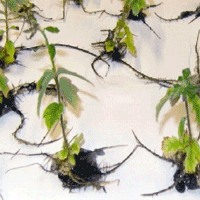
A quick screening to assess the phytoextraction potential of cadmium and copper in Quercus pubescens plantlets
iForest - Biogeosciences and Forestry, Volume 10, Issue 1, Pages 93-98 (2016)
doi: https://doi.org/10.3832/ifor1999-009
Published: Oct 13, 2016 - Copyright © 2016 SISEF
Short Communications
Abstract
The relevance of the environmental pollution by heavy metals warrants the necessity to develop and assess more efficient plant-based technologies. This study was conducted to evaluate a quick screening approach in order to investigate the cadmium (Cd) and copper (Cu) phytoextraction potential of Quercus pubescens in a micro-propagation system. Increasing concentrations of Cd (0, 5, 50, and 250 µM) and Cu (0, 5, 50, 250 and 500 µM) were separately applied to evaluate the effect of metals on their absorption and accumulation in downy oak plants. At high concentrations, Cd and Cu significantly reduced the dry biomass of shoots and roots and the plant tolerance index. Cd was toxic at increasing concentrations, inducing higher reduction of shoot dry mass than roots, whereas Cu increased dry mass at 5 µM. This study represents the first attempt to assess Cd and Cu uptake in Q. pubescens under in vitro conditions. The in vitro screening potential is meanly related to the following purposes: (i) proper selection of plant materials resilient to excess metals in the growth substrate; (ii) efficient removal of metals by the selected tree species; (iii) minor interference with the growth of plants accumulating metals in their tissues; (iv) rapid provision of plant materials for tree breeding programs.
Keywords
Introduction
The accumulation of heavy metals in soils and water poses a risk to the environmental and human health ([14]). Considerable progress in phytoremediation has been made with metals using hydroponically-cultured plants transplanted in metal-polluted sites, where plants eventually absorb and concentrate metals in their roots and shoots ([28]). Phytoremediation is a well-recognized, environmental-friendly strategy to control pollution by heavy metals ([1]). Phytoremediation includes strategies like phytoextraction and phytostabilization, which are defined by the preferential accumulation of pollutants by plants in their above- and below-ground organs, respectively ([23], [22]).
Among heavy metals, cadmium (Cd) is one of the most threatening due to its toxicity for the environment, crops and therefore for consumers ([20]). Cadmium has a strong carcinogenic potential ([11]) and is also very toxic for plants, affecting water and nutrient uptake, as well as their photosynthetic efficiency ([21]). Copper (Cu) is a microelement essential for plant growth, though it may become potentially toxic at elevated levels, particularly as a result of agricultural practices, and industrial or municipal waste disposal on land ([2]).
The plant capacity to tolerate metals can rely on a variety of resistance and tolerance mechanisms ([21], [29]). Plants have evolved avoidance strategies or exclusion processes, which reduce metal accumulation in cells, as well as mechanisms of surviving despite the accumulation of large amounts of metals ([24]). Phytoextraction can be achieved through hyperaccumulator plants, which exhibit the capability to take up from soil large quantities of heavy metals, which are then translocated to the epigeous parts ([30], [31]). However, these plant species have low biomass accumulation and removal potential in absolute terms. Therefore, selection and/or breeding of suitable plant genotypes based on biomass production, accumulation potential, root traits, growth rate, environmental suitability and metal resilience are required for their use in the remediation of heavy metal contamination ([29]). In the last ten years, research was focused on highly productive crop plants, such as crops, maize, sunflower, rice ([15], [18]), as well as on short rotation forest trees, i.e., willow and poplar ([10], [4]). Phytoextraction carried out with the use of other woody plants can be extremely interesting for the restoration of contaminated sites, where environmental conditions are unsuitable for growing energy plants, as well as for their longer reproductive cycles ([19], [6]).
Biotechnologies are currently available for better understanding the process of heavy metal uptake by trees and exploring their potential and exploitability in the remediation improvement ([5]). In vitro cultures provide several advantages when the plant cell tolerance to toxic elements is to be examined in experimental studies ([12], [9]). In this sense, in vitro screening is a preliminary tool for testing woody plant materials, reducing the growth period and the treatment duration, as well as the space needed for the experiments ([7], [8]). We anticipate that in vitro cultures represent a valuable research tool for phytoremediation of contaminated substrates and provide the required axenic conditions for screening the potential of a plant species to tolerate and accumulate heavy metals ([8], [13]). Particularly, Di Lonardo et al. ([8]) set up a test for the quick selection and assessment of poplar clones aimed to characterize the most suitable material for phytoremediation. This approach helps the quick evaluation of the most promising candidates (plant species and their clones) for further trials on polluted soils. Poplar has been used as model species in this screening test ([8]). However, the efficacy of this approach to test other woody plant species, not traditionally used for phytoremediation purposes, is unknown.
Quercus species are long-living forest trees whose micropropagation in vitro is sometimes troublesome. Nonetheless, preliminary results on the tolerance of downy oak (Quercus pubescens Willd.) seedlings to metal-polluted substrates has proven this species as an interesting candidate for the phytostabilization of contaminated sites, particularly in marginal areas ([6]). In this context, the aims of the present study were to: (i) assess growth perfomances of micropropagated plantlets of downy oak (Q. pubescens Willd.) cultivated on Cd and Cu artificially-contaminated substrate; (ii) define their uptake potential and metal bioaccumulation in roots and shoots at increasing concentrations of metals in the substrate.
Materials and methods
Plant material and in vitro growth condition
Plant material was obtained from cut buds of two years old downy oak seedlings. The buds were washed with tap water and their surface sterilized by Cl 2%. In vitro proliferating microshoots of Q. pubescens were subcultured on woody plant medium (WPM - [17]), added with 2% sucrose, 0.5 mg l-1 BA at pH 5.6, in baby food-glass jars sterilized by autoclaving at 121 °C and 108 kPa for 20 min. The aseptic cultures were incubated in a growth chamber at 23 ± 1 °C with a 16-h photoperiod (40 µE m-2 s-1) and routinely subcultured every 4 weeks. To promote rooting, microshoots were placed in contact with 20 mg l-1 IBA half strength WPM medium for 24 h in growth chamber. Finally, microshoots were transferred to phytohormone-free WPM medium for four weeks, added with 1 g l-1 charcoal to remove hormone carry-over effect and enhance shoot and root elongation. At this point of the experiment, the lack of sucrose in the medium induced well-developed rooted plants to autotrophic phase; gas exchange was improved with autotrophic micro-propagation (Fig. 1).
Fig. 1 - Plantlets grown in micro-propagation (five glass jars per treatment containing five rooted shoots each) after 4 weeks of Cu treatment.
Metal treatment
Well-developed rooted plantlets were transferred to phytohormone-free WPM medium containing different metal concentrations: 0, 5, 50, 250 µM Cd and 0, 5, 50, 250 and 500 µM Cu, supplied as CdSO4 and CuSO4, respectively. Twenty plants per concentration (four glass jars per treatment, containing five rooted shoots each) were used in the experiment. Other 20 plants were dried at 70 °C for 24 h and then weighed to estimate a mean value of the dry biomass at the beginning of the experiment.
Determination of metal content
After 15 days of treatment, plantlets were gently removed from the medium and roots were carefully washed. Roots were desorbed with 10 mM CaCl2 solution for 10 min to remove the adhering metals from the cell walls and determine the intracellular fraction of heavy metals. CaCl2 was used as extractant to avoid plasma membrane alteration and metal displacement. Plantlets were separated into shoots and roots, dried at 70 °C for 24 h and then weighed. The dry biomass production (dry biomass at the end of the experiment minus that previously estimated at the beginning of the experiment) was used as a measurement of the metal toxic effects ([3]). The tolerance index (Ti) was calculated as the ratio between the dry mass of plants (shoots and roots) grown in the solution with metal and that of plants grown in the control solution ([32]).
Roots and shoots of plantlets exposed to CdSO4 and CuSO4 were ground in a stainless steel plant mill and mineralized in 4 ml concentrated HNO3 and 1 ml concentrated HClO4 at 225 °C using an automated heating block (Digester DK 42/26®, Velp Scientifica, Milano, Italy). After digestion, Cu and Cd were determined by inductively coupled plasma optical emission spectroscopy (ICP-OES, Varian Vista-MPX). The procedure was validated with reference materials of poplar leaves (GBW 07604 - limit of detection 1 μg l-1). Metal contents were calculated as the product between mean dry biomass of plantlets (n = 5 seedlings) and mean metal concentration in each plant.
Statistical analysis
Treatments were performed in quintuplicate and repeated at least in four independent experiments. Normal distribution of the data was tested using the Kolmogorov-Smirnov test; since no significant departures from normality were found, parametric comparison methods were used ([25]). Data were averaged on a plant basis and the individual means were used for the analysis. The effects of metal treatment on the development traits and metal uptake were tested using the repeated-measures analysis of variance (ANOVA). To assess the differences between treatments for the measured parameters, a post hoc comparison of means was performed using the least significant difference (LSD) test or the Tukey’s test with α = 0.05. Statistical analysis was performed using the software packages STATISTICA® (StafSoft Inc., Tulsa, OK, USA) and SPSS® ver. 16.0 (SPSS Inc., Chicago, IL, USA) for Windows™.
Results and discussion
The micro-propagation system highlighted that high concentrations of Cd and Cu were toxic for downy oak. The study clearly illustrated the detrimental effects of excess metal on plant growth, as shown by the smaller dry biomass accumulation in shoots and roots of Cd-treated plants compared with non-contaminated plantlets (Fig. 2). As expected, Cd-treated plantlets showed a decreased shoot biomass at increasing concentration of the contaminant, while the biomass of roots was negatively affected in a similar way by all Cd treatments (Fig. 2). Regarding the Cu treatments, the dry biomass of shoots and roots of plantlets was significantly higher at 5 µM compared with other treatments and control conditions, suggesting a positive effect of low Cu concentration on plant growth ([16]). However, copper became highly toxic at higher concentrations (Fig. 2). For both metals, the accumulations in seedlings, shoot and root tissues increased with increasing metal contamination (Fig. 3). The accumulation of Cd and Cu was significantly higher at the highest contamination (50 and 250 µM Cd treatment; 250 and 500 µM Cu treatment - Fig. 3). Thus, the reduced growth of micro-propagated downy oak plantlets was likely due to the high metal accumulation in the roots and to a moderate metal translocation to the shoots.
Fig. 2 - Treatment effects on shoot and root dry biomass in Cd- and Cu-treated plants. Mean values (± standard errors) followed by the same letter are not significantly different after LSD test (p ≤ 0.05).
Fig. 3 - Cadmium and Cu contents (milligram per kilogram of dry matter) in shoots, roots and whole seedlings of Cd- and Cu-treated plants. Mean values (± standard errors) followed by the same letter are not significantly different after LSD test (p ≤ 0.05).
The tolerance index in shoots of Cd-treated plants was higher at low (5 µM) than at high (50 and 250 µM) metal concentration, while no significant differences in tolerance index of roots were observed between Cd treatments (Fig. 4). The tolerance index of shoots in Cu-treated plants was higher at 5 µM and 250 µM compared to other concentrations, while the value in roots was higher at low than at high metal concentration (Fig. 4). However, the low tolerance index at increasing contamination in both metal treatments corresponded to a reduction in plant growth. The high concentration of non-essential (Cd) and essential (Cu) metals could have negatively affected the photosynthetic machinery in these woody plants ([8]). Nevertheless, the potential for metal accumulation at the root level might have been affected by the release into the rizosphere of chelating agents with high affinity for metals, thus favoring metal sequestration ([26], [27]).
Fig. 4 - Tolerance index in shoots and roots of Cd- and Cu-treated plants. Mean values (± standard errors) followed by the same letter are not significantly different after LSD test (p ≤ 0.05).
The in vitro culture approach adopted in this study has proven to be effective to test whether downy oak could be further assessed for resilience to metal contamination over longer experimental periods and/ or under in situ conditions. Indeed, the metal concentrations used in our micropropagation conditions were very high, in particular when considering that downy oak is not widely recognized as an efficient forest species for decontamination purposes. Nonetheless, its potential Cd accumulation is high, as detected in pot experiments ([6]), and this might advocate its use in ecological restoration of polluted sites in marginal areas. Our results provide a preliminary evidence of the remediation potential of downy oak in vitro, although they should not be extrapolated to predict the effects of heavy metals on the growth of mature oak trees. The in vitro approach offered a stable growth medium, where plants freely absorbed metals from the substrate and translocate them to shoots, with minor interactions with surrounding conditions during the phytoextraction process. Common-garden investigations and provenance trials are planned in this species to test whether geographic variations of adaptive traits should be considered in the selection of material to be used in the restoration of contaminated sites in marginal areas of Italy. The in vitro screening carried out in this study allowed for: (i) testing the tolerance of plant materials to high-concentration metals in a short period of time; (ii) providing a suitable environment for the study of plant processes in response to metal treatments. This approach can be further improved towards appropriate breeding strategies and field trials.
References
CrossRef | Gscholar
Gscholar
Authors’ Info
Authors’ Affiliation
Giuseppe Palumbo
Erika Di Iorio
Bruno Paura
Dipartimento Agricoltura, Ambiente e Alimenti, Università degli Studi del Molise, I-86100 Campobasso (Italy)
Istituto per la Protezione Sostenibile delle Piante (IPSP), Consiglio Nazionale delle Ricerche (CNR), v. Madonna del Piano 10, I-50019 Firenze (Italy)
Dipartimento di Bioscienze e Territorio, Università degli Studi del Molise, I-86090 Pesche (Italy)
The EFI Project Centre on Mountain Forests (MOUNTFOR), Edmund Mach Foundation, I-38010 San Michele all’Adige (Italy)
Corresponding author
Paper Info
Citation
Di Santo P, Cocozza C, Tognetti R, Palumbo G, Iorio ED, Paura B (2016). A quick screening to assess the phytoextraction potential of cadmium and copper in Quercus pubescens plantlets. iForest 10: 93-98. - doi: 10.3832/ifor1999-009
Academic Editor
Tamir Klein
Paper history
Received: Jan 29, 2016
Accepted: Aug 02, 2016
First online: Oct 13, 2016
Publication Date: Feb 28, 2017
Publication Time: 2.40 months
Copyright Information
© SISEF - The Italian Society of Silviculture and Forest Ecology 2016
Open Access
This article is distributed under the terms of the Creative Commons Attribution-Non Commercial 4.0 International (https://creativecommons.org/licenses/by-nc/4.0/), which permits unrestricted use, distribution, and reproduction in any medium, provided you give appropriate credit to the original author(s) and the source, provide a link to the Creative Commons license, and indicate if changes were made.
Web Metrics
Breakdown by View Type
Article Usage
Total Article Views: 48082
(from publication date up to now)
Breakdown by View Type
HTML Page Views: 40597
Abstract Page Views: 2920
PDF Downloads: 3374
Citation/Reference Downloads: 58
XML Downloads: 1133
Web Metrics
Days since publication: 3185
Overall contacts: 48082
Avg. contacts per week: 105.67
Article Citations
Article citations are based on data periodically collected from the Clarivate Web of Science web site
(last update: Mar 2025)
Total number of cites (since 2017): 5
Average cites per year: 0.56
Publication Metrics
by Dimensions ©
Articles citing this article
List of the papers citing this article based on CrossRef Cited-by.
Related Contents
iForest Similar Articles
Review Papers
Heavy metals and woody plants - biotechnologies for phytoremediation
vol. 4, pp. 7-15 (online: 27 January 2011)
Review Papers
A review of the performance of woody and herbaceous ornamental plants for phytoremediation in urban areas
vol. 13, pp. 139-151 (online: 14 April 2020)
Research Articles
Can species Cedrela fissilis Vell. be used in sites contaminated with toxic aluminum and cadmium metals?
vol. 14, pp. 508-516 (online: 11 November 2021)
Research Articles
Variation of major elements and heavy metals occurrence in hybrid aspen (Populus tremuloides Michx. × P. tremula L.) tree rings in marginal land
vol. 13, pp. 24-32 (online: 15 January 2020)
Research Articles
Tolerance to heavy metal stress in seedlings of three pine species from contrasting environmental conditions in Chile
vol. 9, pp. 937-945 (online: 12 August 2016)
Research Articles
Heavy metal (Zn, Pb, Cd) concentration in soil and moss (Pleurozium schreberii) in the Brynica district, southern Poland
vol. 4, pp. 176-180 (online: 11 August 2011)
Research Articles
Links between phenology and ecophysiology in a European beech forest
vol. 8, pp. 438-447 (online: 15 December 2014)
Research Articles
Bioaccumulation of long-term atmospheric heavy metal pollution within the Carpathian arch: monumental trees and their leaves memoir
vol. 17, pp. 370-377 (online: 27 November 2024)
Research Articles
Analysis of biometric, physiological, and biochemical traits to evaluate the cadmium phytoremediation ability of eucalypt plants under hydroponics
vol. 10, pp. 416-421 (online: 24 March 2017)
Research Articles
Temporal analysis of pollutant metals in trees of three parks in Mexico City’s Metropolitan Area
vol. 18, pp. 138-145 (online: 01 June 2025)
iForest Database Search
Search By Author
Search By Keyword
Google Scholar Search
Citing Articles
Search By Author
Search By Keywords
PubMed Search
Search By Author
Search By Keyword