, and starting from a SOC value of zero, this corresponds to 100/0.35 = 285.7 years of storage.
As today’s forests start from a relatively high SOC values (100 Mg ha-1), why do we observe such a high positive storage rate? In non-Mediterranean environment, the measured high rate of SOC storage could be due to global warming, as a consequence of increased tree growth ([23]), though this does not happen in all forests, for example not in beech forests ([77]). Higher in uneven-aged forest, it seems to be linked to different forest management ([1]). By extending the cycle beyond 200 years, the storage rate could become even higher ([104], [53]), reversing the slope of the models of Jonard et al. ([57]).
Jonard Mathieu, contacted via email, has expressed his thoughts on this topic in Appendix 8 (Supplementary material).
Human economy and harvesting
From an economic point of view, the forester must decide what percentage of current growth that he can take from the forest to meet the needs of the human populations that depends on this resource. The lower that percentage, the less the loss to the soil will be and the better for the forest. It is not true that the forest would die, or burn, or become diseased without human intervention. This is a big misunderstanding that only applies to such artificial forests which lost their natural balance. There is no “right intervention measure”; there is only a withdrawal that interferes as little as possible with the growth of the forest. Withdrawal greater than the current average growth will inevitably leads to a decrease in biomass and a loss of biodiversity in the long term (through the soil, biodiversity feeds on and is therefore correlated with the primary production out of the soil). The removal of biomass (unless it is infected biomass that can reinfect the forest) corresponds to a loss of resource for the forest soil. We could also consider this loss in terms of DNA, with an impact on long-term evolution as suggested in Zanella ([135]), but perhaps we are bordering on risky science. The reduction in the number of species in the forest is also harmful to the balance of the forest; the change in the percentage of tree species which tends to favor the woody species more suitable for the human market is a mistake if practiced on a large scale and in the long term.
The entire cycle of the forest must be kept active at all its stages. And with all species. The forester wishes to keep the entire forest as much intact as possible, respecting “reasonable” human needs, to be discussed from time to time, depending on the regions in which one intervenes, and taking into account the global, social and long-term needs of the forest. The foresters should carry out only cautious “thinning” in all stages of the forest, with the aim of not changing the structural and specific composition of the forest and aware that the more they log, the more they will influence the future of the forest, and the more difficult it will be to predict the future of the forest ([27], [26], [24], [25], [89]).
The key points of a silvicultural technique that gives importance to the soil are:
- minimum harvesting: cutting and removing the minimum possible (instead of removing the maximum while respecting the vitality of the forest);
- the maximum prolongation of the life of trees: maintaining even the decaying stage of the forest (instead of interrupting the cycle of production in the moment of stasis of growth to obtain a high productivity);
- respect for the current and future forest structure: carefully thinning at all stages of the forest (instead of thinning to plan the growth and final cutting of a mature stand);
- respect for the entire biodiversity of the forest in the long term ([5], [83], [110]): biodiversity becomes the main value of the forest (instead of favoring above all those tree species that are most useful for the timber market);
- replenishment of energy reserves and mineral elements in the forest soil: it is recognized that the decline stage of the forest floor must be maintained because it ensures the reconstruction of the soil’s nutrient capital of the soil.
Examples in Alpine environment
The Technical Lines for Corporate Forestry Planning of the Province of Trento ([100]), in the chapter dedicated to “Thematic inventory and the description of the forests” (page 55), the point of view of an eminent forester on the duration of the life cycle of a forest is reported ([118]): “Just as ecologically oriented silviculture distances itself from the spatial ordering of schematic cuts (cultivation ordering) and moves towards a subdivision of the forest based on natural-type spatial units, it must also move away from the mechanistic categories of the typical temporal ordering of the clear-cut forest and move towards biological time scales. In relation to this, it must be observed that the different stages of development of organisms (juvenile, growth, maturity and articulation stages), which are to be considered temporal indicators of their life course, are reached at different times depending on the biotope (faster in case of good fertility, less in case of poor fertility) of the tree species (earlier by pioneer species, later by definitive species) and by the environment (first by dominant species and later by dominated species)”.
To attempt to associate these principles with a practical case, let’s take the easily imaginable example of a mixed Alpine fir forest (fir, spruce and beech), montane, with an irregular structure and a rotation of 150-200 years (very common, that is), which we want to normalize. Nowadays, very sophisticated tools and techniques for both inventory and forecasting harvesting are available. For the purpose of this article, the method that was once taught ([22]), with the real and normal curves and the humps of the first to be cut to “normalize” the structure, is hasty but gives a good idea of how a forester works in practice on the forest with a chainsaw (Fig. 7). The universal law of the forest, and common to many living systems, is that an adult tree produces a population of many young trees. These new individuals decrease in number over the course of their lives, until they reach the single, last dying, tree of their generation. When that tree falls, the story of a new generation begins again. On the curves of Fig. 7, the phenomenon can be seen by moving along the x-axis from left to right; traditionally, foresters count the trees starting from the 20 cm diameter class; the part missing from the graph, those with diameters < 17.5 cm, would count in dozens of thousands of trees per hectare, a number that is drastically reduced in the first 50 years of the forest’s life. Darwin’s theory of evolution ([28]) predicts that over time, the trees best adapted to the environment in which they evolve (and that changes with them) will survive. Those individuals that know how to best cooperate with each other and with the other species in the system to use of available resources will survive ([76]).
Fig. 7 - The normalization cuts. In the figure the “norm” (theoretical) lines are shown in green, the crosses (+) indicate the real trees to be removed to normalize the distribution of the number of trees with age. The black points at the end of the cycle correspond to old trees, released to improve the biodiversity of the forest. The diagram shows that two cycles of managed forest can be inserted in 300-400 years. It is as if, in order to increase the woody production of the forest, the forester had removed the forest from its “overripe” high forest stage and had forced the forest to remain young. This action determines the increase of the surface occupied by the central stages of the forestry cycle (2 and 3, young and mature high forest), to the detriment of that in which the regeneration and old age stages develop; the latter shorten and overlap (1-4). The middle stages with Moder humus systems become dominant.
Pro-Silva Helvetica offers real models of uneven-aged forests online. The first of these is the forest of “l’Envers à Couvet/NE” ([99]), with 70-80 trees ha-1 with a diameter of 20 cm which ending in a single tree with a diameter of 150 cm, 58 m high and 276 years old.
In order to mimic intervention, we propose a more irregular and more frequent structure when sliding upwards in formations with dominant spruce, defined as “multiplane” in the Forestry Typologies ([120]). Due to climate warming, these spruce forests will experience the entry of broadleaved trees and silver firs from below, as a consequence of the upward movement of the vegetation belts corresponding to the beech forest. Moving the structure of spruce forests towards a diameter’s distribution closer to that of mixed fir forests (similar to that of Pro Sylva mentioned above) could be a step that many foresters could share. Fig. 7 shows that two identical distributions of the number of trees in the forest stages were followed. In Fig. 8, however, the two curves have been grouped together to form a single cycle of double duration. The grouping assumes that the forest not subjected to a silvicultural regime can have a shift that lasts twice as long as the current one.
Fig. 8 - Soil-conscious forestry involves: (1) promoting the aging of the population; (2) checking the presence of renewal in balance with the biological functionality of the soil; (3) careful sampling of the woody biomass in the humps of the distribution of the number of trees by age class; (4) doubting about the silvicultural action undertaken, and consequently, repeated monitoring of the forest’s response in the years following the felling. For the forest to continue to exist, it is necessary to respect the return to the soil of the nutrients that the trees have taken to grow. In the past, the length of the restitution phase was shortened because it was considered not essential for the functioning of the forest. The few trees of stage 4 correspond to rare individuals scattered among those of the three previous stages, released not for their action on the soil but to increase the biodiversity of the forest ([67], [50], [58]). The space occupied by a long forestry cycle has been managed in such way as to generate two shorter cycles on the same surface, gaining in terms of volume produced and production times.
The nutrients are found in different “reservoirs”: the A horizon of the Mull system (macroaggregates of organo-mineral substance produced by earthworms); the organic OH horizon (microaggregates and minute particles of organic substance produced by enchytraeids and arthropods) of the Moder system; the decomposing wood of the Ligno system (ligOF and ligOH horizons - [133]). The groups of animals in each system associate the humus systems with the dynamics of the forest, allowing the release or fixation of nutrients at the right time. The A horizon is ready to release the ions that bind the organic and mineral substance in the macro-aggregates; the OH horizon is instead made up of blocked organic matter awaiting to be processed. It can be transformed into the A horizon by earthworms in a favorable environment for such animals. Dead wood is also very important in the forest ([116]). The transformation of wood into aggregates rich in nutrients available for plants is due to a specific fauna and fungi ([102], [48], [117]) that transform branches, trunks and stumps into spongy horizons that release nutrients for the roots of the plants. The humipedon evolves with the stages of the forest in this way:
- in the senescent stage and in the early juvenile stages, the A horizon of the Mull system (earthworms) and the ligOF and ligOH horizons of the Ligno system (fungi and wood fauna) develop;
- in the intermediate stages the OH horizon increases in thickness at the expense of an A horizon which tends to disappear (from a thick A from earthworms in the Amphi of the first stages of development of the tall forest, to a thin A not from earthworms in the Moder of the dense perches and in the adult tall forest still growing strongly;
- in the adult stages of relative stability, a redistribution of nutrients takes place outside and inside the soil, with a consequent decrease in the thickness of the OH horizon, storage of nutrient elements in the soil in the form of macroaggregates of the A horizon, or in any case of more organic substance easily recyclable in dead wood and partially biodegraded.
In all these stages, the allocation of the resources produced by photosynthesis evolves accordingly and should be studied in more detail. It is known that trees invest more in trunks than in branches or leaves as they age ([52]). It is also known that they grow less tall once they reach sexual maturity ([115]). Moreover, we are beginning to understand the process of rhizodeposition and estimate its importance in the carbon cycle in the storage of this element in the form of organic molecules of different complexity in the soil ([122]). It has been found that such deposition is associated with selected soil microorganisms ([119]), and that these microorganisms are often symbionts of plant roots that activate a new process called rhizophagy ([70]). Finally, it has been reported that Rendzina in different environments are different plant-soil systems and produce humic acids with different molecular weight and functionality ([101]).
Regarding the productivity of mixed uneven-aged forests such as that of l’Envers, Switzerland, it has been reported that that from 1974 to 2008 (35 years), the volume of the forest measured in 1975 has been removed ([99]). This means a stand renewal rate of 35 years and corresponds to a huge cutting rate of 10 m3 per hectare per year (1000 m3 / 100 years).
Fig. 9 - Left: re-working of the figure on page 4 of Pro Silva Helvetica, Portrait de la forêt jardinée de l’Envers à Couvet / NE. Harmony that happens in the soil between utility and beauty ([99]). The figure represents the number of stems per hectare on the ordinate for the list of 5 cm diameter categories on the abscissa. It originally showed the curves corresponding to four historical years (2018-red, 2002-brown, 1946-beige and 1896-yellow) and also the norms (discontinuous black lines) calculated by renowned forest managers (Liocourt and Schuetz, in [99]). Two normal functions have been added to this graph in green (with beech dominant) and in blue (with fir dominant), defined starting from the same stature (that of the tallest tree in Envers, 58 m), using two maximum diameters (Dmax) and two coefficients of passage from one 5 cm class to another (K). On the right: the cycle of withdrawal and return that occurs in the soil in harmony with the development of the chronological classes of the high forest, as illustrated in chapter 2, and which is missing from the figure.
Hence, it seems possible to remove in 35 years what a forest produces in a cycle of a few hundred years. Although such figures look realistic, there is probably something missing in terms of how the system works. We took the liberty of reproducing the figure on page 4 of the factsheet ([99]) - which corresponds to the evolution of the distribution curve of the stems in 1896 (yellow), 1946 (beige), 2001 (brown) and 2018 (red) - and adding two new curves (Fig. 9). The additional two distribution curves of the number of stems with age were calculated using two growth coefficients (K) and two maximum diameters (Dmax) obtained by the Susmel’s formulas for two hypotetical forests in the mountainous area of the Abieti-Fagetum, one dominated by fir (blue line) and one by beech (green line). In both cases, the height of the tallest tree in l’Envers (a 58 m tall silver fir) was taken as the stature S in the formulas. The values calculated with the equations of Susmel ([112]) for S = 58 m are, with fir dominant (eqn. 1):
with Dmax = 2.64 and S = 153 cm, while with beech dominant (eqn. 2):
with Dmax = 2.33 and S = 135 cm. Susmel’s formulas were constructed based om measurements in Italian relict nuclei and primary (virgin) forests in Croatia ([113], [114], [112], [59]) as a potential ecological model for the Italian alpine forests. By the way, the search for a key parameter that could facilitate forest management, in the eco-functional line of thinking of the stature (S) of Susmel, is still ongoing. Seidel & Böttger ([107]) recently proposed a parameter called box-dimension (Db), based on laser measurements addressing the quantity and vertical distribution of plant material. This parameter could represent a modern alternative to the stature (S) because it explains 86% of the variability in the functional complexity of the forest and it is linearly correlated with basal area, mean height of the greatest crown projection area and biomass of the stand.
In Fig. 9, the l’Envers forest lies between the blue and green curves obtained with the Susmel’s equation. It is interesting to observe that the formation of dominant fir with beech (blue) delimits the model downwards, while that of dominant beech with fir places an upper limit (green). From an ecological point of view, this means that with the climate warming and the consequent rise of the beech belt, we must expect shifts upwards and to the right. The Susmel’s curves are already shifted further to the right on the graph, and deviate in a curious way from the real ones, as if the young stages were closer to those of the beech forest and the old ones closer to those of the fir forest. Another puzzling is the distance of the dominant beech curve from those of the real distribution (those of l’Envers forest): it is like if medium- and large-sized trees were missing. These are likely the trees removed by foresters with production cuts.
Fig. 9 also shows a cycle of returning nutrients to the soil, which is only partially covered by the real graph and also by the graphs produced using the Susmel equations. The balance between extraction and return is in favour of the former in the juvenile stages and instead becomes in favour of the latter in the final part of the cycle (100-200 cm of diameter; 150-400 years of age). Extending the shift could allow more nutrients to be returned to the forest by making the distribution curves evolve upwards and to the right, as indicated by the orange arrows in Fig. 9. The increase in necromass due to the extension of the shift would eventually feed the renewal, which could return to the historical values of 1896. In fact, at page 12 of the report Pro Silva Helvetica ([99]) on the forest of l’Envers, it is stated: “...not to mention that the most important thing is not what we harvest, but what we leave (Couvet, division 109, never 2009)”.
Always in the spirit of Pro-Silva, on page 19 of the description of the uneven-aged forest of Tscheppa Verda ([98]), under the picture of a beautiful specimen of Silver fir, an observation by Walter Trepp dated Aug 26, 1986 is reported: “….Is this tree sick? I bet not! Every year, at the end of fall, part of the needles turns yellow. This is how tree get rid of the dead needles. Trees with wide crowns - typical of garden forests - produce more dry needles than trees with narrow crowns in regular high forests. The scientists have obviously not yet integrated these observations!”.
This is probably a way of restoring the nutrient potential of the soil which needs to be re-evaluated in silvicultural terms, allowing the trees to age beyond what seems to us economically invalid. Old trees produce more seeds, more pine cones or more fruit ([92]) than younger ones, as in the last period of their life they distribute the maximum of their genetic potential as an inheritance.
Planet forest management
From the point of view of forest management on a planetary scale, we know that even primary forests are affected by anthropogenic climate change and therefore cannot be longer considered strictly primary. However, there remain forests from which nothing is harvested, that complete their full cycle by investing all their dead parts in the soil.
We believe that the time has come to decide at the global level (FAO?) to prevent the direct exploitation of a relevant proportion of these forests (e.g., 20% of primary forests called “Intact Forest Landscape”), considering them an inviolable asset of Planet Earth. For these “untouched” forests, an income could be established equal to that which would result from their hypothetical naturalistic exploitation. This sum would be paid to the countries where these forests grow, in order to preserve them as an asset of the Planet and of humanity. We believe that soil-sensitive forestry should be applied to all the other forests in the world since it is the only one capable of guaranteeing the general conservation of the planet’s biodiversity.
Noteworthy, forests have a very important impact on the climate and that they constitute real “biotic pumps”: Makarieva & Gorshkov ([75]) calculated that the forests of our planet can generate and feed “flying rivers” - rapid overview of this captivating discovery in Pearce ([91]) - whose existence has been proved for the Amazonian and Congo forests ([136], [39], [108], [43]).
Soil-conscious forestry and new forestry plantings in climate change
Climate warming causes plant species from lower altitude or latitude belts to migrate to higher ones. Therefore, species belonging to the lower phytoclimatic belt should be favoured in the upper belt, allowing current ecosystems to follow the ongoing global warming and gradually lose the least adapted species. In the plains and in the low mountains where the dry period of the year is prolonged, an OH horizon appears above the A horizon with a consequent shift of the Mull system towards the Amphi. In the mountains or in a more continental environment, when the rainfall is sufficient, the transformation of the Moder into Amphi and Mull is observed, with the reduction in thickness or disappearance of the OH horizon, and the integration of its organic matter content by the earthworms in an underlying A horizon. This would mean that in the environments that tend to become drier, the earthworm fauna is replaced by arthropods; in the mountains, however, where there is no lack of water, the arthropods are displaced by earthworms. Obviously, the change in the soil will soon correspond to the arrival of different plant species. However, these are personal observations conducted in France and Italy that should be confirmed by scientific investigations.
Coppice forest, wood arboriculture, urban parks
From the point of view of a soil-conscious forestry, coppices, plantations and urban parks are anthropized systems similar to agroforestry. These are ecosystems that belong to anthropic evolutionary lines and create new biodiversity. Although interesting from a biological point of view, these ecosystems are recent in terms of evolution. When they are located in phytoclimatic zones allowing the ecosystem complexity to grow up, and left to evolve without human intervention, these systems become new forests, and mix with the existing ones. From an evolutionary point of view, the coppice is a mutilated forest: humans have decided to bring the foliage to the ground, removing the trunks of all the trees. By doing so, they limited the forest’s biospace, dramatically lowering its potential stature for economic reasons, such as the use of wood as a source of energy. Moreover, the specific tree composition of coppices is often reduced, making them monospecific cultures as in agriculture. In wood arboriculture, the planted species are selected to maximize wood production; a system layout is used that facilitates mechanization; even if polycyclic with the presence of shrubby species, these ecosystems have nothing to do with real forests.
The coppice has a long history of coexistence with man. In the Italian mountain regions (which is almost the entire country) these forests have played a fundamental role in the development of human societies, substantially supporting the economy. For this reason, these forests have also been well regulated so as not to be lost. With the oil revolution, coppices experienced widespread abandonment, triggering a series of ecological dynamics more complex than assumed in the past, which are much studied and debated nowadays. To date, depending on the socio-economic developments of the last decade, these formations were subjected to restoration in various areas, and are now exploited again for the production of firewood and poles, while the inland populations are rediscovering and adapting uses (craftsmanship, family-scale woodworking industries) that were thought to be forgotten forever. There is a biodiversity linked to the coppice forest ([79]), just as there is that linked to the mowing of secondary prairies, often subject to protection at community level. As with the latter, the coppice forest has also characterized the traditional landscape ([94]), thus it is at the basis of ecological and landscape aspects, traditions, uses and customs and therefore it should be safeguarded ([74], [79]).
Urban forests are often exotic trees, juxtaposed with criteria reflecting the functional aesthetics of cities, interspersed with meadows which are also very simplified and related to human recreational activities. Their management is entirely dictated by principles of safety and practicality that are not those applied in the forest. These “tree systems” are simplified human deviations and manipulations, practical and convenient from an economic and social point of view, but they are not forests, though providing the planet with new anthropogenic plant covers.
The soil of these tree systems is also different from the natural one, resembling the agricultural soil, often tilled before planting, or urban soil often containing the remains of buildings and debris of all kinds. In the long run, the dynamics of living organisms will return these soils back to a more natural stratification. Being in the plains or in the city, the humipedons very often tend to become earthworm Mulls, especially when irrigated. From a soil point of view, a diminished forest corresponds to a diminished soil. As in other situations, provided that urban forests, coppices and plantations are sustainable in the long term (probably because they are limited in extension and receive inputs from outside), we can consider them as highly anthropized forests but almost essential to human development.
Agroforestry
From the perspective of soil-conscious forestry, we consider agroforestry as territory lost to the real forests. Agroforestry areas are hybrids between forest and agriculture where the laws of the natural forest do not apply. With agroforestry, agriculture is brought into the forest (or vice versa, as in the case of forestry revaluation of areas abandoned by agriculture). The focus here is on some human “consumption” ([6], [13]). In non-tropical countries, private forests already more or less managed by man are modified to make them compatible with a different exploitation model often linked to agritourism. In tropical countries, planting of trees for timber production on abandoned agricultural land is not uncommon nowadays all over the world, also as an action to mitigate the climate warming.
Agroforestry creates a new clade of natural evolution, which may be economically interesting, but which must be scientifically separated from forest biodiversity. A salami made with pork from free-range pigs feeding in the forest is obviously tastier (and maybe healthier) than those produced with porks confined in battery-fed pigs. Would it be possible to create forest-like environments for these animals, starting from non-forest environments? From the point of view of the soil, agricultural fields that become “half forests” should be preferred to forests transformed into “half crop fields”, to put it more directly.
Conclusions
Soil-conscious forestry is the application of forest sciences research to principles that at first glance seem to contradict those of the past, that is, as little interventions and as long a cutting cycle as possible. Actually, if we have come to these resolutions, it is because things are not going as hoped: if we continue as before, the forests could disappear. Perhaps, we do not have yet the knowledge necessary to manage natural ecosystems in the long term. Since the future of humanity depends on forest health and on the ability of humans to coexist with the largest possible number of living organisms on the planet, it is good to make a conscious decision not to touch a relevant portion of the planet’s forests within the limits of a worthy coexistence, with enough food and comfort for humans as well... is that possible?
Soil-centered forestry means helping all forests to evolve towards a stage very close to “old growth-forest”.
The metaphor of the forbidden apple applies to aspects of forestry that are not obvious. They deserve the full attention of science: soil reserves and biodiversity have limits that must not be exceeded.
Soil cannot be exploited beyond a certain point. It is not just a matter of quantity. Soil also recycles the DNA of the ecosystem, which is the DNA of all living biota that inhabit the ecosphere. It is from the ground that the life cycle of the forest begins again. Biodiversity is not only the number of species, but also the functional web of relationships between species: when an ecosystem is destroyed, the network of communication and exchange that kept it alive is also lost. When parents or grandparents are lost, the foundations on which the family rested are lost. All the components of a human society are necessary for the good functioning of the whole, and the same is true for the forest. In order to live and evolve, it is also necessary to age and even to die.
“Are you sure that everything you wrote is useful for forestry purposes?” asked Romeo and Juliet, forest managers who were asked to read the paper and express their opinions.
“It is a manual of ecological forestry. The planet’s biodiversity and forests are in danger. It is necessary to consider the soil as a living system in both agriculture and forestry because everything comes from the soil. One approach is to stop using pesticides in agriculture; another is to allow forests to age more.”
“These are things that were more or less known, right?”, continued Romeo and Juliet, sharing their perspective.
“What about the idea that forests grow better without forest managers?”
“Ah. Do you think science is bad for forests?”, reacted Romeo.
“If there were no humans on Earth, would other plant and animal species be healthier?”
“Mhhhm… maybe… no doubt”, answered Juliet first. “But this is natural. We are born with an intrinsic and unstoppable drive for development that requires energy. To stay alive, we must extract energy from organic matter, which introduces death and degradation into the cycle of life.”
“Yes, but there is a forbidden apple: we can only hope to survive as a society connected to a living and autonomous system. From a biological and scientific perspective, for now, the only way out is on our planet with enough forest. The rest might be short-term economic planning.”
“OK, about this ancient apple story, where religion blamed women first - Juliet was a little upset - I do not see what this article says that is different from others.”
“When it comes to the apple, it might seem like the woman is at fault, but it was the guy who brought her right to it, and now he looks like an idiot. The article explains that young and old forests function differently; that we know little about soil microorganisms; and that we need to learn how to classify humus. Do you know the difference between a Mull and a Moder?”
“My forestry professor”, Romeo replied quickly, “told me that humus is like the phoenix, a bird that can control fire and be reborn from its ashes. Maybe that is why it is difficult to classify.”
Funding
This research was funded by University of Padua, through the Ordinary Endowment Funds for Research (DOR, Dotazione Ordinaria Ricerca) attributed to prof. Augusto Zanella from 2007 to 2022.
Acknowledgments
We thank Mathieu Jonard for carefully answering some questions about carbon storage in the soil of French forests. The email exchange is reproduced in the chapter “The growth of the forest”. This article also arises from a long discussion involving Prof. Orazio Ciancio and Prof. Susanna Nocentini. The aim of the debate was to find practical indications of systemic sylviculture. We thank the founders of this modern forestry for the numerous suggestions and for the evolution of thinking that they have provoked in this year of fruitful exchange of ideas.
Data Availability Statement
Data from Silvia Fusaro’s and Roberto Zampedri’s doctoral thesis are available upon request (fusaro.silvia.17@gmail.com; roberto.zampedri@fmach.it).
Conflicts of Interest
The authors declare no conflict of interest.
References
Gscholar
CrossRef | Gscholar
CrossRef | Gscholar
CrossRef | Gscholar
CrossRef | Gscholar
CrossRef | Gscholar
Gscholar
Gscholar
CrossRef | Gscholar
CrossRef | Gscholar
Gscholar
Gscholar
Gscholar
Gscholar
Gscholar
Gscholar
Gscholar
Gscholar
Gscholar
Gscholar
Gscholar
Gscholar
Gscholar
Gscholar
Gscholar
Gscholar
Gscholar
Gscholar
Gscholar
CrossRef | Gscholar
Gscholar
Gscholar
Gscholar
Gscholar
Gscholar
Gscholar
Gscholar
CrossRef | Gscholar
Gscholar
Gscholar
Gscholar
Online | Gscholar
Online | Gscholar
Gscholar
CrossRef | Gscholar
CrossRef | Gscholar
Gscholar
Gscholar
Gscholar
Gscholar
Gscholar
Gscholar
Gscholar
Gscholar
CrossRef | Gscholar
Authors’ Info
Authors’ Affiliation
Jean-François Ponge 0000-0001-6504-5267
Muséum National d’Histoire Naturelle, Paris (France)
Faculty of Agricultural, Environmental and Food Sciences, Free University of Bozen-Bolzano (Italy)
Université Savoie-Mont-Blanc, Chambéry (France)
Dipartimento di Scienze e Tecnologie Ambientali Forestali, Università di Firenze (Italy)
Corresponding author
Paper Info
Citation
Zanella A, Bernier N, Zampedri R, Fusaro S, Mei G, André J, Ponge J-F, Giannini R (2024). The soil-conscious forestry and the forbidden apple. iForest 17: 252-268. - doi: 10.3832/ifor4584-017
Academic Editor
Marco Borghetti
Paper history
Received: Feb 07, 2024
Accepted: Jun 11, 2024
First online: Aug 16, 2024
Publication Date: Aug 31, 2024
Publication Time: 2.20 months
Copyright Information
© SISEF - The Italian Society of Silviculture and Forest Ecology 2024
Open Access
This article is distributed under the terms of the Creative Commons Attribution-Non Commercial 4.0 International (https://creativecommons.org/licenses/by-nc/4.0/), which permits unrestricted use, distribution, and reproduction in any medium, provided you give appropriate credit to the original author(s) and the source, provide a link to the Creative Commons license, and indicate if changes were made.
Web Metrics
Breakdown by View Type
Article Usage
Total Article Views: 9022
(from publication date up to now)
Breakdown by View Type
HTML Page Views: 5528
Abstract Page Views: 1969
PDF Downloads: 1333
Citation/Reference Downloads: 3
XML Downloads: 189
Web Metrics
Days since publication: 337
Overall contacts: 9022
Avg. contacts per week: 187.40
Article Citations
Article citations are based on data periodically collected from the Clarivate Web of Science web site
(last update: Mar 2025)
Total number of cites (since 2024): 1
Average cites per year: 0.50
Publication Metrics
by Dimensions ©
Articles citing this article
List of the papers citing this article based on CrossRef Cited-by.
Related Contents
iForest Similar Articles
Research Articles
Dynamics of humus forms and soil characteristics along a forest altitudinal gradient in Hyrcanian forest
vol. 14, pp. 26-33 (online: 10 January 2021)
Research Articles
Soil stoichiometry modulates effects of shrub encroachment on soil carbon concentration and stock in a subalpine grassland
vol. 13, pp. 65-72 (online: 07 February 2020)
Research Articles
The manipulation of aboveground litter input affects soil CO2 efflux in a subtropical liquidambar forest in China
vol. 12, pp. 181-186 (online: 10 April 2019)
Research Articles
Wood-soil interactions in soil bioengineering slope stabilization works
vol. 2, pp. 187-191 (online: 15 October 2009)
Research Articles
Soil fauna communities and microbial activities response to litter and soil properties under degraded and restored forests of Hyrcania
vol. 14, pp. 490-498 (online: 11 November 2021)
Research Articles
Soil respiration along an altitudinal gradient in a subalpine secondary forest in China
vol. 8, pp. 526-532 (online: 01 December 2014)
Research Articles
Impact of deforestation on the soil physical and chemical attributes, and humic fraction of organic matter in dry environments in Brazil
vol. 15, pp. 465-475 (online: 18 November 2022)
Research Articles
Spatial heterogeneity of soil respiration in a seasonal rainforest with complex terrain
vol. 6, pp. 65-72 (online: 07 February 2013)
Research Articles
Seasonal dynamics of soil respiration and nitrification in three subtropical plantations in southern China
vol. 9, pp. 813-821 (online: 29 May 2016)
Research Articles
Soil respiration and carbon balance in a Moso bamboo (Phyllostachys heterocycla (Carr.) Mitford cv. Pubescens) forest in subtropical China
vol. 8, pp. 606-614 (online: 02 February 2015)
iForest Database Search
Search By Author
Search By Keyword
Google Scholar Search
Citing Articles
Search By Author
Search By Keywords
PubMed Search
Search By Author
Search By Keyword