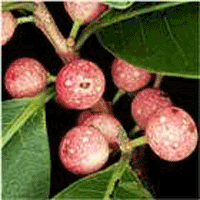
Elevational changes in a neotropical Fig (Ficus spp.) community in North Western Ecuador
iForest - Biogeosciences and Forestry, Volume 1, Issue 2, Pages 104-106 (2008)
doi: https://doi.org/10.3832/ifor0462-0010104
Published: May 20, 2008 - Copyright © 2008 SISEF
Short Communications
Abstract
Differences in primary production levels at high elevations sites have a direct impact on the numbers, and types, of fauna that areas of tropical forest can support. We investigated the effects of increasing elevation on a Neotropical fig (Ficus spp.) community in the Andean Choco region of Ecuador. Results show that there is a significant reduction in mean density of fig trees (p = 0.01), likewise mean basal area decreases with increasing elevation (p < 0.05). Causes are suggested and the possible consequences of this on frugivores is discussed.
Keywords
Choco, Cloud Forest, DBH, Frugivore, Los Cedros Biological Reserve
Introduction
It is well established that plant community structure and composition in tropical forests is highly influenced by changes in elevation ([34], [37]). This variation is mainly due to the changes in temperature, soil pH, solar radiation and rainfall levels associated with conditions at high elevations ([1], [40]) although local topography also plays a role ([28]). One effect of these changes is to create zones or “belts” of forest types which are distinct in the floral assemblages present ([22]). These belts are found to occur at different altitudes depending on latitude and local conditions ([28]). As would be expected there are also corresponding changes in faunal assemblages associated with this variation in forest type due to associated changes in primary production levels and the types of food resources produced ([23], [24], [8]).
The Choco bio-geographic region of north-western Ecuador contains some of the most diverse forests on earth. The region was listed by Myers et al. ([27]) as a “Biodiversity hotspot” and a priority for conservation due to this diversity as well as the high level of endemism and the high threat level faced by this region. The forests of the Ecuadorian Choco originally extended from just above sea-level, up the western slopes of the Andes, to over 3000m. Only an estimated 4% of the original forest cover still remains in Ecuador, most of this within protected areas ([27]), the largest of these being the 45.000 ha Parque Nacional Cotocachi-Cayapas and the contiguous 5.500 ha Los Cedros Biological Reserve (LCBR). The LCBR protects areas of primary and regenerating secondary forest that lie above 1000 meters above sea level. Although these are sizable tracts of intact forest they are of a different structure to those of the formerly forested lowland sites. Thus remaining forest types will have a direct effect on the numbers and types of animals and plants the area can and does support ([15]).
Within tropical forest communities it has been suggested that there are certain species which play significant roles in the maintenance of the community as a whole. Defined as “keystone resources” ([30], [29], [36], [31], [33]), these species must play a very important if not vital role in sustaining an ecosystem ([39], [31]). Such “keystone resources” may produce food at times when other resources are scarce, be acceptable and accessible to large numbers of consumers as well as being widely distributed and relatively common ([31]). Throughout the tropics and sub-tropics, including the Ecuadorian Choco, trees of the Genus Ficus sp.p. display intra- and inter-specific asynchronous fruiting regimes ([20], [26], [29], [2], [25], [39]) making fruits available to consumers throughout the year ([20], [26], [7], [30], [39], [36], [31]), they also feature prominently in the diets of many mammalian and avian species ([33]). Furthermore, they are found throughout the Neotropics, including the Choco-Darien bio-geographic zone, from lowland rainforest into the montane forest belt.
This study examines changes in density and distribution within the fig community of the LCBR in relation to increases in elevation. The main questions addressed are: (1) whether fig tree density decreased with increases in elevation; (2) whether DBH of fig trees also decreases with increased elevation; and (3) what effect this will have on fruit production. Both of which will affect the abundance and production of this “keystone resource” and therefore forest carrying capacity. This study forms part of the larger PRIMENET initiative (http://www.primenet.org.uk), which aims to document and protect the remaining areas of Ecuadorian Choco forest, and the critically endangered brown-headed spider monkey (Ateles fusciceps) which is endemic to the region ([38]).
Materials and methods
The Los Cedros Biological Reserve is located in Imbabura province, North Western Ecuador (0° 19’ N and 78° 47’ W). The reserve encompasses primary and secondary lower montane and montane tropical cloud forest ([19]). Situated on the western slopes of the Cordillera de los Andes, The reserve encompasses an altitudinal range from approximately 1000 m. up to 2710 m. at the peak of the Cordillera de la Plata range within an area of 60 km². There are two seasons, one long wet season from September to May and a shorter dry season from June to August, although rainfall still occurs on most days during this period. Average annual rainfall is 3150 mm with an average year round temperature of 20° C ([11]). Canopy height ranged from approximately 20-25 meters at lower altitudes to 20 meters at higher altitudes, closed canopy cover was found at all altitudes covered in this study, with crown projection of fig trees ranging from approx 25-100 m².
Between 28 May and 30 July 2006 a total of 19 km of transect walks were completed in nine different locations throughout the reserve; the transects consisted of 16 km of existing trails, at 1200-2000 m, and 3 km of newly cut transects, at 1200-1600 m, all trails were repeated to ensure correct sampling. Strip transect methodology was employed ([21]) with 5 m either side of the center line as the cut off point, thus giving a 10 m wide sampling strip. This strip width has been used in many previous studies of a similar nature ([18], [5], [9], [17]) and has been shown to give good coverage of area with limited manpower based on visibility either side of the transect. Smaller strip widths have been employed in other studies ([13], [12], [16], [3]) but it was felt that the larger strip width was better suited to this study. The total area sampled was 188.500 m², all fig trees of diameter at breast height (DBH) greater than 10 cm were counted and DBH measured.
DBH measurements were then used to estimate fruit production for the different altitudinal zones using the equation ([32], [35] - eqn. 1):
where Fr is the Fig fruit biomass production in grams, and DBH is the diameter at breast height in centimeters.
Breast height was taken to be 150 cm from the ground, although when trees with large buttressed roots were encountered DBH was measured immediately above the buttresses ([6]). Trees of both the sub-genus Urostigma, or strangling figs, and Pharmacosycea, or free-standing figs ([25]) were included. Similarly both monoecious and dioecious species were included. Although some differences in fruit production have been noted due to differing pollination strategies, these differences are not large and do not occur regularly ([20]). Sampled areas were stratified into four altitudinal zones, these were: 1200-1399 m, 1400-1599 m, 1600-1799 m and 1800-1999 m. Fig trees were identified ([14]) and tagged with high visibility “flagging tape” so as not to be re-counted: tape was removed after the study was completed to minimize impact on the area. Data were also collected on the location of trees on the existing trail system and new transects to test if there was a difference in observed densities, this was done to ensure there was no bias in the results due to sampling methodology ([18]).
Data were entered into Minitab V13 for analysis. Statistical analyses were carried out to examine trends and test for differences in density and fig tree DBH among the four altitudinal zones. DBH data were log transformed to ensure a normal distribution.
Results
A total of 165 trees were recorded with an overall density of 8.75 ha-1. Slightly higher densities were found on the newly cut transects, but this was not found to be significant (t = 1.008, p > 0.05). All trails and transects were therefore used in subsequent analysis. Overall mean fig tree DBH was 40.8 cm.
Mean densities of fig trees in the four elevational zones were 9.8 at 1200 - 1399 m, 8.3 at 1400 - 1599 m, 11.7 at 1600 - 1799 m and 1.9 at 1800 - 1999 m. A Kolmogorov-Smirnov test confirmed that density data were normally distributed (p > 0.05). Differences in densities were found to be significant (p = 0.01). Mean DBH for each of the altitudinal zones was 46.6 cm at 1200 -1399 m, 40.0 cm at 1400 -1599 m, 40.8 at 1600 - 1799 m and 32.7 at 1800 -1999 m.
A Kolmogorov-Smirnov test confirmed that log transformation of DBH data resulted in normally distributed dataset (p > 0.05). Regression of log DBH against altitude proved significant for the altitudinal factor (p < 0.05). By rearrangement of the regression equation the trend in mean basal area is represented by (eqn. 2):
Estimated fig tree fruit production for 1200 - 1799 m range was calculated together as differences in DBH between these altitudes, without inclusion of higher altitudes, was not significant. Estimated production for this range was Fr ≈ 55 Kg year-1 and 139 Kg ha-1 year-1. Estimated fruit production for 1800+m was calculated to be Fr ≈ 35 Kg year-1 and 96 Kg ha-1 year-1.
Discussion
The results confirm expected patterns of reduced densities of tropical plants at elevations with more temperate climes. This is also true for the reduction in mean DBH of fig trees. The results also suggest that 1800 m could mark the transition zone between the pre-montane and montane forest belts for this region ([22], [37]). The reduction in mean DBH of 52% between 1300m and 1800m probably reflects the impact of forest gap generation on the reduced growth rate at increasing altitude which is most probably due to the changes in temperature, solar radiation, humidity and soil pH associated with higher elevations ([24]).
Tree DBH is used as an estimator of fruit production as previous studies have shown fruit biomass production to be a power function of DBH in many tree species ([35]), and specifically tropical and sub-tropical figs ([32]). Similarly, the use of DBH has also proven more reliable then other methods at accurately estimating fruit production ([6], [36]).
The possible effect on frugivorous consumers who rely on figs when other food sources are scarce stem not only from reduced densities of fig trees, that reduces the number of food resources within a given territory, but also from a reduction in relative production levels of the individual trees between altitudinal zones as a result of smaller size in higher zones. The regression equation suggests a 52% reduction in DBH between 1300 m and 1800 m with a related reduction in relative fruit production ([32], [35]). It follows that these reductions could result in corresponding reductions in the presence and abundance of large bodied frugivores, such as primates. Indeed a reduction in numbers of primates at higher elevations has been reported in several previous studies ([4], [24]), including spider monkeys ([10]) and is commonly attributed to the increased energetic costs of finding sufficient food as a result of lower densities and reduced quality of resources ([41], [24]), and in the case of folivores on lower quality, less digestible food as a result of environmental conditions at high elevation sites ([24]).
In the case of conservation of the Choco bio-geographic region, and its endemic fauna, there are far reaching implications. As mentioned above much of the original forest cover at low elevations has been removed for human settlement. Although there remains some sizable areas of intact forest these are at higher elevations and may not be able to support viable numbers of large bodied frugivores. To properly protect all the species that inhabit the area it may be necessary to extend protection to cover a larger area of forest at lower elevations to help ensure the survival of species such as the brown headed spider monkey (A. fusciceps). This may be of prime concern as the very low numbers left in the wild, estimated at less than 250 mature individuals, may be at such levels as the forests they inhabit are unable to support a larger population leaving them, thus prone to extinction from other causes.
Acknowledgements
The authors wish to thank Jose deCoux, Ana Mariscal, Noga Shanee, Oxford Brookes University and the volunteers at the Los Cedros Biological Reserve. We are grateful to two anonymous reviewers for their comments on an earlier version of this manuscript. Also we wish to thank Martin Stanley of the Holly Hill Trust for his financial support.
References
Gscholar
Gscholar
Gscholar
Gscholar
Gscholar
Gscholar
Gscholar
Gscholar
Gscholar
Gscholar
Gscholar
Gscholar
Gscholar
Authors’ Info
Paper Info
Citation
Shanee S, Peck MR (2008). Elevational changes in a neotropical Fig (Ficus spp.) community in North Western Ecuador. iForest 1: 104-106. - doi: 10.3832/ifor0462-0010104
Paper history
Received: Aug 09, 2007
Accepted: Mar 05, 2008
First online: May 20, 2008
Publication Date: May 20, 2008
Publication Time: 2.53 months
Copyright Information
© SISEF - The Italian Society of Silviculture and Forest Ecology 2008
Open Access
This article is distributed under the terms of the Creative Commons Attribution-Non Commercial 4.0 International (https://creativecommons.org/licenses/by-nc/4.0/), which permits unrestricted use, distribution, and reproduction in any medium, provided you give appropriate credit to the original author(s) and the source, provide a link to the Creative Commons license, and indicate if changes were made.
Web Metrics
Breakdown by View Type
Article Usage
Total Article Views: 44488
(from publication date up to now)
Breakdown by View Type
HTML Page Views: 37926
Abstract Page Views: 2552
PDF Downloads: 3138
Citation/Reference Downloads: 176
XML Downloads: 696
Web Metrics
Days since publication: 6251
Overall contacts: 44488
Avg. contacts per week: 49.82
Article Citations
Article citations are based on data periodically collected from the Clarivate Web of Science web site
(last update: Mar 2025)
Total number of cites (since 2008): 8
Average cites per year: 0.44
Publication Metrics
by Dimensions ©
Articles citing this article
List of the papers citing this article based on CrossRef Cited-by.
Related Contents
iForest Similar Articles
Technical Reports
Conservation and use of elm genetic resources in France: results and perspectives
vol. 13, pp. 41-47 (online: 03 February 2020)
Research Articles
Networking sampling of Araucaria araucana (Mol.) K. Koch in Chile and the bordering zone of Argentina: implications for the genetic resources and the sustainable management
vol. 2, pp. 207-212 (online: 22 December 2009)
Editorials
Workshop COST E52 “Evaluation of beech genetic resources for sustainable forestry”
vol. 2, pp. 104 (online: 10 June 2009)
Research Articles
Delineation of seed collection zones based on environmental and genetic characteristics for Quercus suber L. in Sardinia, Italy
vol. 11, pp. 651-659 (online: 04 October 2018)
Commentaries & Perspectives
The genetic consequences of habitat fragmentation: the case of forests
vol. 2, pp. 75-76 (online: 10 June 2009)
Technical Reports
Population genetic structure of Platanus orientalis L. in Bulgaria
vol. 4, pp. 186-189 (online: 11 August 2011)
Research Articles
Patterns of genetic diversity in European beech (Fagus sylvatica L.) at the eastern margins of its distribution range
vol. 10, pp. 916-922 (online: 10 December 2017)
Research Articles
Comparison of genetic parameters between optimal and marginal populations of oriental sweet gum on adaptive traits
vol. 11, pp. 510-516 (online: 18 July 2018)
Research Articles
Seedling emergence capacity and morphological traits are under strong genetic control in the resin tree Pinus oocarpa
vol. 17, pp. 245-251 (online: 16 August 2024)
Review Papers
Genetic diversity and forest reproductive material - from seed source selection to planting
vol. 9, pp. 801-812 (online: 13 June 2016)
iForest Database Search
Search By Author
Search By Keyword
Google Scholar Search
Citing Articles
Search By Author
Search By Keywords
PubMed Search
Search By Author
Search By Keyword