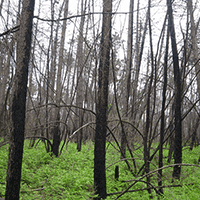
Impact of wildfire on the edaphic microarthropod community in a Pinus pinaster forest in central Italy
iForest - Biogeosciences and Forestry, Volume 8, Issue 6, Pages 874-883 (2015)
doi: https://doi.org/10.3832/ifor1404-008
Published: May 05, 2015 - Copyright © 2015 SISEF
Research Articles
Abstract
The purpose of this study was to investigate the response of the soil microarthropod community to wildfire in forest ecosystems. The edaphic microarthropod communities of pine stands burned in 2001, in 2009, both in 2001 and in 2009, in 2012 were compared to an area never burned. Sampling was conducted in the spring and autumn of 2011 and 2012 in the areas burned in 2001, in 2009 and those never burned, while in the area burned in 2012, soil samples were collected in March (10 days after fire), June and September. The abundance and biodiversity of the microarthropod community were assessed. A multitemporal analysis was also carried out to assess the effect of fire on soil microarthropod abundance 5 months, 2-3 years and 10-11 years after fire. The results showed that the abundance of edaphic microarthropod communities decreased dramatically in areas burned twice, but also in areas burned once in 2009 and five months after the fire in the area burned in 2012. Different taxonomic groups did not seem to respond to fire in the same way, some taxa being more sensitive than others. Pseudoscorpionida decreased in both the short- and the long-term, while Diplopoda, Thysanoptera and Symphyla showed a reduction in the intermediate- and long-term. In the short-term, Diptera and Coleoptera larvae appeared to be the most influenced taxonomic groups. Our study shows that biomonitoring is a valuable tool to investigate the reaction of forest ecosystems to fire, and that edaphic microarthropods can provide interesting answers about the direct and indirect effects of fire on soil.
Keywords
Biomonitoring, Microarthropod Community, Forest Fires, Pinus pinaster Aiton
Introduction
Understanding ecosystem responses to disturbance at different scales is fundamental to develop sound strategies for forest management and nature conservation ([42]). To this end, biomonitoring - i.e., the analysis of the reactive components of ecosystems in response to environmental changes to assess deviations from the normal situation ([80], [60]) - and ecological indicators can be used to quantify the magnitude of stress, the degree of exposure or the ecological response to stresses ([18]), thereby providing a simple and efficient method to assess the composition, structure and function of complex ecological systems ([36]).
Fire is one of the most important disturbances in many natural and semi-natural ecosystems worldwide ([27]), including the Mediterranean Region ([65]). Fire also has an ecological role in influencing ecosystem biodiversity and guaranteeing the conservation of a variety of habitats ([28], [54], [7]) and changing landscapes ([25]). The soil biota is one of the first soil components to respond to fire exposure, as fire alters the species composition and decreases the zoocenotic density ([6]).
Fire may have direct or indirect effects on soil fauna. One of the principal direct impacts on the edaphic microarthropod community is the exposure of forest fauna to high temperatures, which can cause alterations in species composition and decrease the abundance of some taxonomic groups ([76], [20], [6]). Contrastingly, some authors claimed that the direct effects of fire on most soil vertebrates are minimal ([68], [58]). Indirect fire impacts include changes in habitat and food availability for these organisms. After a fire, alterations of hydrothermal conditions, litter mass and pH have an impact on animal survival, particularly the stenoecious species which suffer high temperatures as well as light and moisture extremes, with subsequent habitat loss ([69], [6]). While some changes of the edaphic community can be irrelevant in the short-term after fire, the subsequent post-fire, aboveground succession can determine changes in soil fauna, affecting the age and trophic structure of soil animals ([6]).
Edaphic microarthropods play an important role in regulating the rate of decomposition ([87], [74]) and nutrient cycling ([31]) through interactions with the microbial and fungal communities ([74], [75], [45]), and they also contribute to soil structure and humus formation ([87], [62], [81]). For all these reasons, the preservation of soil biodiversity should be considered an integral component of forest management practices ([53]). Therefore, understanding the reaction of a forest to fire and, in particular, how it impacts edaphic microarthropods is important, even though subtle variations in the structure of edaphic communities and their spatial distribution have been given little consideration in the macroscopic approach to ecosystem functionality ([31]).
Biological indicators are often more sensitive to change than other indicators, and they could be used in environmental monitoring and assessment ([3]). However, studies on the impact of fire on edaphic microarthropod communities are still limited and focus mainly on the two most numerous groups in soil, such as mites (Acari) and springtails (Collembola - [21], [13], [26], [50], [51], [32], [16], [17]). Studies on the impact of fire on edaphic microarthropod communities that consider other taxa are few, and they are mostly limited to cases of prescribed fire ([88], [55], [15], [6], [52], [86], [78]). It is also worth noting that many of these experiments are based on data simulated in the laboratory, while the number of studies based on real data collected in the forest is low, and (at least in the Mediterranean region) such studies have focused on a relatively small number of taxa. For instance, some authors have studied the effect of fire on only one taxon, such as Myriapoda ([72], [24], [82], [84]) or edaphic carabids ([71]), while few studies have examined multiple edaphic groups ([11], [76], [10]).
In this study, we investigated the response to fire of the whole edaphic microarthropod community in Mediterranean pine stands. We also conducted a multitemporal analysis to assess the effect of fire frequency on soil microarthropod abundance. The aims were: (1) to monitor microarthropod abundance and the shift in biodiversity in forest areas burned in 2001, 2009 and 2012; (2) to assess the impact of fire frequency on the microarthropod community composition in forest areas burned in 2001 and again in 2009; (3) to evaluate the microarthropod composition recovery over time, taking into account three time intervals after the fires, hereafter indicated as short- (5 months), intermediate- (2-3 years) and long- (10-11 years) term intervals.
Materials and methods
Study area
The experimental site is located in central Italy (Tuscany) in the hilly district of Cerbaie, 50 km east of Pisa, including the Montefalcone National Nature Reserve (43° 44′ 35″ N, 10° 42′ 25″ E) and a surrounding area (43° 44′ 08″ N, 10° 41′ 51″ E - Fig. 1).
The area is characterized by a temperate climate ([41], [73]) with a 14-15 °C mean annual temperature and 1000-1150 mm mean annual precipitation, concentrated in autumn and winter i.e., it has a humid and sub-humid climate type according to Thornthwaite’s classification ([83], [73]). Altitude varies between 45 and 114 m a.s.l. The study areas were located on quite flat terrain with very limited altitudinal differences (less than 20 m among the areas). Prevailing winds in the area are from the north and southwest. The geological setting is a sand and coastal conglomerate (Pliocene), and soils are loose, medium-deep, with a mainly acidic reaction, and variable in texture, drainage and pore space ([63]).
Forest cover is dominated by the maritime pine (Pinus pinaster Aiton) in the higher zones and mixed oaks (Quercus cerris L. and Q. petraea Liebl.) in the lower and more humid areas. Coniferous stands are 80-85 years old, with pine in the dominant layer and butcher’s broom (Ruscus aculeatus L.), holly (Ilex aquifolium L.), cornelian cherry (Cornus mas L.), heathers (Erica spp.), juniper (Juniperus communis L.), brambles (Rubus spp.), brooms (Cytisus scoparius (L.) Link), laurel (Laurus nobilis L.), cistus (Cistus spp.) and ferns (Pteridium aquilinum (L.) Kuhn) in the shrub layer.
In the last several years, three fires have occurred in the area. About 70 hectares (ha) of pine forest burned in August 2001; in August 2009 another fire burned about 130 ha of pine forest, including 70 ha already burned in 2001 ([61]). In March 2012, a fire burned 15 ha of pine forest close to the areas burned in 2001 and 2009 (Fig. 1). Data about the duration of the fires, the burned areas, and burned biomass were registered by the National Forest Service (Tab. 1). The fires spread mainly as passive and active crown fires involving duff, litter, shrubs and the tree canopy. Detailed data on fire severity in the study areas were not available, but based on direct field observations by fire managers, it was possible to estimate that the severity of all fires ranged from “moderate or severe surface burn” to “deep burning or crown fire”, according to Keeley ([38]). In the area burned in 2001, natural pine regeneration (around 10 years old) has set in, together with many shrubs and ferns. In the area burned in 2009, there are burned pine snags and ferns. The areas burned both in 2001 and in 2009 are covered mainly by nitrogen-fixing species, such as brooms (C. scoparius Link), cistus (Cistus spp.), heathers (Erica spp.) and fire moss (Ceratodon purpureus Brid.). In the area burned in 2012, there are numerous fire-damaged trees and dead trees, with the understorey completely destroyed by fire; the terrain is covered by grey and white ash (Fig. 2).
Tab. 1 - Duration, burned area and burned biomass of the forest fires where the impact on edaphic microarthropod communities was analysed. (na): not available.
Fire | Burned area (hectares) | Duration (hour) |
Wood biomass burned (m3) |
---|---|---|---|
August 2001 | 70 | 47.0 | 16 200 |
August 2009 | 130 | 53.0 | na |
March 2012 | 15 | 3.5 | na |
Fig. 2 - View of the burned and unburned areas analyzed in this study. (A): area burned in 2001; (B): area burned in 2009: (C), area burned twice in 2001 and in 2009; (D): area burned in 2012; (E): area not affected by fire (control area).
Ground data
Sampling was conducted in the following areas:
- an area that burned in 2001 (A);
- an area that burned in 2009 (B);
- an area that burned twice, in 2001 and again in 2009 (C);
- an area that burned in 2012 (D);
- an area not affected by fire (Control E).
In each area, two square plots (7.1 × 7.1 m) were placed so as to be homogeneous in terms of site aspect, forest cover and soil characteristics. In the area burned twice (C), two sub-areas with very similar types of vegetation (C1 and C2) were selected to provide more representative information of the area’s apparent higher soil variability, and also because large surfaces burned in 2001 and in 2009. In each sub-area, two plots were etablished (Fig. 1). In each plot, three 10 × 10 × 10 cm soil samples were collected along a 10-m transect (placed along the plot diagonal) for the identification of edaphic microarthropod communities. The x, y coordinates of all sampling points (36) were recorded using a GPS receiver Trimble Juno 3B Handheld with 2-5 m positional accuracy.
Soil samples were collected in the spring and autumn of 2011 and 2012 for sampling areas A, B, C and control E, and at three different dates after the fire in the area that burned in 2012 (D - Tab. 2). The soil layer was also analysed for carbon content and pH in all plots by collecting three randomly selected samples. The samples were collected using a metal cylinder (10-cm long with a 5-cm inner diameter) after litter removal. Soil temperature and moisture were measured at a depth of 10 cm using a moisture meter (HH2 - Moisture Meter - Readout Unit; Delta-T Devices) after sample collection.
Tab. 2 - Dates of sampling in each treatment.
Sampling area | Sampling dates | ||||||
---|---|---|---|---|---|---|---|
2011 | 2012 | ||||||
Spring | Autumn | Spring | Autumn | 23 Mar | 25 Jun | 18 Sep | |
A (burned in 2001) | - | × | × | × | - | - | - |
B (burned in 2009) | × | × | × | × | - | - | - |
C (burned in 2001 and 2009) | × | × | × | × | - | - | - |
D (burned in March 2012) | - | - | - | - | × | × | × |
E (control - never burned) | × | × | × | × | - | - | - |
Data Analysis
Laboratory analysis
Edaphic microarthropods were extracted from soil samples using a Berlese Tüllgren funnel ([5], [85]) with an exposition of 7 days; then microarthropods were preserved in 75% ethanol (v/v). Extracted soil fauna were counted and identified to the Order taxonomic level using a stereomicroscope with a magnification of 40×.
The soil pH and carbon content were also estimated. The soil pH was determined with pH-meter using a suspension of 10 g of soil and water, with a soil solution ratio of 1:5. The carbon content was assessed using the Walkley-Black method ([59]).
Abundance of edaphic microarthropods
The number (N) of microarthropods in each taxon was expressed as Nm-2 and then normalized as ln x + 1 ([35], [23]).
In each plot, the average number of microarthropods was computed from the soil samples (3 per plot). Then, in each sampling area (A, B, C, D and control E), the mean number of microarthropods was estimated using soil samples from two plots per area.
Statistical analysis
Effect of fire on edaphic microarthropod abundances
The effect of fire on the microarthropod community was assessed with univariate and multivariate analysis of variance (ANOVA) using the PAST Programme ver. 2.17 ([29]). In the area burned in 2012 (D), differences in edaphic microarthropod abundances in relation to time after fire (10 days, three months, five months) and to the control were investigated using one-way ANOVA.
A t-test was used to determine whether the abundances of individuals were significantly different in the different sampling seasons and years from fires in areas A, B, C and E.
The non-parametric Kruskal-Wallis test ([43]) was used to evaluate differences in edaphic microarthropod abundance between sampling areas. In case of statistically significant differences, the Mann-Whitney post-hoc comparison test was used and the Bonferroni correction applied ([8], [9]).
Variations in the abundance of the edaphic microarthropod community data between areas burned once, twice and not burned were investigated using Principal Component Analysis (PCA - [19], [30]), which was conducted on a rectangular matrix of abundance (sample area × taxon) of each microarthropod taxon. To evaluate the number of informative axes, a bootstrap resampling technique with 1000 replicates was used ([33]). The significance of the eigenvector coefficients was evaluated by determining the 95% confidence limits.
The SIMPER analysis ([14]) using the Bray-Curtis similarity measure was applied to quantify the contribution of each taxon to the dissimilarity between groups of samples. Then, the groups of taxa that most contributed to the dissimilarity were checked using the Kruskal-Wallis test to assess whether fire influenced the increase and/or decrease in density of these groups.
Linear regression analysis was used to investigate the relationship between physical (carbon content, moisture and temperature) and chemical (pH) soil characteristics and the abundance of edaphic microarthropods for all sampling seasons.
Biodiversity of the edaphic microarthropod community
Biodiversity of the edaphic microarthropod community was evaluated using Shannon’s index, the evenness index and Simpson’s index.
Shannon’s index ([77]) was based on the number of microarthropods in a taxon and the total number of individuals in a sample ([46]). The evenness index was calculated using the Pielou’s index ([66]), which shows how the taxa are equally abundant ([46]). Simpson’s index ([79]), which describes the variance in the distribution of specific abundances ([47]), was used to assess the dominance of taxa.
The Kruskal-Wallis test was applied to compare the biodiversity indexes between the different areas and sampling seasons.
Results
Intermediate- and long-term fire effects on edaphic microarthropod abundances
To assess the intermediate- and long-term effects of fire on edaphic microarthropod abundance, data for the areas burned in 2001 (A), in 2009 (B) and in 2001 and 2009 (C) were compared with the control (E). The total number of soil microarthropod taxa (Tab. S1 in Appendix 1) was 22 in the control area, 22 in the area burned only in 2001 (A) and 20 in the area burned only in 2009 (B). In the area burned twice, 23 taxa were recorded in sub-area C1 and 19 taxa were recorded in sub-area C2. The highest number of individuals (Nm-2) was found in the control (sampling date: Autumn 2011), while the lowest number was found in sub-area C2 (sampling date: Spring 2011 - Fig. 3).
Fig. 3 - Abundance of microarthropods recorded in forest areas burned once or twice and after the intermediate- and long-term after fire. Error bars represent the standard deviation. (A): area burned in 2001; (B): area burned in 2009; (C): area burned in 2001 and in 2009; (E): control area.
Linear regression analysis of the microarthropod abundances and the chemical and physical soil characteristics did not show significant relationships. Only the sampling area burned in 2012 showed a significant linear regression (p=0.01) between abundance and carbon content, with a coefficient of determination of 0.69.
In all cases, Acari and Collembola were the dominant taxonomic groups. In the control area, Acari accounted for 43% of the total number, and in the burned areas they exceeded 50% in all cases. Collembola accounted for approximately 40% of the total number in the areas C1 and C2.
The t-test showed that the total abundances did not differ significantly between sampling seasons and between fire years, while significant differences were detected after the Kruskall-Wallis test between the abundances in the control area and those in the areas burned once and twice (A, B and C), with an Hc of 18.26 (df = 4, p < 0.001). In the area burned in 2009 (B) and in the sub-areas burned twice in 2001 and 2009 (C), microarthropod abundances were significantly lower (post-hoc Mann-Whitney test: p < 0.01). Microarthropod abundances in B and C were significantly different from those found in the area burned in 2001 (A). In contrast, area A did not significantly differ from the control (p = 0.6). In sub-area C1, the repeated passage of fire significantly influenced all edaphic microarthropod biodiversity indexes, i.e., the equitability of the proportional abundance of species (evenness p = 0.046), the species richness (Shannon p = 0.002) and the dominance (Simpson p = 0.012); however, in sub-areas C1 and C2, we found significant differences only for the Shannon’s index (p = 0.025 - Tab. 3).
Tab. 3 - Biodiversity indexes (± standard deviation) of edaphic microarthropod communities recorded in each treatment and results of the Kruskall-Wallis test (Hc, df = 4) for differences between the control (E) vs. the areas burned once (A and B), and vs. the areas burned twice (C1 and C2). Significant differences after the Mann-Whitney test are indicated. (*): p<0.05; (**): p< 0.01; (ns): not significant; (A); area burned in 2001; (B): area burned in 2009; (C1, C2): areas burned in 2001 and in 2009.
Areas | Shannon’s Index | Simpson’s Index | Evenness Index |
---|---|---|---|
E | 1.3 ± 0.2 | 0.6 ± 0.1 | 0.5 ± 0.1 |
A | 1.2 ± 0.1 ns | 0.6 ± 0.0 ns | 0.4 ± 0.1 ns |
B | 1.2 ± 0.1 ns | 0.6 ± 0.0 ns | 0.5 ± 0.0 ns |
C1 | 0.9 ± 0.2 ** | 0.5 ± 0.1 * | 0.4 ± 0.1 * |
C2 | 1.1 ± 0.2 * | 0.6 ± 0.1 ns | 0.5 ± 0.0 ns |
Kruskal-Wallis Hc | 15.82 ** | 9.22 * | 8.54 ns |
The multivariate PCA also showed that the repeated passage of fire can play an important role in influencing the abundance of microarthropod community, some taxa being affected more than others. Overall, the first two principal components accounted for 41% of total variance in the edaphic microarthropod abundance; eigenvalues for the first and second PC axes were 29.16 and 9.63, respectively (Fig. 4). The first PC axis clearly reflected a gradient of fire disturbance. Indeed, data from the control area and from that burned in 2001 (A) all showed large PC1 scores (right side of Fig. 4), whereas the areas C1 and C2 (burned twice) and B (burned in 2009) tended to group on the left , except for three samples taken in 2012. PC1 was mainly related to the abundance of Symphyla, Diplopoda and Pseudoscorpionida, while PC2 was associated with the abundance of Diptera (adults and larvae), Hymenoptera (excluded ants) and Psocoptera. In fact, a remarkable average dissimilarity between the total abundance in the control and in areas C1 and C2 (35% and 40%, respectively) was found using the SIMPER procedure. The most influenced taxonomic groups were Diplopoda, Thysanoptera, Pseudoscorpionida and Symphyla. Three years after fire, the difference between abundances in the control E and the area burned in 2009 (B) was 27%, the most affected taxa being Thysanoptera, Symphyla and Pseudoscorpionida. The abundances of Symphyla and Pseudoscorpionida were significantly reduced (Kruskal-Wallis test, p < 0.001) both in B and C following fire, whereas the number of Thysanoptera decreased in all areas affected by fire (A, B, C) with p < 0.001. The number of Diplopoda was significantly reduced in the area burned twice (C1 and C2, p < 0.001 - Tab. 4).
Fig. 4 - Principal component analysis (PCA) in the intermediate- and long-term after fire. (A): area burned in 2001; (B): area burned in 2009; (C): area burned in 2001 and in 2009; (E) control area.
Tab. 4 - Abundance (N m-2 ± standard deviation) of the main sol microarthropods recorded in each treatment and results of the Kruskall-Wallis test (Hc) for differences between the control (E) vs. the areas burned once (A and B), and vs. the areas burned twice (C1 and C2). Significant differences after the Mann-Whitney test are indicated. (***): p<0.001; (ns): not significant.
Areas | Pseudoscorpionida | Symphyla | Thysanoptera | Diplopoda |
---|---|---|---|---|
E | 141 ± 97 | 946 ± 608 | 592 ± 835 | 271 ± 246 |
A | 78 ± 89 ns | 17 ± 31 ns | 6 ± 13 *** | 200 ± 343 ns |
B | 17 ± 31 *** | 304 ± 217 *** | 42 ± 71 *** | 146 ± 232 ns |
C1 | 12 ± 25 *** | 17 ± 31 *** | 4 ± 12 *** | 0 ± 0 *** |
C2 | 0 ± 0 *** | 92 ± 94 *** | 8 ± 15 *** | 13 ± 35 *** |
Kruskal-Wallis Hc | 24.07 *** | 26.53 *** | 23.23 *** | 20.32 *** |
Short-term fire effect on microarthropod abundance
The short-term fire effect on microarthropod abundance was assessed using data from the area burned in March 2012 (D). As compared with the control, a reduction of the number of taxa by 7-, 17- and 14-fold was observed in this area after ten days, three months and five months after fire, respectively (Tab. S1 in Appendix 1). Ten days after fire, the total number of microarthropods was 13 633, while 8 316 and 16 649 individuals were counted after three and five months, respectively (Fig. 5).
Fig. 5 - Abundance of microarthropods recorded in the short-term after fire. Error bars represent the standard deviation. Sampling date: March, June and September 2012.(D): area burned in 2012; (E): control area.
Even at such a short time after fire, there was no significant relationship between the abundance of edaphic fauna and soil moisture and temperature. The decrease in carbon content in the first five months after fire showed a significant direct relationship to the number of microarthropods, whereas the relationship between pH and the abundance of soil fauna was not significant.
After the 2012 fire, Acari and Collembola were the most represented taxonomic groups in the intermediate- and long-term after fire. Ten days after fire, their percentages were very high, with Acari and Collembola accounting for 81% and 5% of all microarthropods, respectively. After three months, the percentage of Acari was still high (72%), but after five months, their abundance returned to values comparable with those of the control.
The t-tests confirmed that the abundance of individuals did not differ significantly five months after fire, and the ANOVA showed a significant decrease in edaphic microarthropod abundance between the control area and the area burned in 2012 (F=10.87, p<0.001).
The first two PC axes accounted for 53% of the total variation in the dataset (PC1: 32%, PC2: 21% - Fig. 6). The eigenvalues for the first axis and second axis were 29.09 and 19.53, respectively. Samples from the unburned area (E) showed large PC1 scores, grouping to the rightmost part of the biplot of Fig. 6 (except for the soil sample taken in the autumn 2012), while those from the area burned in 2012 were located to the left. The soil samples taken three months after fire appear clustered together in the negative semi-axis of both components (PC1, PC2), indicating a dramatic effect of fire on the abundance of the edaphic microarthropod community. PC1 was mostly related to the abundance of both Coleoptera and Diptera larvae, Diplura, Diplopoda, Symphyla and Pseudoscorpionida, whereas PC2 was mainly associated to the abundance of Pseudoscorpionida, Diptera larvae, ants and Symphyla.
Fig. 6 - Principal component analysis (PCA) in the short-term after fire. (D): area burned in 2012; (E): control area.
The SIMPER analysis highlighted a high dissimilarity percentage between the control and the burned area three months after fire (31%), decreasing to 28% after five months. Symphyla, Pseudoscorpionida, the larvae of Coleoptera and ants were the most affected microarthropods three months after fire, but after five months the Diplopoda and Pseudoscorpionida were the most reduced taxa. Immediately after fire (10 days), the dissimilarity was 28% due to the non-significant reduction (p>0.05) in the number of Diptera, Diplura and Symphyla larvae. The density of Pseudoscorpionida was significantly reduced ten days after fire (Kruskal-Wallis test: p < 0.05), and we did not find this taxonomic group in the soil sampled three and five months after fire. Biodiversity, as expressed by the Shannon, evenness and Simpson indexes, did not show significant differences after Kruskal-Wallis test between the control area E and the area burned in 2012 (ten days, three months and five months after fire).
Discussion
As often occurs in wildfire research, we had limited control over our experimental design; in particular, no replicates in other wildfire areas were available. Nonetheless, studying wildfires may provide critical information that can be difficult to obtain from experimental burns, since wildfires typically occur under more extreme conditions ([67]). To overcome this limit, sampling was repeated three times on two plots, with three samples per plot.
Our results show that fire in maritime pine forests significantly affects edaphic microarthropod communities, both in the medium-to long-term and in the short-term after fire. Repeated and frequent fires in the same area (in this case, two fires over a period of eight years) had produced significant changes in the soil fauna.
Similar studies carried out in different forest habitats and after different intervals of time after fire have shown a reduction in soil arthropod abundance ([2], [11], [40], [10], [90], [82], [12], [6], [52]). According to other authors, the arthropod community can show some resilience to fire ([48], [49], [1], [64], [55], [56], [57]).
After fire, the most abundant groups are usually mites and springtails ([11], [15]). In our study, changes in edaphic communities caused by wildfire were observed in relation to both time after fire and fire frequency. Microarthropod communities tended to recover within 10 years since burning. In the area burned twice (2001 and 2009), the most sensitive taxonomic groups were Pseudoscorpionida, Symphyla, Thysanoptera and Diplopoda, which remarkably decreased their abundance. In the short-term after fire (five months), Diplopoda were significantly reduced, while the total number of centipedes, Araneidae and Isopoda was not influenced by fire. Similarly, Abbott ([1]) found that millipedes were the least abundant taxonomic group one year after fire in the Jarrah Forest (western Australia). In our study, the Diplopoda community recovered two years after fire. Furthermore, a study on centipedes in the Mediterranean region ([84]) showed a reduction of the abundance of this taxon due to surface fire, while the centipede community composition did not seem to be affected by crown fire.
Our results show that the most sensitive taxonomic groups in the short-term after fire included organisms that play an active role in the soil only during their larval stage (Coleoptera and Diptera larvae), as well as Pseudoscorpionida. Probably 10 days after fire, soil conditions were not suitable for the development of Diptera and Coleoptera larvae, which repopulated the soil later. Pseudoscorpionida were the only taxonomic group affected by fire both two years after fire and in areas burned twice. They were also influenced in the short-term, confirming their high sensitivity to fire, as observed by York ([90]) in Australian blackbutt forests.
In our study, fire affected some taxonomic groups (Diplopoda, Symphyla, Chilopoda, Protura, Diplura, Pseudoscorpionida) more than others which appeared more resilient (Isoptera and Blattoidea), as revealed by the results of the PCA. Some of these organisms, e.g., Symphyla, generally characterize undisturbed soil ([4]) where they may deeply influence the soil ecosystem regardless of their abundance ([44]). It has been reported that the Chilopoda community undergoes deep structural changes after fire ([84]). Their observed decrease after fire was not significant in this study, likely because these organisms usually migrate into the deeper soil layers to find more suitable conditions for survival ([76]).
The principal component analysis carried out revealed remarkable differences in the soil microarthropod community between burned and unburned areas both in the medium- to long- and in the short-term after fire. In the long term, the effects of fire on such communities and their microhabitat are mainly indirect, probably due to changes in forest cover. Ten year after fire, the numerical strength of microarthropods was comparable to that observed in the unburned area, likely due to the local restoration of suitable environmental conditions in a natural-regenerated stand of maritime pine and broadleaves re-established after fire.
In the short-term, the effect seems mainly direct, e.g., soil microarthropods were probably killed by fire and heat, rather than by indirect effects, as pointed out by Malmström et al. ([52]) for mites, springtails and Protura. Five months after fire, the microarthropod communities had still not totally recovered compared with the control area.
The biodiversity of the edaphic microarthropod communities showed significant changes in the area burned twice, as revealed by the stronger influence of fire on species richness than on equitability and dominance. In the short-term after fire, biodiversity indexes were comparable to the situation before fire occurrence.
Even if the abundance of soil microarthropods is often correlated to soil conditions ([89]), we did not detect significant relationships between microarthropod density and soil chemical and physical characteristics, both in the intermediate-long term and in the short-term after fire, except for the decrease of carbon content in the first five months after fire. The increase in soil alkalinity caused by ash just after fire ([70], [39]), did not seem to affect edaphic microarthropod abundance. The observed restoration of soil pH to the pre-fire level within five months is in agreement with the results by Jalaluddin ([34]). According to Fernandez et al. ([22]), the stored carbon content, which decreased in the first three months after fire, returned to the control level two years later, and it did not affect the abundance of soil microarthropods. According to Kautz et al. ([37]), the observed decrease in microarthropod abundance in the short-term after fire can be determined by changes in the immediate supply of food rather than by soil chemical parameters, such as carbon or pH.
It is worth to notice that only high taxonomic levels (Orders or Families) were considered in this study. Indeed, the analysis of the microarthopod biocoenosis at lower taxonomic levels could highlight further differences between genera or species. Further studies are needed to investigate such possible differences.
Conclusions
Monitoring the soil microarthropod community is an important tool for better understanding the effects of fire on forest ecosystems. Our results show that a more efficient assessment of fire impacts could be achieved by surveying all microarthropod taxa rather than monitoring only chemical or physical soil parameters. Edaphic microarthropods are effective bio-indicators of the environmental change; however, the interactions of biological, chemical and physical aspects should be always monitored to obtain a broader picture of soil forest ecosystems.
After a wildfire, the natural evolution of forest structure and composition can also affect soil components, leading to habitat conditions unsuitable for the microarthropod community. Some taxonomic groups (e.g., Pseudoscorpionida) are more sensitive to fire and/or repeated fire passes than others, and this can produce long-lasting transformations of the microarthropod community. In our study, such transformations were still evident two years after the last fire.
Despite the number of studies on the soil microarthropod communities after fire in the literature, their sampling methods are often different, thereby their results are often difficult to compare. Moreover, usually only a limited number of taxonomic groups are considered in different forest habitats and after different fire conditions. The analysis of whole microarthropod communities, such as that carried out in this study, are much fewer and need further research effort. To this regard, it would be useful to study the relationship between microarthropods and other soil organisms (e.g., fungi, microorganisms) to throw light on the influence of fire on the soil ecosystem and trophic chains, as previously reported by Murunga ([57]) for soil arthropods in Kenya.
Our work indicates that monitoring the effects of fire on the whole edaphic microarthropod community in forest habitats can provide useful information for better management and rehabilitation of forest ecosystems impacted by fire.
Acknowledgements
We wish to thank Dr. Elena Perilli of the Italian National Forest Service (Corpo Forestale dello Stato) for supporting this research in the Montefalcone National Nature Reserve.
References
Gscholar
Gscholar
Gscholar
Gscholar
Gscholar
Gscholar
Gscholar
Gscholar
Gscholar
Gscholar
Gscholar
Online | Gscholar
Gscholar
Gscholar
Gscholar
Gscholar
Gscholar
Gscholar
Gscholar
Gscholar
Gscholar
Gscholar
Gscholar
Gscholar
Supplementary Material
Authors’ Info
Authors’ Affiliation
Susanna Nocentini
Enrico Marchi
Francesca Bottalico
Silvia Fiorentini
Davide Travaglini
Department of Agricultural, Food and Forestry Systems, University of Florence, v. San Bonaventura 13, I-50145 Florence (Italy)
Department of Agriculture, Food and Environmental Sciences, University of Florence, p.le delle Cascine 28, I-50144 Florence (Italy)
Corresponding author
Paper Info
Citation
Lisa C, Paffetti D, Nocentini S, Marchi E, Bottalico F, Fiorentini S, Travaglini D (2015). Impact of wildfire on the edaphic microarthropod community in a Pinus pinaster forest in central Italy. iForest 8: 874-883. - doi: 10.3832/ifor1404-008
Academic Editor
Massimo Faccoli
Paper history
Received: Jul 21, 2014
Accepted: Jan 27, 2015
First online: May 05, 2015
Publication Date: Dec 01, 2015
Publication Time: 3.27 months
Copyright Information
© SISEF - The Italian Society of Silviculture and Forest Ecology 2015
Open Access
This article is distributed under the terms of the Creative Commons Attribution-Non Commercial 4.0 International (https://creativecommons.org/licenses/by-nc/4.0/), which permits unrestricted use, distribution, and reproduction in any medium, provided you give appropriate credit to the original author(s) and the source, provide a link to the Creative Commons license, and indicate if changes were made.
Web Metrics
Breakdown by View Type
Article Usage
Total Article Views: 53567
(from publication date up to now)
Breakdown by View Type
HTML Page Views: 45036
Abstract Page Views: 2913
PDF Downloads: 4228
Citation/Reference Downloads: 22
XML Downloads: 1368
Web Metrics
Days since publication: 3717
Overall contacts: 53567
Avg. contacts per week: 100.88
Article Citations
Article citations are based on data periodically collected from the Clarivate Web of Science web site
(last update: Mar 2025)
Total number of cites (since 2015): 13
Average cites per year: 1.18
Publication Metrics
by Dimensions ©
Articles citing this article
List of the papers citing this article based on CrossRef Cited-by.
Related Contents
iForest Similar Articles
Research Articles
Role of serotiny on Pinus pinaster Aiton germination and its relation to mother plant age and fire severity
vol. 12, pp. 491-497 (online: 02 November 2019)
Research Articles
Post-fire effects and short-term regeneration dynamics following high-severity crown fires in a Mediterranean forest
vol. 5, pp. 93-100 (online: 30 May 2012)
Short Communications
Upscaling the estimation of surface-fire rate of spread in maritime pine (Pinus pinaster Ait.) forest
vol. 7, pp. 123-125 (online: 13 January 2014)
Research Articles
Analysis of forest fires causes and their motivations in northern Algeria: the Delphi method
vol. 6, pp. 247-254 (online: 13 June 2013)
Research Articles
Wildfire cause analysis: four case-studies in southern Italy
vol. 3, pp. 8-15 (online: 22 January 2010)
Research Articles
Influence of tree density on climate-growth relationships in a Pinus pinaster Ait. forest in the northern mountains of Sardinia (Italy)
vol. 8, pp. 456-463 (online: 19 October 2014)
Research Articles
Effects of defoliation by the pine processionary moth Thaumetopoea pityocampa on biomass growth of young stands of Pinus pinaster in northern Portugal
vol. 3, pp. 159-162 (online: 15 November 2010)
Research Articles
Post-fire recovery of the plant community in Pinus brutia forests: active vs. indirect restoration techniques after salvage logging
vol. 11, pp. 635-642 (online: 04 October 2018)
Research Articles
Large wildland fires and extreme temperatures in Sardinia (Italy)
vol. 7, pp. 162-169 (online: 14 February 2014)
Research Articles
Long-term implications of traditional forest regulation methods applied to Maritime pine (Pinus pinaster Ait.) forests in central Spain: a century of management plans
vol. 3, pp. 33-38 (online: 02 March 2010)
iForest Database Search
Search By Author
Search By Keyword
Google Scholar Search
Citing Articles
Search By Author
Search By Keywords
PubMed Search
Search By Author
Search By Keyword