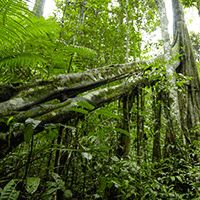
Tree growth, wood and bark water content of 28 Amazonian tree species in response to variations in rainfall and wood density
iForest - Biogeosciences and Forestry, Volume 9, Issue 3, Pages 445-451 (2016)
doi: https://doi.org/10.3832/ifor1676-008
Published: Jan 16, 2016 - Copyright © 2016 SISEF
Research Articles
Abstract
Pole diameter and wood density are variables commonly used in allometric equations to estimate tree biomass and carbon stocks in tropical forests. The effect of variations in tree water content on pole diameters is often disregarded in allometric equations. This study aimed to determine the effect of rainfall seasonality on tree growth, stem wood and bark water content and to assess the relationship between water content and wood density (dry mass to fresh mass volume ratio) in 120 trees from 28 species in a terra-firme rain forest in the central Amazon. In 2006, stem wood and bark water content were gravimetrically determined in the dry season (August-September) and rainy season (April-May). In the same year, growth in diameter was measured at monthly intervals in the 120 trees (DBH ≥ 10 cm) with dendrometric bands previously adapted to the tree. Mean wood water content was lower in the dry season than the rainy season. On the contrary, bark water content was higher in the dry season than in the rainy season. Wood densities higher than 0.75 g cm-3 were found in 64.3% of the trees. Trees with denser woods grew slower and had lower stem water content. Monthly rainfall did not affect tree growth in diameter, which was contrary to our initial expectation on the effect of rainfall seasonality on tree growth in central Amazonia. This finding supports the hypothesis that in central Amazonia, the mild dry season is not long enough to deplete soil water beyond the reach of the root system, which allows the trees to grow at quite constant rates over the year.
Keywords
Amazonia, Allometry Equations, Pole Diameter, Rainfall Seasonality
Introduction
Tree wood density and diameter growth rate at breast height (DBH) are variables commonly used in mathematical models to estimate tree biomass and carbon stocks in tropical forests ([28]). In most allometric models, tree water content is assumed to be constant over the year, and often a constant value is used to convert green tissue into dry matter (biomass). Allometric equations generated by Higuchi et al. ([15]), for example, used a factor of 0.60 to convert fresh tissue into tree biomass. In tropical forests, wood density has high natural variability, which makes it difficult to obtain robust estimates of carbon stocks in biomass ([2], [43]). Tree diameters can be influenced by changes in stem water content ([38], [33], [4]), which can also affect the accuracy of biomass estimation.
Basic wood density (dry mass to fresh volume ratio) can vary among species, tree-to-tree, and even among different parts of the same plant ([28]). It can vary among geographical locations and in response to changes in environmental factors such as soil fertility, temperature and rainfall ([26], [27], [6]). In the Amazon basin, it is believed that wood density can give information about the successional stage of a forest on a given location ([2], [28]). Trees with high wood density have xylem conduits less prone to cavitation and embolism during the dry season ([14], [24]). They also tend to accumulate less water than those with low-density wood ([40], [30]).
Variations in pole water content are mostly related to rainfall seasonality ([12], [5]). Fluctuations in pole diameter are associated with diurnal and seasonal changes in the water content of stem, particularly with variation in the water content of the bark, and to a lesser extent, with sapwood swelling and contraction ([44], [33]).
Variations in the water content of poles reflect their water-storing capacity - tree capacitance ([13], [39], [44]), as the pole can store water to be used when the amount of water lost by transpiration exceeds the water uptake capacity of the tree ([4]). It occurs, for example, during the hottest period of the day or during the dry season of the year. At night, the pole expands and throughout the day there is a progressive contraction due to decline in water content ([29], [41]). During the dry season, the soil water content often declines, and hence it can be expected that the amount of water stored in the pole is also reduced in this season (less water to keep the stomata open for most of the day). Not unsurprisingly, it has been found that in some parts of the Amazon, trees grow slower in the dry season ([32], [42]).
In this study we hypothesized that trees grow faster in the wet season and that bark and wood hold less water in the dry season. The aims of this study were: (1) to determine the effect of monthly rainfall on tree growth in diameter and water content of the bark and stem wood; and (2) to assess the relationship between water content and wood density in tree species of a pristine terra-firme rain forest in central Amazonia.
Materials and methods
Study site, plant material and climate parameters
Data were collected in 2006 at the Tropical Forest Experimental Station (ZF2 reserve - 60° 08′ W, 2° 36′ S) National Institute for Research in the Amazon, State of Amazonas, Brazil. The vegetation is a dense terra-firme forest and the soil is an oxisol with low fertility, clay texture and pH of 4.2 to 4.5 ([20]). The regional climate is tropical humid, with an annual rainfall of 2420 mm ([19]). A total of 120 trees from 28 species (3 to 12 trees per species) with DBH of 10 cm or greater were used in the study (Tab. 1).
Tab. 1 - Mean ± standard deviation of tree growth in diameter (TGD) and wood density (WD, dry mass to fresh mass volume ratio), and the range of diameter of the sampled trees at 1.3 m height (DBH, cm) of the 28 species used in the study. Species and their labels, the botanical family and number of sampled trees per species (N) are also shown.
Species | Label | Family | N | TGD (mm month-1) |
WD (g cm-3) |
DBH (cm) |
---|---|---|---|---|---|---|
Byrsonima duckeana W.R.Anderson | Bdu | Malpighiaceae | 4 | 0.22 ± 0.07 | 0.62 ± 0.02 | 22.5 - 45.7 |
Erisma bicolor Ducke | Ebi | Vochysiaceae | 3 | 0.12 ± 0.05 | 0.56 ± 0.02 | 12.7 - 19.0 |
Eschweilera bracteosa (Poepp. ex O.Berg) | Ebr | Lecythidaceae | 12 | 0.13 ± 0.10 | 0.83 ± 0.01 | 12.0 - 26.0 |
Eschweilera collina Eyma | Eco | Lecythidaceae | 7 | 0.15 ± 0.09 | 0.79 ± 0.01 | 11.1 - 27.0 |
Eschweilera grandiflora (Aubl.) Sandwith | Egr | Lecythidaceae | 3 | 0.13 ± 0.06 | 0.82 ± 0.03 | 19.1 - 46.1 |
Eschweilera pedicellata (Rich.) S.A.Mori | Epe | Lecythidaceae | 5 | 0.08 ± 0.07 | 0.81 ± 0.02 | 12.0 - 48.3 |
Eschweilera sp. | Esp | Lecythidaceae | 4 | 0.09 ± 0.08 | 0.82 ± 0.02 | 13.1 - 27.0 |
Geissospermum argenteum Woodson | Gar | Apocynaceae | 4 | 0.10 ± 0.07 | 0.85 ± 0.01 | 12.0 - 35.9 |
Gustavia augusta L. | Gau | Lecythidaceae | 3 | 0.07 ± 0.08 | 0.77 ± 0.02 | 15.1 - 20.6 |
Inga laurina (Sw.) Willd. | Ila | Fabaceae | 4 | 0.47 ± 0.16 | 0.76 ± 0.03 | 14.1 - 22.5 |
Lacistema aggregatum (P.J.Bergius) Rusby | Lag | Lacistemataceae | 3 | 0.17 ± 0.06 | 0.60 ± 0.02 | 12.5 - 19.0 |
Licania canescens Benoist | Lca | Chrysobalanaceae | 3 | 0.20 ± 0.07 | 0.87 ± 0.03 | 28.0 - 37.0 |
Licania micrantha Miq. | Lmi | Chrysobalanaceae | 5 | 0.13 ± 0.07 | 0.87 ± 0.01 | 14.0 - 34.1 |
Mezilaurus itauba (Meisn.) Taub. ex Mez | Mit | Lauraceae | 5 | 0.07 ± 0.05 | 0.76 ± 0.02 | 10.1 - 21.3 |
Micrandropsis scleroxylon (W.A. Rodrigues) W.A.Rodrigues | Msc | Euphorbiaceae | 3 | 0.10 ± 0.07 | 0.89 ± 0.01 | 28.9 - 40.3 |
Micropholis guyanensis (A.DC.) Pierre | Mgy | Sapotaceae | 4 | 0.16 ± 0.10 | 0.71 ± 0.01 | 17.6 - 40.4 |
Minquartia guianensis Aubl. | Mgu | Olacaceae | 3 | 0.11 ± 0.07 | 0.81 ± 0.03 | 16.1 - 49.8 |
Pourouma tomentosa subsp. apiculada (Spruce ex Benoist) C.C.Berg & Heusden | Pto | Urticaceae | 4 | 0.27 ± 0.09 | 0.42 ± 0.02 | 16.0 - 34.0 |
Pouteria cladantha Sandwith | Pcl | Sapotaceae | 3 | 0.11 ± 0.04 | 0.78 ± 0.13 | 10.1 - 20.0 |
Pouteria guianensis Aubl. | Pgu | Sapotaceae | 3 | 0.10 ± 0.05 | 0.89 ± 0.02 | 22.0 - 64.8 |
Pouteria macrophylla (Lam.) Eyma | Pma | Sapotaceae | 4 | 0.17 ± 0.06 | 0.91 ± 0.02 | 14.2 - 44.0 |
Pouteria sp. | Psp | Sapotaceae | 3 | 0.13 ± 0.05 | 0.79 ± 0.03 | 13.0 - 19.8 |
Protium apiculatum Swart | Pap | Burseraceae | 8 | 0.11 ± 0.04 | 0.63 ± 0.01 | 10.0 - 30.0 |
Protium hebetatum D.C. Daly | Phe | Burseraceae | 3 | 0.10 ± 0.09 | 0.58 ± 0.02 | 11.7 - 13.8 |
Scleronema micranthum (Ducke) Ducke | Smi | Malvaceae | 4 | 0.47 ± 0.22 | 0.65 ± 0.02 | 21.9 - 46.9 |
Swartzia tomentifera (Ducke) Ducke | Sto | Fabaceae | 4 | 0.13 ± 0.04 | 0.81 ± 0.02 | 11.0 - 34.1 |
Tachigali venusta Dwyer | Tve | Fabaceae | 4 | 0.45 ± 0.12 | 0.56 ± 0.02 | 10.1 - 21.3 |
Theobroma sylvestre Aubl. ex Mart. in Buchner | Tsy | Malvaceae | 5 | 0.07 ± 0.04 | 0.71 ± 0.02 | 11.0 - 15.0 |
Climate data were collected using a weather station Li-1401 (Li-Cor, Lincoln, NE, USA) placed at the top of a 40-m tall observatory tower located near the study site (60° 06′ 53″ W, 02° 35′ 21″ S). Air temperature, relative humidity (Humitter 50y, Vaisala Ov, Finlandia) and photosynthetically active radiation (PAR, LI-190SA, Li-Cor, Lincoln, NE, USA) sensors were connected to a datalogger (LI-1400, Li-Cor, Lincoln, NE, USA). Air temperature and relative humidity data were collected at 30-minute intervals and PAR data at intervals of 15 minutes. Rainfall data were collected at 14-day intervals using a conventional pluviometer positioned at the top of the observatory tower. The daily cumulative irradiance (mol m-2 day) was calculated by integrating the instantaneous PAR values over the whole day period. The monthly average of cumulative irradiance was obtained from the daily data.
Wood and bark water content and wood density
In the rainy season (April-May) and dry season (August-September) of 2006, core samples of bark and wood (3 to 5 cm in-length and 5.15 mm in diameter) were collected from the tree poles at 1.3 m from the ground surface (i.e., diameter at breast height - DBH) with an increment borer (Haglöf, Sweden) to determine water content. Stem wood and bark samples were placed into test tubes, and transported to the laboratory for determination of bark and wood fresh mass and fresh wood volume. Wood samples were placed in a forced-air oven and dried at 102 °C until constant mass (about 72 h), and subsequently the dry mass was determined.
Water content (WC) was obtained as the difference between fresh mass (FM) and dry mass (DM) divided by the fresh mass ([30]): WC (%) = 100×[(FM -DM)/FM]. Stem wood samples were collected from each tree in March and April of 2006 and again in April 2007 to determine wood density (dry mass to fresh wood volume ratio). An increment borer (5.15-mm inside tip diameter) was used to obtain the samples, as previously described. These three samples allowed to obtain a representative mean value from each tree. To avoid the potential risk of compressing the bark or wood during the core extraction and to obtain a smooth and uniform core sample, we used an increment borer with a carefully sharpened tip. Volume of the fresh wood sample was determined from the length of the sample and the inside tip diameter of the increment borer. The total length of the wood core was measured with digital calipers.
Tree growth in diameter
In all 120 sampled trees, tree diameter was measured at monthly intervals from January to December 2006, using dendrometric bands, which had been previously installed, four months before the beginning of this study. To determine the relationship between monthly rainfall (total amount of rainfall over a month) and tree growth, for each month, growth data were pooled across species to obtain the mean monthly growth rate of the trees.
Statistical analysis
An analysis of variance (ANOVA) under the assumption of a completely randomized design was used for data analysis. Pearson’s coefficients were calculated for the relationship between monthly rainfall and tree growth, water content and tree diameter, and between tree growth and stem water content and wood density. All statistical analyses were performed with the SAEG 9.0 statistical package from the Federal University of Viçosa (Brazil).
Results and discussion
Climate data
In 2006, total annual rainfall was 2448 mm, which was quite close to the historical mean (2420 mm) for central Amazonia ([19]). Rainfall was less than 100 mm month-1 in the drought season, from August to October. February, April, May and November were the rainiest months (250 to 300 mm month-1). The average temperature was 25.1°C, with an average annual minimum of 22.6 °C and a mean maximum of 28.1 °C. In the driest months, the minimum and maximum temperatures were 23.3 and 30.0 °C, respectively. As expected, the monthly mean cumulative PAR was higher in the driest months, when it was above 30 mol m-2 day-1.
Wood and bark water content
In most of the tree species (26 out of 28), trees had stem wood water content higher in the rainy season than in the dry season, but several species showed an opposite pattern for the water content of the bark (Fig. 1). On average, wood water content was 38.3% in the rainy season and 34.2% in the dry season (p = 0.000, F = 20.4 - Fig. 2a).
Fig. 1 - Water content of wood and bark during the rainy season (black bars) and the dry season (white bars) of 2006. Each bar denotes the mean of several trees within a species. Error bars represents the standard error of the mean. Labels and the number of trees per species are given in Tab. 1. Upper panel - rainy season: F = 2.9, p = 0.000; dry season: F = 5.3, p = 0.000. Lower panel - rainy season: F = 2.0, p = 0.007; dry season: F = 4.1, p = 0.000.
Fig. 2 - Box plot of the water content of wood (A) and bark (B) across the 28 tree species (120 trees) used in the study, in the dry (August-September ) and rainy season (April-May) of 2006. For panel A: F = 20.4, p = 0.000. For panel B: F= 5.7, p = 0.017.
During the rainy season, wood water content of the 28 tree species ranged from 25.5% (M. scleroxylon) to 51.4% (P. tomentosa), while bark water content varied from 31.9% (L. micrantha) to 66.4% (L. aggregatum - Fig. 1). In the dry season, the lowest wood water content was observed in M. scleroxylon (26.3%), and the highest in L. aggregatum (45.4%). On the other hand, mean bark water content was higher in the dry season (46.1%) than in the wet one (42.7%, p = 0.017, F = 5.7 - Fig. 2b). Trees from several species (e.g., E. bracteosa, I. laurina and G. augusta) had higher bark water content in the dry season than in the rainy season.
The stem wood water contents recorded in this study are within the range found by other researchers ([40], [30]). Osunkoya et al. ([30]) found wood water contents varying from 30% (Shorea parviflora) to 50% (Dillenia excelsa, Goniothalamus tapis and Glochidion rubrum). In Borneo, in a study involving 286 species, Suzuki ([40]) found wood water contents ranging from 26 to 76%. In Amazonian trees, Nogueira et al. ([28]) reported wood water content in the range of 22 to 67% (mean of 33.9%), with moisture increasing toward the tip of the tree. Variations in water content of wood and bark have been attributed to environmental and phylogenetic factors, with higher water contents often associated with thicker barks ([34]). In temperate and tropical forests, tree water content of poles and branches can vary seasonally ([12], [5]). In the dry season, and during the driest and warmest periods of the day, transpiration increases ([9], [21]), which may explain the lower water content of the stem wood in the dry season (Fig. 2). This is because the pole shrinks as the water stored in the whole tree, particularly in the pole and branches, is used for transpiration. For example, under the severe stressful conditions of tropical dry forests, the stem diameter of baobab trees (Adansonia spp.) can reversibly contract up to 3 cm by the end of the dry season ([5]). At night and during the rainy season, the stem rehydrates and the cycle starts over again, which leads to diurnal and seasonal fluctuations in pole diameter ([12], [5], [41]).
Contrary to our initial expectations, the bark water content of most trees was either equal or higher in the dry season than in the rainy season (Fig. 1). One explanation can be related to leaf phenology of trees in central Amazonia, since production of leaves and bark water content seem to be interrelated ([7], [34]). In central Amazonia the production of litter tends to increase in the dry season ([17]), which suggests that the production of new leaves can follow a similar pattern ([10]). It has been postulated that stem rehydration is associated with flushing or flowering in tropical dry forests ([3]). The bark often has higher water content than the wood as a result of accumulation of osmotically active products ([8]). Our results are in agreement with those reported by Gibbs ([12]), who found that the water content of the bark can increase when the water content of the wood declines.
On a mass basis, the wood has less water content than the bark because of its main cellular components. Although the sapwood contains parenchyma tissue which can easily expand, the xylem conduits are less prone to contraction and expansion. Indeed, the mature xylem can contract and expand involving elastic deformation (reversible change in shape), but with little exchange of water with the adjacent tissue ([16]). On the other hand, the living components of the bark (phellogen, vascular cambium, phelloderm) may dilate in response to hydration due to the presence of osmotically-active compounds, such as sugars and amino acids. Thus, it seems plausible to conclude that most of the pole contraction occurs in the bark and associated living tissues, such as the sapwood parenchyma ([25], [44], [9]). Therefore, in this work, the rainfall effect on the stem water content could be attributed to a high transpiration rate of the forest canopy in the dry season.
Across species there was no correlation between tree growth in diameter and monthly rainfall throughout the year (p = 0.400, r = 0.05, F = 0.066 - Fig. 3). This finding contradicts our initial expectation on the effect of rainfall on tree growth in central Amazonia. Thus, our results support the hypothesis that in central Amazonia, the typical mild dry season is not too intense to cause detectable reduction in tree growth.
Fig. 3 - Relationship between tree growth and monthly rainfall across the 28 studied species. The black diamond indicates a negative value (see text for discussion). Mean tree growth in diameter was 0.17 mm month-1. Each data point represents the mean growth rate of the 120 sampled trees in a given month of 2006.
Tree growth was faster in some species (e.g., I. laurina, S. micranthum, and T. venusta) than in others (e.g., G. augusta, M. itauba, and T. sylvestre - Tab. 1). This was expected, taken into account the wide biodiversity found in the Amazon. On average, growth rate was 0.17 mm month-1. This value is lower than those observed in eastern Amazonia (about 0.30 mm month-1), but it is within the range of growth rates reported for central Amazonia, from 0.1 to 0.2 mm month-1 (Wagner et al. ([42]). Although from a theoretical point of view it is not possible to record negative growth rates in trees, some trees shrank markedly during the dry season. This explains the negative point (diamond) showed in Fig. 3, and the high variation in tree growth when monthly rainfall was below 200 mm, as the root system of trees can differ in their capability to uptake water from the deep layers of the soil during the drought season.
Irrespective of the season, we did not find any significant relationship between tree size, expressed as DBH, and water content (Fig. 4). At first glance this finding seems to be unexpected because large trees endure more intense transpiration at the hottest times of the day, as they are more exposed to high irradiance and air turbulence above the forest canopy (e.g., wind and thinner boundary layer that allow transpiration to increase). The absence of an effect of tree size on stem water content suggests that large trees are able to uptake water from the deepest layer of the soil profile ([22]), which allows them to keep the stem water content at similar levels as those recorded in smaller trees. However, because of their prominence in the forest canopy, large trees are more prone to diurnal and seasonal variations in diameter ([38], [5]), which seems to occur without affecting the pole water content (Fig. 4). That is, during the periods of intense transpiration the pole volume is reduced in the same proportion as that of the amount of water stored in the pole, which keeps water content constant.
Fig. 4 - Relationship between water content of wood (solid circles) and bark (empty squares) and tree diameter measured at 1.3 m (DBH), in the rainy season (A) and dry season (B) of 2006. Each data point represents one tree. For panel A: F = 0.00 (bark) and F = 0.23 for wood; p = 0.479 (bark) and p = 0.315 for wood. For panel B: F = 1.48 (bark) and F = 0.00 for wood; p = 0.112 (bark) and p = 0.483 for wood.
Water-induced expanding and shrinking of poles pose a major challenge for accurate determination of tree growth in diameter ([1], [37]). Substantial reduction in stem water content during the dry season usually leads to stem shrinkage. It has been estimated that tree diameter may decrease up to 8% as a result of changes in tree water content ([31]). A measurement error of 1% in tree diameter can lead to underestimation (or overestimation) of the total biomass by about 4.0 ton ha-1, assuming a mean stem diameter of about 20 cm and 500-550 trees ha-1; that is, more than 1.0 Pg (carbon) if extrapolated to the entire Amazon basin. To circumvent the effect of seasonal variation in pole water content, it has been suggested that the best time to determine tree growth using allometric equations is during the dry season, when the pole hydration is minimal ([36]).
Wood density and water content
Wood density varied among the 28 species studied, with a mean value of 0.75 g cm-3 (Tab. 1). Tree species that had higher wood densities were: P. macrophylla (0.91 g cm-3), P. guianensis (0.89 g cm-3) and M. scleroxylon (0.89 g cm-3). The lowest wood density was found in P. tomentosa (0.42 g cm-3). Across species, 64.3% of the species had wood densities above 0.74 g cm-3 and only 3.6% of species had wood densities lower than 0.50 g cm-3.
The mean wood density value found in this study is 7% higher than the mean value previously recorded in the Amazon region, about 0.70 g cm-3 ([11], [27]). Our mean wood density values are consistent with the common belief that in the Amazon basin, high wood density values are often found in central Amazonia ([2], [18], [28]). The mean wood density we found in this study is within the range of those found in other tropical forests, usually from 0.21 to 0.80 g cm-3 ([2], [30]).
A negative correlation (r = -0.21, p = 0.050, F = 2.6) was found between wood density and tree growth (Fig. 5). In general, trees with low wood density grow faster than their counterpart with high-density wood ([40], [30]). However, several intrinsic (e.g., genetic make-up of the species) and extrinsic factors (e.g., light and water availability) can affect the growth-wood density relationship; this may explain the low correlation value we found in Fig. 5.
Fig. 5 - Relationship between wood water content and wood density (A) and between tree growth and wood density (B) across the 28 studied species (120 trees). Each data point represents one tree.
The wood water content of the studied species negatively correlated with wood density (r = -0.65, p = 0.000, F = 85.6 - Fig. 5a), which is in agreement with the correlation trend reported by others ([35], [30], [28]). Denser woods have more space filled with cell walls mainly composed of cellulose, hemicellulose and lignin (less porous wood) and, consequently, less water is stored within the stem wood ([28], [23]).
Although the period examined was short (twelve months), the number of trees we measured (n = 120) and the closeness of the annual rainfall in 2006 to the historical regional mean (2448 vs. 2420 mm, respectively), allowed us to conclude that under the rainfall conditions of a typical year (about 2400 mm), the dry season in central Amazonia is not long enough to cause severe soil water depletion at the root zone, which allowed the trees to grow at quite constant rates over the year. More research is needed to elucidate how trees of the central Amazon can respond to water availability if the dry season becomes longer and dryer. Also, the ecophysiological implications of the increase of bark water content in the dry season in the central Amazonia remain to be investigated.
Acknowledgements
The authors thank the Ministry of Science Technology and Innovation (MCTI/INPA), and the Foundation for Research Support of the State of Amazonas (FAPEAM). The Coordination of Improvement of Higher Education Personnel (CAPES) and the National Council for Scientific and Technological Development (CNPq). We also thank the anonymous reviewers for their helpful comments and suggestions.
References
CrossRef | Gscholar
Gscholar
CrossRef | Gscholar
CrossRef | Gscholar
Authors’ Info
Authors’ Affiliation
Forest Ecology and Ecophysiology Laboratory, Federal University of Goiás/Jataí, GO (Brazil)
Tree Ecophysiology Laboratory, Coordination of Environmental Dynamic, National Institute for Research in the Amazon, Manaus, AM (Brazil)
Corresponding author
Paper Info
Citation
Dias DP, Marenco RA (2016). Tree growth, wood and bark water content of 28 Amazonian tree species in response to variations in rainfall and wood density. iForest 9: 445-451. - doi: 10.3832/ifor1676-008
Academic Editor
Vicente Rozas
Paper history
Received: Apr 15, 2015
Accepted: Aug 24, 2015
First online: Jan 16, 2016
Publication Date: Jun 01, 2016
Publication Time: 4.83 months
Copyright Information
© SISEF - The Italian Society of Silviculture and Forest Ecology 2016
Open Access
This article is distributed under the terms of the Creative Commons Attribution-Non Commercial 4.0 International (https://creativecommons.org/licenses/by-nc/4.0/), which permits unrestricted use, distribution, and reproduction in any medium, provided you give appropriate credit to the original author(s) and the source, provide a link to the Creative Commons license, and indicate if changes were made.
Web Metrics
Breakdown by View Type
Article Usage
Total Article Views: 51158
(from publication date up to now)
Breakdown by View Type
HTML Page Views: 43042
Abstract Page Views: 3297
PDF Downloads: 3502
Citation/Reference Downloads: 40
XML Downloads: 1277
Web Metrics
Days since publication: 3465
Overall contacts: 51158
Avg. contacts per week: 103.35
Article Citations
Article citations are based on data periodically collected from the Clarivate Web of Science web site
(last update: Mar 2025)
Total number of cites (since 2016): 27
Average cites per year: 2.70
Publication Metrics
by Dimensions ©
Articles citing this article
List of the papers citing this article based on CrossRef Cited-by.
Related Contents
iForest Similar Articles
Research Articles
Comparison of alternative harvesting systems for selective thinning in a Mediterranean pine afforestation (Pinus halepensis Mill.) for bioenergy use
vol. 14, pp. 465-472 (online: 16 October 2021)
Research Articles
Deploying an early-stage Cyber-Physical System for the implementation of Forestry 4.0 in a New Zealand timber harvesting context
vol. 17, pp. 353-359 (online: 13 November 2024)
Research Articles
Identification of wood from the Amazon by characteristics of Haralick and Neural Network: image segmentation and polishing of the surface
vol. 15, pp. 234-239 (online: 14 July 2022)
Research Articles
Allometric equations to estimate above-ground biomass of small-diameter mixed tree species in secondary tropical forests
vol. 13, pp. 165-174 (online: 02 May 2020)
Research Articles
NIR-based models for estimating selected physical and chemical wood properties from fast-growing plantations
vol. 15, pp. 372-380 (online: 05 October 2022)
Short Communications
Effect of intensive planting density on tree growth, wood density and fiber properties of maple (Acer velutinum Boiss.)
vol. 9, pp. 325-329 (online: 22 October 2015)
Research Articles
Characterization of VOC emission profile of different wood species during moisture cycles
vol. 10, pp. 576-584 (online: 08 May 2017)
Research Articles
Examining the evolution and convergence of wood modification and environmental impact assessment in research
vol. 10, pp. 879-885 (online: 06 November 2017)
Research Articles
Physical, chemical and mechanical properties of Pinus sylvestris wood at five sites in Portugal
vol. 10, pp. 669-679 (online: 11 July 2017)
Research Articles
Mechanical and physical properties of Cunninghamia lanceolata wood decayed by brown rot
vol. 12, pp. 317-322 (online: 06 June 2019)
iForest Database Search
Search By Author
Search By Keyword
Google Scholar Search
Citing Articles
Search By Author
Search By Keywords
PubMed Search
Search By Author
Search By Keyword