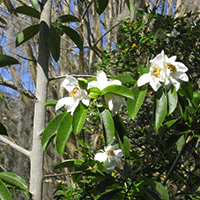
Inorganic and organic nitrogen uptake by nine dominant subtropical tree species
iForest - Biogeosciences and Forestry, Volume 9, Issue 2, Pages 253-258 (2015)
doi: https://doi.org/10.3832/ifor1502-008
Published: Dec 02, 2015 - Copyright © 2015 SISEF
Research Articles
Abstract
We explored inorganic and organic N uptake patterns by dominant tree species in a subtropical plantation of southern China to improve understanding of nitrogen (N) cycling in these forests. We labeled intact roots by brief 15N exposures in field hydroponic experiments. Nine dominant tree species were examined to compare the effects of functional plant group (conifers versus broadleaves), mycorrhizal types, and forest successional stages on N uptake. All investigated species took up glycine at lower rates than other N forms, with mean values of 2.55 ± 0.36 µg N g-1 d.w. root h-1. Nitrate uptake rates for all species (average 5.81 ± 0.35 µg N g-1 d.w. root h-1) were significantly lower than ammonium (36.86 ± 5.17 µg N g-1 d.w. root h-1). All investigated species absorbed ammonium for more than 80% of total N uptake. Nitrate acquisition by these species was about 14% of total N uptake, with only 6% for glycine. Conifers showed significantly higher uptake rates of glycine, but lower uptake of nitrate than broadleaves. Arbuscular mycorrhizal (AM) and ectomycorrhizal (ECM) tree species showed significant difference in nitrate uptake, with higher rates by AM tree species. Tree species at late-successional forest stages showed higher uptake rates of nitrate than those in earlier successional stages. Our findings indicate that ammonium is the dominant N source and glycine is a minor N source throughout forest succession.
Keywords
Plant Functional Group, AM Fungi, ECM Fungi, N Uptake, Subtropical Tree Species, Succession
Introduction
Nitrogen (N) is one of major elements limiting plant growth in terrestrial ecosystems over the globe ([17]). Although numerous studies have suggested inorganic NH4+ and NO3- are the most important N sources for most plant species, many species directly acquire organic N as amino acids from soil solution ([14], [25], [40]). Uptake patterns of inorganic and organic N may contribute to species coexistence ([23], [42], [9]).
Substantial organic-N is stored in cold climates, limiting mineralization of soil organic matter. Such high-latitude and high-altitude regions are often dominated by tundra ([26]), boreal ([8], [18]), alpine ([43]) and temperate ([3], [36], [11], [37], [38], [39]) forests. Many studies of inorganic and organic N uptake have been done in these ecosystems.
Recent studies in subtropical and tropical forests investigated N acquisition by tree species ([30], [29], [1]), showing dominant tree species in these regions absorb organic N as intact amino acids. Few such studies have been conducted in subtropical forests. Subtropical forests are widely distributed in southern China and occupy about 2.4×106 km2. In the past decades, a large area of subtropical forests has been modified by anthropogenic activities. Many plantations have been established in these regions to support local economic development. Low N availability in plantations can limit tree growth and wood production. Therefore, to clarify the N uptake pattern by dominant tree species will be beneficial to the management of these plantations through improved supply of N forms. Additionally, the importance of distinguishing plant N sources has increased for better understanding the ecological consequences of N uptake by plants ([6], [4]).
To investigate organic and inorganic N uptake by subtropical trees, we selected a subtropical plantation at the research station of Qianyanzhou. Nine dominant tree species (three conifers and six broadleaves, i.e., Pinus massoniana, Pinus elliottii, Schima superba, Magnolia officinalis, Cyclobalanopsis glauca, Machilus velutina, Cunninghamia lanceolata, Michelia maudiae and Cinnamomum camphora) were selected based on their successional stages and mycorrhizal types. Numerous studies have suggested that plant functional group and mycorrhizal type can affect root nutrient-uptake functions ([22], [13], [5], [19], [24]). Therefore, we hypothesized that broadleaves preferentially absorb nitrate, whereas more ammonium and glycine is adsorbed by conifers. Additionally, we hypothesized that ectomycorrhizal (ECM) tree species have stronger capacity to take up organic and inorganic N than arbuscular mycorrhizal (AM) tree species. Generally, nitrate dominates early successional soils, while ammonium becomes the major N form at later stages ([6]). We hypothesized that pioneer tree species preferentially utilize nitrate, while tree species in later successional stages utilize more ammonium and dissolved amino acids. To test these hypotheses, we conducted a short-term 15N labeling experiment in subtropical plantations.
Materials and methods
Study site
This study was conducted in a plantation at the Qianyanzhou Ecological Experimental Station (26° 44′ N, 115° 04′ E, 102 m a.s.l.), Jiangxi Province of southern China. The site is characterized by a typical subtropical climate. The mean annual air temperature was 17.9 °C ([41]), while mean annual precipitation was 1542 mm, with a dry period from July to August ([35]). The region is dominated by subtropical evergreen broad-leaved forests. Before 1983, the native forests were nearly completely cleared, leading to serious soil erosion and land degradation. Subsequently, several large projects of reforestation and sustainable land use projects were initiated to improve ecosystem structure and function as well as local economic development. With the establishment of these plantations, secondary forests have also developed. Soils are red soils, classified as aliudic cambosols and derived from sandstone, sandy conglomerate, mudstone and alluvium ([31]). Soils have total N concentrations less than 0.1% ([21]).
Field hydroponic experimental design
In these plantations, nine dominant tree species (Pinus massoniana Lamb., Pinus elliottii Engelm., Schima superba Gard., Magnolia officinalis Rehd. et Wils., Cyclobalanopsis glauca (Thunb.) Oerst., Machilus velutina Champ. ex Benth., Cunninghamia lanceolata (Lamb.) Hook, Michelia maudiae Dunn. and Cinnamomum camphora (L.) Presl.) were selected to examine their in situ N uptake by roots. They were divided into different functional groups: conifers versus broadleaves, ectomycorrhizal (ECM) versus arbuscular mycorrhizal (AM) trees or early versus late successional species (Tab. 1). The general characteristics of the 9 tree species were described in Tab. 2.
Tab. 1 - The nine dominant tree species analyzed in subtropical plantations at the Qianyanzhou research station. ECM refers to ectomycorrhiza while AM indicates arbuscular mycorrhiza.
Tree species | ID | Family | Functional group | Mycorrhizal type | Successional stages |
---|---|---|---|---|---|
Pinus massoniana | PMA | Pinaceae | Coniferous | ECM | Early |
Pinus elliottii | PEL | Pinaceae | Coniferous | ECM | Early |
Schima superba | SSU | Theaceae | Broad-leaved | AM | Intermediate |
Magnolia officinalis | MOF | Magnoliaceae | Broad-leaved | AM | Late |
Cyclobalanopsis glauca | CGL | Fagaceae | Broad-leaved | ECM | Late |
Machilus velutina | MVE | Lauraceae | Broad-leaved | AM | Late |
Cunninghamia lanceolata | CLA | Taxodiaceae | Coniferous | ECM | Late |
Michelia maudiae | MMA | Magnoliaceae | Broad-leaved | AM | Late |
Cinnamomum camphora | CCA | Lauraceae | Broad-leaved | AM | Late |
Tab. 2 - General characteristics of the nine dominant tree species analyzed in the Qianyanzhou forest ecosystem. Values are presented as mean ± SE of five replicates.
Tree species | Mean tree height (m) |
Mean diameter at breast height (cm) |
Water content (%) |
NO3--N (mg kg-1) |
NH4+-N (mg kg-1) |
---|---|---|---|---|---|
Pinus massoniana | 11.36 ± 0.80 | 21.06 ± 1.86 | 11.87 ± 1.34 | 0.89 ± 0.28 | 4.87 ± 0.44 |
Pinus elliottii | 15.48 ± 0.21 | 26.52 ± 0.84 | 9.86 ± 0.47 | 0.41 ± 0.07 | 6.41 ± 1.30 |
Schima superba | 19.90 ± 1.22 | 19.54 ± 1.58 | 14.12 ± 1.96 | 1.92 ± 0.12 | 14.60 ± 3.37 |
Magnolia officinalis | 11.20 ± 0.70 | 16.54 ± 0.92 | 19.47 ± 0.06 | 0.75 ± 0.06 | 18.93 ± 1.98 |
Cyclobalanopsis glauca | 10.00 ± 0.57 | 15.00 ± 1.23 | 12.60 ± 2.62 | 1.97 ± 0.56 | 21.74 ± 6.40 |
Machilus velutina | 5.30 ± 0.14 | 4.89 ± 0.15 | 4.17 ± 1.20 | 1.29 ± 0.24 | 6.61 ± 1.65 |
Cunninghamia lanceolata | 20.56 ± 0.56 | 25.82 ± 0.85 | 13.24 ± 0.95 | 2.48 ± 0.43 | 6.94 ± 1.20 |
Michelia maudiae | 8.92 ± 0.41 | 12.00 ± 1.03 | 9.18 ± 0.26 | 1.09 ± 0.11 | 15.40 ± 1.11 |
Cinnamomum camphora | 10.56 ± 0.65 | 19.16 ± 1.66 | 15.62 ± 0.88 | 1.24 ± 0.64 | 16.10 ± 3.21 |
To examine their N uptake, a mixture of ammonium, nitrate and glycine was used. Glycine was used as an organic N source, because it is a common low-molecular-weight amino acid in soils ([20]). Three labeling solutions and one controlled solution were used. Each solution contained equal parts of ammonium, nitrate and glycine (33 μmol N L-1 for each N form) to provide a “choice” of different N sources. Each labeling solution only contained one of the three tracers: 10 atom% K15NO3, 10 atom% (15NH4)2SO4 and 99 atom% 15N-glycine, while the other two were not labeled with 15N. This allowed to discern N uptake patterns of the chemical forms of N among the species. In addition, a solution containing all three N chemical forms without labelled 15N tracer was used as control to examine natural variation in δ15N values among the species. In addition to N, uptake solutions contained 10 mg L-1 of ampicillin to minimize microbial activity and avoid the decomposition of the amino acid and 0.2 mM CaCl2 to maintain the function of roots ([37]).
The labelling was performed at 9:00 am in July 2013. Soil temperature was about 26 °C. Five trees of similar size were randomly selected in each tree species. Four pairs of fine roots with diameter less than 2 mm (weight ranged from 0.05 to 0.20 g for a fine root; length was about 15 cm for every root) were dug out diagonally along the stem base for each tree. Each treatment had 10 replicates. After rinsing with water, they were immersed into the labeling solution in 15 ml centrifuge tubes, individually. After 2 hours, they were excised, washed with 50 mM KCl, and then rinsed with H2O. All root materials were dried at 70 °C for 48 h to measure dry mass. Dried roots were ground into fine power and weighted into tin capsules for measurements of N content and 15N/14N ratios by continuous-flow isotope ratio mass spectrometry coupled with an elemental analyzer (EA 1110 - CE Instruments, Milan, Italy) and a ConFlo II device connected to IRMS (Finnigan MAT 253, Bremen, Germany).
Calculations and statistics
15N calculations were based on 15N atom percent excess (APE), calculated as the difference between 15N treated and control plants. N uptake rates (µg N g-1 d.w. root h-1) were calculated multiplying APE by dry root biomass and its N content and divided by dry root biomass, APE of labeled N and the labeling hours. The contribution of each N form was calculated by dividing its uptake rate by the total uptake of three N forms.
Differences in uptake rates for three N forms among tree species were analyzed using a one-way analysis of variance (ANOVA). Two-way ANOVA was used to examine the effects of N forms and tree species on N uptake rates. Statistical calculations were performed with the statistical software package SPSS® ver. 18 (SPSS Inc., Chicago, IL, USA). Least significant difference (LSD) was used to compare the mean values as post-hoc test. All differences were tested at the P<0.05 level. Standard errors (SE) of means are presented in the figures as a variability parameter.
Results
Tree species and N forms as well as their interactions significantly affected N uptake by roots (Tab. 2). All nine tree species took up glycine at lowest rate as compared to other N forms, with mean values about 2.55 ± 0.36 µg N g-1 d.w. root h-1 (Fig. 1). Among tree species, P. elliottii showed the highest uptake rate for glycine, while C. glauca took up glycine at lowest rate, with significant difference in uptake rates between some tree species (Fig. 1). Uptake rates of nitrate for all nine tree species ranged from 4.30 ± 0.50 to 7.55 ± 0.33 µg N g-1 d.w. root h-1, higher than glycine uptake (ranging from 1.92 ± 0.14 to 7.01 ± 1.47 µg N g-1 d.w. root h-1) but lower than ammonium uptake (varying from 22.10 ± 1.60 to 56.56 ± 5.90 µg N g-1 d.w. root h-1). Pinus elliottii had the highest uptake rate for ammonium, while C. glauca showed the lowest uptake rates for ammonium (Fig. 1).
Fig. 1 - Uptake rates of glycine, ammonium and nitrate by nine dominant tree species in a subtropical plantation. Values are presented as mean ± SE of ten replicates. Different letters above the bars indicates significant difference in nitrogen uptake between nitrogen forms for each species (P<0.05). Labels of tree species are the same as in Tab. 1.
All species showed a preferential uptake for ammonium which accounted for more than 80% of total uptake for three N forms (Fig. 2). The contribution of nitrate uptake to all nine tree species was estimated to be about 15% of total uptake of three N forms, with the lowest value for P. elliottii (Fig. 2). The contribution of glycine uptake to P. massoniana and P. elliottii was similar to that of nitrate uptake. Thus, the overall pattern of uptake from the three N sources was: glycine < nitrate < ammonium (Fig. 2).
Fig. 2 - The proportional contribution of nitrate, glycine and ammonium to total nitrogen uptake by trees in a subtropical plantation. Values are presented as mean ± SE of ten replicates. Labels of tree species are the same as in Tab. 1.
Conifers showed significantly higher uptake rates for glycine but lower uptake rate for nitrate than broadleaves (Tab. 3, Fig. 3). AM and ECM tree species showed significant difference in nitrate uptake, with higher rates for AM tree species (Fig. 4). Tree species from early successional stage showed stronger capacity to take up ammonium and glycine than those from later successional stage, with significant differences in glycine uptake between those groups. By comparison, tree species at late successional stage showed higher uptake rates for nitrate than early successional species (Fig. 5).
Tab. 3 - Two-way analysis of variance (ANOVA) testing the effects of tree species and N forms on N uptake by plants in a subtropical plantation. P values for significant effects and interactions are reported.
Factors | Mean Square |
df | F | P values |
---|---|---|---|---|
Tree species | 6.927 | 8 | 5.930 | <0.001 |
N forms | 217.064 | 2 | 185.829 | <0.001 |
Tree species × N forms | 3.848 | 16 | 3.295 | <0.001 |
Error | 1.168 | 243 | - | - |
Fig. 3 - Uptake of ammonium, nitrate and glycine by broadleaves and conifers. Different lower-case letters above the bars indicates significant difference between broad-leaved and coniferous tree species (P<0.05).
Fig. 4 - Uptake of ammonium, nitrate and glycine by ectomycorrhizal (ECM) and arbuscular mycorrhizal (AM) trees. Different lower-case letters above the bars indicates significant difference between ECM and AM tree species (P<0.05).
Fig. 5 - Uptake of ammonium, nitrate and glycine by trees in early and late successional stages. Different lower-case letters above the bars indicates significant difference between tree species in early and late successional stages (P<0.05).
Discussion
We investigated inorganic and organic N acquisition patterns by nine dominant tree species in a subtropical plantation using short-term 15N labeling. These dominant trees showed similar uptake rates of nitrate to those trees in subtropical rainforests, but 3 times uptake of glycine and ammonium ([30]). Our estimated uptake rates of all three N forms were similar to those of temperate and sub-alpine trees ([38], [39]). Our results demonstrated that investigated dominant subtropical tree species preferentially take up ammonium, contributing about 80% of total N uptake by these dominant trees. This may relate to ammonium dominance of this soil solution (Tab. 2 - [15]). Additionally, this study used hydroponic solution, in which microbial competition and adsorption of NH4+ ions by soil minerals are excluded. NH4+ diffusion is relatively faster, with higher concentrations, in the uptake solution than in soils. These factors could increase ammonium uptake by roots of these subtropical trees.
Nonetheless, such N uptake patterns here provide a basis for understanding ecological consequences of N uptake by worldwide subtropical trees. In the rhizosphere, microorganisms strongly compete for N with roots ([16]). The strong dependence on ammonium of all investigated trees indicates that they could strongly compete for ammonium in soil solution. However, these dominant trees grow together very well. This suggests that rapid mineralization may provide enough ammonium for these trees; otherwise the other N sources may be not accounted in this study. Additionally, subtropical soils are generally characterized by low pH. Numerous factors such as acidic atmospheric deposition and N transformations have been suggested to cause soil acidification ([33]). Ammonium uptake by roots also has potential to decrease pH in the rhizosphere ([27]). This indicates that the preference for ammonium by these dominant trees could lead to the substantial decrease in soil pH during forest succession. However, this still needs further investigations for understanding the ecological consequences (e.g., soil acidification as well as tree coexistence) of the preference for ammonium by worldwide subtropical trees.
Both broadleaves and conifers preferentially take up ammonium and glycine (Fig. 3), but broadleaves show higher nitrate uptake than conifers. Therefore, our first hypothesis is partly supported. This indicates substantial difference in root physiology between broadleaves and conifers, but underlying mechanisms should be investigated for a better understanding of N uptake by roots.
Numerous studies have suggested that mycorrhiza can enhance N uptake by roots ([2], [32]). Although many studies have explored how ECM or AM affect N uptake by roots ([10], [28], [7], [12], [24], [34]), few studies have been conducted to compare N uptake by mycorrhiza. Here we observed that ECM tree species did not show stronger capacity to take up ammonium and glycine than AM tree species, while AM tree species acquire nitrate faster than ECM tree species (Fig. 4). These results are contrary to our second hypothesis. These species are known for mycorrhizal infection patterns, but we did not measure infection in this study. Therefore, our results represent a conservative speculation on effects of ECM or AM on N uptake by trees. Nitrogen-labeling of trees with demonstrated AM or ECM infections should be conducted.
Although it has been suggested that pioneer species acquire more nitrate while later successional species take up more ammonium ([6]), we did not observe this pattern in this study. Contrary to our hypothesis, tree species in both early and late stages utilize more ammonium and glycine (Fig. 5). However, late tree species showed higher uptake of nitrate and lower uptake of glycine than pioneer tree species. A possible explanation is that these plantations are part of secondary succession, developing on pre-existing soils, where ammonium is the dominant N form (Tab. 2 - [15]).
In summary, ammonium is the major N source for these investigated nine subtropical tree species and nitrate is also important for these trees except for Pinus species. Glycine was selected as organic N in this experiment and was not preferred by any species, but subtropical soils contain other dissolved amino acids which may be significant N sources for trees. Future investigation should consider other amino acids for a better understanding of N cycling in subtropical forests. As these results were measured using field hydroponic experiment, microenvironments around roots (e.g., microbial competition and interactions among roots) were changed. This could change N-uptake patterns. To improve knowledge of N uptake by tree species, in situ N uptake should be investigated including mycorrhizal status, microbial competition, soil N forms availability, seasons, and developmental stages of trees.
Acknowledgements
This study was supported by National Basic Research Program of China (2012CB 416903) and the National Natural Science Foundation of China (31130009 and 4107 1209) the Knowledge Innovation Foundation Program for Outstanding Young Scholar of the Chinese Academy of Sciences (KZCX2-YW-QN302).
References
CrossRef | Gscholar
Gscholar
Gscholar
Authors’ Info
Authors’ Affiliation
Qianru Li
Xingliang Xu
Qingkang Li
Huimin Wang
Key Laboratory of Ecosystem Network Observation and Modeling, Institute of Geographic Sciences and Natural Resources Research, Chinese Academy of Sciences, Beijing 100101 (China)
Key Laboratory of Tropical Forest Ecology, Xishuangbanna Tropical Botanical Garden, Chinese Academy of Sciences, No. 88 Xuefu Road, Kunming 650223, Yunnan (P.R. China)
Corresponding author
Paper Info
Citation
Li C, Li Q, Qiao N, Xu X, Li Q, Wang H (2015). Inorganic and organic nitrogen uptake by nine dominant subtropical tree species. iForest 9: 253-258. - doi: 10.3832/ifor1502-008
Academic Editor
Giustino Tonon
Paper history
Received: Nov 14, 2014
Accepted: Oct 07, 2015
First online: Dec 02, 2015
Publication Date: Apr 26, 2016
Publication Time: 1.87 months
Copyright Information
© SISEF - The Italian Society of Silviculture and Forest Ecology 2015
Open Access
This article is distributed under the terms of the Creative Commons Attribution-Non Commercial 4.0 International (https://creativecommons.org/licenses/by-nc/4.0/), which permits unrestricted use, distribution, and reproduction in any medium, provided you give appropriate credit to the original author(s) and the source, provide a link to the Creative Commons license, and indicate if changes were made.
Web Metrics
Breakdown by View Type
Article Usage
Total Article Views: 50704
(from publication date up to now)
Breakdown by View Type
HTML Page Views: 41620
Abstract Page Views: 3646
PDF Downloads: 4164
Citation/Reference Downloads: 54
XML Downloads: 1220
Web Metrics
Days since publication: 3498
Overall contacts: 50704
Avg. contacts per week: 101.47
Article Citations
Article citations are based on data periodically collected from the Clarivate Web of Science web site
(last update: Mar 2025)
Total number of cites (since 2016): 29
Average cites per year: 2.90
Publication Metrics
by Dimensions ©
Articles citing this article
List of the papers citing this article based on CrossRef Cited-by.
Related Contents
iForest Similar Articles
Research Articles
Potential spread of forest soil-borne fungi through earthworm consumption and casting
vol. 8, pp. 295-301 (online: 26 August 2014)
Research Articles
Concordance between vascular plant and macrofungal community composition in broadleaf deciduous forests in central Italy
vol. 8, pp. 279-286 (online: 22 August 2014)
Research Articles
Stand structure and deadwood amount influences saproxylic fungal biodiversity in Mediterranean mountain unmanaged forests
vol. 9, pp. 115-124 (online: 08 September 2015)
Short Communications
Culturable fungi associated with wood decay of Picea abies in subalpine forest soils: a field-mesocosm case study
vol. 11, pp. 781-785 (online: 28 November 2018)
Research Articles
Effects of arbuscular mycorrhizal fungi on microbial activity and nutrient release are sensitive to acid deposition during litter decomposition in a subtropical Cinnamomum camphora forest
vol. 16, pp. 314-324 (online: 13 November 2023)
Research Articles
Mapping fungi from below ground: online genetic resources and ectomycorrhizal geographic distributions
vol. 4, pp. 252-255 (online: 13 December 2011)
Research Articles
Dead wood quality influences species diversity of rare cryptogams in temperate broadleaved forests
vol. 9, pp. 276-285 (online: 28 September 2015)
Research Articles
Contribution of environmental variability and ecosystem functional changes to interannual variability of carbon and water fluxes in a subtropical coniferous plantation
vol. 9, pp. 452-460 (online: 25 January 2016)
Review Papers
Soil fungal communities across land use types
vol. 13, pp. 548-558 (online: 23 November 2020)
Review Papers
Linking deadwood traits with saproxylic invertebrates and fungi in European forests - a review
vol. 11, pp. 423-436 (online: 18 June 2018)
iForest Database Search
Search By Author
Search By Keyword
Google Scholar Search
Citing Articles
Search By Author
Search By Keywords
PubMed Search
Search By Author
Search By Keyword